1.2-I1
Husam Alshareef is a Professor of Materials Science and Engineering at King Abdullah University of Science and Technology (KAUST). He is also the Director of the newly-established Center of Excellence in Renewable Energy and Storage Technologies at KAUST. He obtained his Ph.D. at NC State University followed by a post-doctoral Fellowship at Sandia National Laboratories, USA.
He spent over 10 years in the semiconductor industry where he implemented processes in volume production for chip manufacturing. He joined KAUST in 2009, where he initiated an active research group focusing on the development of nanomaterials for energy and electronics applications. His work has been recognized by over 25 awards including the SEMATECH Corporate Excellence Award, two Dow Sustainability Awards, the Kuwait Prize for Sustainable and Clean Technologies, and the KAUST Distinguished Teaching Award. He has published over 600 papers and 80 issued patents. He is a Fellow of several prestigious societies including the American Physical Society (APS), Institute of Electrical and Electronics Engineers (IEEE), US National Academy of Inventors (NAI), Institute of Physics (IoP), Royal Society of Chemistry (RSC), and the Institute of Materials, Minerals and Mining. He has been a Clarivate Analytics Highly-cited Researcher in Materials Science for several years.
MXenes, a class of two-dimensional transition metal carbides and nitrides, have garnered significant attention in recent years as promising materials for energy-related applications. Their unique combination of properties, including exceptional electronic conductivity, impressive ionic conductivity, a large specific surface area, and highly tunable surface chemistries, make them highly versatile for a wide range of advanced energy technologies. These properties not only enhance energy storage and conversion efficiency but also allow for precise control over their performance characteristics by tailoring their preparation and material characteristics.
In this presentation, I will highlight the latest advancements from our group that leverage the exceptional properties of MXenes to develop innovative energy harvesting and storage devices. Our research explores a broad spectrum of applications, demonstrating the versatility of MXenes in addressing challenges across various domains of energy technology.
Specifically, I will discuss the integration of MXenes into commercial-scale silicon heterojunction solar cells, where we show they can replace expensive silver back contacts. I will also present our work on MXene use in salinity gradient energy harvesters, which exploit ionic conductivity and surface functionality for efficient energy extraction from saltwater gradients. Furthermore, our work in thermoelectric nanogenerators showcases how MXenes contribute to converting waste heat into electrical energy by optimizing thermal and electronic transport properties.
Additionally, MXenes have been demonstrator in triboelectric nanogenerators, where their mechanical robustness and electronic conductivity enable efficient energy harvesting from mechanical motion. Their role in ultrasound energy devices will also be highlighted, with a focus on their unique ability to convert acoustic energy into usable electrical energy.
Finally, I will present our advancements in integrating MXenes into microsupercapacitors, which combine high energy density and power density for next-generation compact energy storage solutions. These integrated devices demonstrate the ability to pair energy harvesting with storage, paving the way for self-powered electronic systems.
This comprehensive exploration underscores the transformative potential of MXenes in energy applications and highlights their role as key enablers of sustainable and efficient energy technologies. Through the examples presented, I aim to showcase the versatility and impact of MXenes in shaping the future of energy harvesting and storage devices.
1.2-I2
The field of battery research continually seeks to improve energy storage capabilities while addressing sustainability concerns. This applies in particular to the exploration and development of novel materials, such as the promising material group of MXenes. MXenes, known for their two-dimensional morphology, vast chemical composition range, and excellent electrical conductivity, require the synergistic integration of conversion or alloying materials to achieve high charge storage capacities.
The presentation highlights the development of high-performance sodium-ion batteries using MXene / antimony hybrid electrodes. By carefully optimizing synthesis parameters and material design strategies, researchers achieved an optimized electrode composition. This hybrid material exhibited a high reversible capacity of 450 mAh/g at 0.1 A/g, along with excellent cycling stability and rate capability. We also explore the combination of MXenes and SnO2, a conversion material, for enhanced lithium-ion battery performance of over 500 mAh/g for 700 cycles at 0.1 A/g. The researchers synthesized a nanocomposite consisting of a 50/50 mixture of SnO2 and MXene. The resulting nanocomposite demonstrated high-capacity retention over numerous cycles and excellent rate capability.
Additionally, we demonstrate MXene electrode recycling and upcycling. With binder- and additive-free MXene paper electrodes, we show the significance of finding sustainable and efficient approaches to recycle spent batteries. Researchers investigated the use of annealed delaminated MXene electrodes, obtained through vacuum-assisted filtration and annealing processes, in lithium-ion and sodium-ion batteries. The electrodes exhibited good electrochemical performance and were easily recovered through direct recycling processes, achieving high capacity recovery rates. Moreover, the cycled MXene electrodes could be transformed into TiO2/C hybrids with adjustable carbon content, providing opportunities for their utilization in various battery and electrocatalysis applications.
Collectively, we emphasize the potential of MXenes and MXene hybrid materials for enhancing charge storage capabilities in batteries. They also underline the significance of developing sustainable recycling and upcycling approaches for MXene electrodes, contributing to the overall advancement of battery technology.
1.2-O1
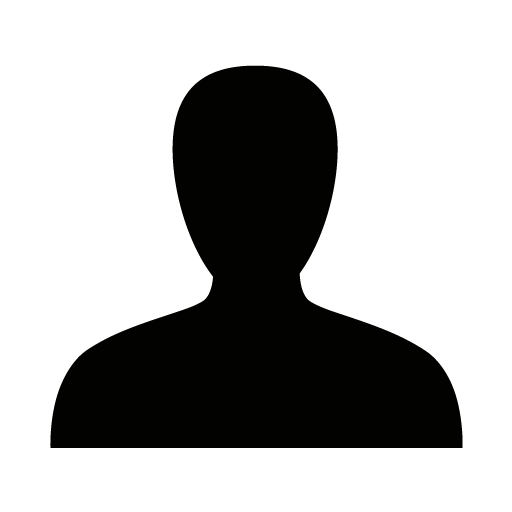
The oxygen evolution reaction (OER) is critical for water electrolysis, a key technology for the production of green hydrogen. While non-noble metal oxides show promise as OER catalysts, their application is limited by poor conductivity and stability.[1] This study demonstrates the functionalization of CuCo based hydroxides with V2CTx MXene to address these challenges, resulting in significantly improved OER performance in alkaline media.[2]
The CuCo@V2CTx composites were synthesized via a hydrothermal process, with varying MXene content (1%, 10%, 25%, 50%). The optimized CC50 catalyst achieved a lower overpotential and superior stability, outperforming the pure Co and CuCo hydroxides. Post-mortem analyses, including XPS and ICP-OES, revealed that the MXene not only enhanced hydrophilicity and charge transfer properties but also reduced Cu leaching during OER, improving the overall stability.
Characterization using SEM/TEM/EDX, XRD, and XPS confirmed that MXene mitigates aggregation and stabilizes CuCo on the electrode surface. Furthermore, the preferential leaching of V2CTx over Cu during stability tests preserved a higher concentration of active CuCo species, contributing to long-term catalytic performance. This work highlights the potential of MXene based hybrids as high-performance electrocatalysts for sustainable energy applications.
1.2-O2
iNSyT Technologies, a startup originated from the NanoInstitute at LMU Munich, is offering distruptive microscopy technologies for the demands of modern materials science. For centuries, microscopes have propelled technological progress. However, traditional microscopy, while effective for static structures, falls short in capturing the ultrafast, nanoscale processes essential for advancing renewable energy technologies—often at the risk of altering the sample itself. Our non-invasive, in-operando microscopy technology changes that. By capturing dynamic behaviors in real-time without disrupting the material or the process, iNSyT provides transformative insights into processes like catalytic water splitting, battery charging cycles, semiconductor corrosion, and light conversion in solar cells. With this level of precision, iNSyT enables scientists and industries to unlock the full potential of materials, accelerating breakthroughs and pushing the boundaries of what’s possible.
MXene, a novel class of two-dimensional materials, holds immense potential for revolutionizing energy storage systems due to its exceptional electrical conductivity, large surface area, and ability to accommodate various ions, thereby enhancing the performance of batteries and supercapacitors. [1-2]
In this pioneering study, state-of-the-art operando microscopy – interferometric scattering microscopy (iSCAT) – is employed to reveal the intricacies of ion diffusion within MXene nanostructures at the single-nanoparticle level (Fig. 1). iSCAT has already provided unique insights in various fields, from quantitative mass imaging of single macromolecules in biology [3-4] to elucidating ultrafast mechanisms in materials synthesis, such as the early-stage formation of covalent organic frameworks.[5] This research delivers an in-depth analysis of ion transport and charge transfer dynamics within 2D MXene structures, influenced by factors such as sheet size and thickness. The use of non-invasive operando microscopy for real-time tracking has provided critical insights into how these variables affect ion mobility within MXene frameworks. Notably, the study identifies how ion diffusion contributes to power density at the nanoparticle level, offering precise measurements of charge transport boundaries in MXene films.
These breakthroughs are crucial for advancing energy storage technologies, offering deeper insights into ultrafast nanoscale processes and the impact of nanoparticle heterogeneity on device performance at a larger scale.
1.3-I1
Prof. Zdenek Sofer is tenured professor at the University of Chemistry and Technology Prague since 2019. He received his PhD also at University of Chemistry and Technology Prague, Czech Republic, in 2008. During his PhD he spent one year in Forschungszentrum Julich (Peter Grünberg Institute, Germany) and also one postdoctoral stay at University Duisburg-Essen, Germany. Research interests of prof. Sofer concerning on 2D materials, its crystal growth, chemical modifications and derivatisation. His research covers various applications of 2D materials including energy storage and conversion, electronic, catalysis and sensing devices. He is an associated editor of FlatChem journal. He has published over 460 articles, which received over 15000 citations (h-index of 61).
The surface chemistry, structure, and morphology of MXenes play a critical role in their applications. Various methods of covalent functionalization have been reported, demonstrating significant improvements over pristine MXenes terminated with hydroxyl and fluorine functional groups. Furthermore, the direct conversion of MXenes into various chalcogenides, while retaining their original structure, can significantly enhance properties such as hydrogen and oxygen evolution overpotentials, as well as the capacitance of the material for electrochemical energy storage. Chemical functionalization, such as the introduction of charged zwitterionic compounds and defect engineering, has been shown to significantly improve the performance of MXenes in energy storage applications. Treating MXenes with different reagents, such as elemental chalcogens or pnictogens, effectively modifies their surface functionalization and can lead to the formation of composite materials. These composites, consisting of functionalized MXenes and transition metal dichalcogenides, exhibit significant enhancements in capacitance for supercapacitors and lithium-ion batteries. By leveraging specific chemical transformations, MXenes containing vanadium, niobium, and molybdenum can be converted into chalcogenides or vanadates/niobates. These processes pave the way for the development of advanced materials with promising applications in photocatalysis, supercapacitors, and batteries.
1.3-I2
Single-molecule detection represents the highest sensitivity in analytical techniques, enabling precise studies of individual molecular events. Methods based on single-molecule fluorescence energy transfer offer nanoscale distance measurements with significant implications for structural biology, biophysics, and material-biomolecule interfaces. Förster Resonance Energy Transfer (FRET) operates in the 3–10 nm range, typically involving two organic fluorophores as energy donor and acceptor. Graphene-based energy transfer (GET) extends this range to 10–40 nm, with a fluorophore as the energy donor and graphene acting as a broadband, unbleachable energy acceptor. FRET has been used predominantly to study protein conformational changes, but its measurements are often qualitative due to challenges in resolving nanometer-scale distances accurately. GET is a more straightforward quantitative method that enables precise z-localization of fluorophores. Notably, it has recently enabled real-time monitoring of DNA-protein interactions at the structural level [1]. However, graphene's hydrophobic nature and the strong influence of thickness on fluorescence lifetime measurements limit its biocompatibility to non-DNA systems and overall reproducibility.
Our research focuses on titanium carbide (Ti₃C₂Tₓ) MXene as a complementary, powerful energy transfer tool. MXenes possess unique advantages for studying biological assemblies that require hydrophilic surfaces, combining FRET-like short-distance sensitivity (1–10 nm) with GET's quantitative protocol, while offering unique material advantages such as hydrophilicity, biocompatibility, and thickness-independent energy transfer efficiencies.
This research line began by exploring MXene-DNA interactions with ensemble fluorescence spectroscopy measurements and molecular dynamics simulations. The interaction between MXene and DNA was found to be driven by salt bridges, coinciding with prior reports [2], with ssDNA and dsDNA lying horizontally on the surface while maintaining their native structure, approximately 1 and 1.3 nm away from the surface, respectively. In real-time hybridization experiments, we observed a fluorescence increase as ssDNA converted to dsDNA on the surface, suggesting that MXenes possess nanoscale sensitivity to sub-nanometer changes in the fluorophore position [3].
Next, we investigated MXenes’ fluorescence quenching mechanism and distance dependency of the energy transfer with single-molecule fluorescence microscopy and DNA origami nanopositioners using MXene thin films on glass. We positioned a single dye (ATTO 542) at defined distances from the surface (1–8 nm) via glycine-immobilized DNA origami nanostructures on MXene surfaces. Fluorescence quenching was observed between 1 to 8 nm, following a cubic distance dependence, consistent with the Förster mechanism observed in transparent conductors at the bulk level. These findings not only supported the hypothesis of MXenes' sub-nanometer sensitivity but also established them as hydrophilic, short-distance spectroscopic nanorulers uniquely positioned to operate in a distance regime inaccessible to GET. Additionally, the energy transfer efficiency was nearly independent of material thickness, enhancing robustness for sensing applications.
Finally, we demonstrated the utility of MXene's sensitivity, robustness and hydrophilic, salt-driven interactions for leaflet-resolved, single-molecule biosensing of fluorescently-labeled, 5-nm thick supported lipid bilayers (SLBs) as model cell membranes, directly fused on the MXene surface [4]. Similar to typical SLBs formed on mica surfaces—where a thin hydration layer separates the bilayer from the surface to maintain biomimetic fluidity—MXenes also supported biomimetic SLBs, offering an advantage over other metallic surfaces and graphene. At the single-molecule level, we determined the SLB thickness by independently resolving each leaflet of the bilayer and the hydration layer separating it from the MXene surface. This capability to discern such fine structures highlights MXene substrates as uniquely suited to studying lipid-protein interactions, membrane organization, and dynamic hydration phenomena, potentially offering new insights into lipid biology and biomembrane research.
1.3-I3
Hermenegildo García Gómez is a full Professor of the Instituto de Tecnología Química at the Univeristat Politècnica de Valencia. His group has expertise in CO2 utilization developing catalysts for CO2 conversion to methanol and C2+ products. He has published over 800 papers, has received more than 75.000 citations, has an H index of 133 and his name is included continuously since 2015 in the annual list of the most cited Scientists published by the Shanghai-Tomson Reuters. He is the recipient of the Janssen-Cilag award of the Spanish Royal Society of Chemistry (2011) and the Rey D. Jaime I award in New technologies (2016). He is doctor honoris causa by the University of Bucharest and Honorary Professor at the King Abdulaziz University since 2015. He was awarded by the Lee Hsun lecturership of the Chinese Academy of Science at Shenyang. He has participated in over 20 EU funded projects and is member of the panel of ERC Consolidator Grant as well as other Comissions and panels. He is President of the international advisory editorial board of ChemCatChem. Several of his publications have constituted research fronts in Chemistry (as defined by Essential Science Indicators) Database, such as Photocatalytic CO2 reduction by non TiO2 photocatalysis, catalysis by MOFs, etc.
MXenes having Metal-surface functional groups as well as structural defects offer much potential as solid catalysts, similarly as it happens with other metal carbides/nitrides and metal oxides. In addition, MXenes are also suitable supports for single atom and small metal clusters. The presentation will show that NH3-thermoprogrammed desorption and pyridine adsorption/desorption monitored by IR spectroscopy are suitable techniques to measure the acidity of these materials. It has been found that the actual density of Brönsted and Lewis acid sites and their strength depends on the MXene type, preparation procedure and post-synthetic treatments. MXenes have been found to catalyze a series of organic reactions, including hydrocarbon oxidation, electrophilic additions to C-C triple and double bonds and guanylation reactions among other. In many of these cases, the turnover frequencies achieved by MXenes are among the highest reported for these reactions using solid catalysts. This is the case of 1-butanamine addition to 1-hexyne for which turnover frequencies over 104 h-1 have been measured. This illustrates the potential that MXenes can offer in the field.
2.1-I1
Dra. Ana Primo Arnau
Research Group Leader, tenured Scientist. UPV, Valencia
Dr. Ana Primo earned her Ph.D. in Chemistry from the Universidad Politécnica de Valencia (Spain) in 2006. Following her doctoral studies, she undertook a postdoctoral stint at the Institute Charles Gerhardt in Montpellier, France, from 2007 to 2009. Currently, she holds a tenured position as a scientist at the “Instituto de Tecnología Química” (UPV-CSIC). Together with Professor Hermenegildo García, she founded the HG Energy Group, which she currently leads alongside Professor García.
Her research focuses on the synthesis of 2D materials such as graphene and boron nitride, exploring their applications in catalytic and photocatalytic processes. Notable among her investigations are CO2 reduction for methanol production and water splitting for hydrogen generation. With over 100 publications, Dr. Primo’s work has garnered more than 7,000 citations, reflecting her significant contributions to the field of chemistry, and she has an h-index of 44.
MXenes dots as photocatalysts
Ruben Ramirez Grau1, María Cabrero-Antonino1, Hermenegildo García1*, Ana Primo1*1Instituto de Tecnología Química, Consejo Superior de Investigaciones científicas-Universitat Politècnica de Valencia, Avda. de los Naranjos s/n, 46022, Valencia, Spain
Introduction
MXenes are 2D nanomaterials constituted by alternating one-atom thick layers of an early transition metal of the 3, 4, 5 or 6 groups of the Periodic Table (denoted as “M”) and a carbide, nitride or carbonitride layer (denoted as “X”) and have a general formula of Mn+1Xn.1 We recently reported that MXene dots (MDs) prepared by liquid-phase laser ablation of the corresponding MAX phase exhibit intrinsic photocatalytic hydrogen generation activity in the absence of any other photoresponsive component.2 Decrease of lateral size of MXenes to a few nanometers results in the operation of quantum confinement effects reflected in optical absorption and photoluminescence of MDs, these MDs exhibiting photocatalytic activity. Theoretical DFT calculations for Ti3C2 models indicates that the bandgap depends on the diameter of the MDs and on the nature of the surface functional groups. The compositional and structural versatility of MXenes will allow band engineering to align the energy values of the valence and conduction band to the values required to promote a given chemical reaction.
Continuing with this line of research, it is of interest to further explore the photocatalytic activity of these MDs. Specifically, it would be important to determine whether or not MDs promote the photocatalytic CO2 reduction, the efficiency of the process and the products formed. Herein it is reported that MDs are active in the photocatalytic CO2 reduction using H2 as electron and proton donor.
Materials and Methods
3 mg of MAX phase were suspended in 3 ml of MilliQ water and the suspension was submitted to ultrasounds in order to prepare a homogenous dispersion. Then, the system was irradiated for 3 h using the second harmonic of a Q switched Nd:YAG laser (Quantel Brilliant, 532 nm, 5 mJ/pulse, 5 ns fwhm) operating at 1 Hz. Finally, the resultant material was centrifuged for 4 h at 4000 rpm and, then, 1 h more at 13 000 rpm to isolate from the bulk MAX and other phases that sediment in the process the small MXene QD that remains in the supernatant.
Results and Discussion
Four MDs, namely Ti3C2, Ti2C, V2C and Nb2C were prepared from the corresponding Al MAX phases by 522 nm laser ablation in aqueous suspension, as previously reported.2 After preparation, the four MD samples were characterized by different techniques, including AFM and transmission optical microscopy. Table 1 lists the corresponding average thickness and average particle lateral size values for each sample. The activity of the four MD samples was evaluated for the photocatalytic CO2 hydrogenation under UV-Vis irradiation at 200 oC at 1 bar pressure, using a H2/CO2 ratio of 1/3. For the four samples, evolution of CO and methane as the only products was observed in different proportions. Fig. 1 shows the temporal product evolution upon irradiation of each MD, while Table 1 indicates the CO and methane production rates determined from these plots.
Significance
Photocatalytic CO2 reduction is a clean technology that converts CO2 into high-value-added products. The interest of the present study is to show that, MXenes by themselves, can exhibit an intrinsic photocatalytic activity that can be modulated depending on the composition. Since MXenes allow a wide range of compositions, including bimetallic solid solutions and a wide range of surface functional groups, there is still much room for improvement in the intrinsic photocatalytic efficiency by adjusting these parameters and also to achieve photocatalytic stability.
2.1-I2
The pseudocapacitive behavior of Ti3C2Tx MXenes promises high power and energy densities thanks to redox reactions occurring during the (de-)protonation of the MXene surface in acidic electrolyte. Nevertheless, local electrochemical processes occurring at the MXene-electrolyte interface and the associated changes of the MXene surface chemistry are currently largely unexplored. To this aim, soft X-ray spectroscopies are particularly relevant as they enable the selective characterization of either the electrolyte or the material of interest thanks to their element specificity.1 Furthermore, the high spatial resolution (<30 nm) offered by X-ray Microscopy can provide precious information about local inhomogeneities at the nanoscale,2,3 enabling the characterization of single MXene flakes.
In this talk, I will introduce X-ray spectromicroscopy for monitoring the surface chemistry of Ti3C2Tx MXenes as well as intercalated species at the single flake level. In situ cells for the characterization of MXenes at controlled temperature or in liquid will be introduced. The effect of proton and alkali cations intercalation on the electronic structure of Ti and O atoms in the MXene will be discussed, as well as the electronic signature of water confined in the MXene interlayer. Perspectives towards correlative optical spectromicroscopy techniques will also be discussed.
2.2-O1
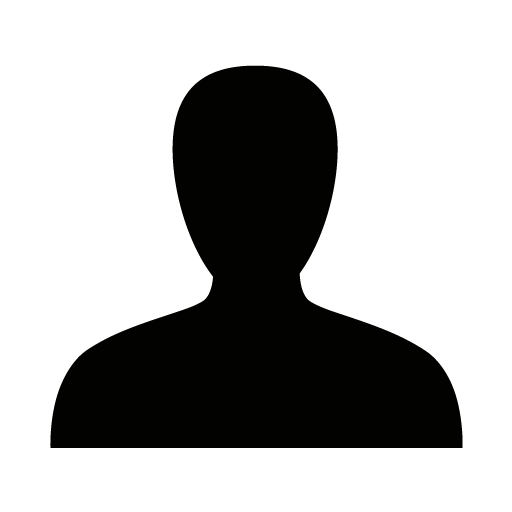
MXenes are highly conductive materials with a high surface area, offering potential as promising catalyst supports in proton exchange membrane fuel cells (PEMFCs), a technology recognized for sustainable energy conversion. This study centers on a comparative analysis of various platinum (Pt) loadings uniformly distributed across MXene sheets to enhance catalytic performance. The synthesized materials were comprehensively characterized using X-ray diffraction (XRD), X-Ray Photoelectron Spectrscopy, scanning electron microscopy (SEM) and Raman spectroscopy to investigate their structural attributes and morphology and functional groups, providing insights into their stability and interaction with Pt particles. Electrochemical tests were conducted in rotating disc electrode (RDE) and half-cell setup to assess the efficiency of the catalysts in oxygen reduction reaction (ORR), a crucial step in PEMFC performance due to its role in limiting cell output. Results reveal that Pt particles on MXenes not only function effectively as ORR electrocatalysts but also improve reaction kinetics, positioning MXene-supported Pt catalysts as a promising alternative for PEMFC applications.
2.2-I1
Christina Birkel is an Associate Professor in the School of Molecular Sciences at Arizona State University (ASU) and holds a joint professorship position in the Department of Chemistry and Biochemistry at the Technische Universität (TU) Darmstadt. In 2024, she was appointed Navrotsky Professor of Materials Research at ASU. Prior to her independent career, she was a Junior Research group leader at TU Darmstadt (Habilitation in 2018), a Postdoctoral Researcher (group of Prof. Galen Stucky) at the University of California, Santa Barbara (Feodor Lynen Research Stipend, Alexander-von-Humboldt Foundation) and completed her PhD thesis (group of Prof. Wolfgang Tremel) at the Johannes Gutenberg-University of Mainz holding a stipend of the Graduate School of Excellence (2010). Her group focuses on the synthesis and structural science of new types of layered solids and two-dimensional materials with a strong focus on carbides and (carbo)nitrides (MAX phases and MXenes), as well as the investigation of their properties including transport (electronic, magnetic), high-temperature and electrochemical behavior.
MXenes – transition metal-based carbides and (carbo)nitrides with the general chemical composition Mn+1XnTx (M = early-to-mid transition metals, X = C and/or N, Tx = surface groups, n = 1-4) are an incredibly fascinating class of 2D materials that have left almost no potential application area untouched. As much research as is dedicated to exploring their unique properties, for example in the context of biomedicine, catalysis and energy storage, especially of Ti-MXenes, as little focus is dedicated to non-Ti-MXenes as well as their synthesis science.
In this talk, I will discuss the synthesis of different V-MXenes derived from their MAX phase siblings. The preparation of the precursor MAX phases is not trivial as shown for MAX phase examples V4AlC3 and (V/Mo)5AlC4. MAX phases with minimal amounts of side phases as well as knowledge of their chemical composition are key to obtaining high-quality MXenes that allow for meaningful discussions of their properties. These aspects will be covered during my discussion of the “higher n” MXenes V4C3Tx and (V/Mo)5C4Tx including discussion of their electrocatalytic performance in the hydrogen evolution reaction (HER). Besides, I will highlight key synthetic chemistries during the exfoliation of V2AlC as well as its Mo-substituted analogs and show how the catalytic properties depend on the V/Mo ratio.
We take advantage of diverse synthesis and exfoliation tools ranging from conventional to microwave heating and aqueous acid as well as Lewis acid (molten salt) etching. All materials are carefully characterized by diffraction (synchrotron and lab X-ray), microscopy and spectroscopy (lab and hard-X-ray photoelectron spectroscopy) techniques.
2.2-O2
Producing hydrogen (H2) through electrocatalytic water splitting in an electrolyzer offers a clean and sustainable pathway to generate an eco-friendly fuel and energy carrier. The oxygen evolution reaction (OER) plays a critical role in this process, though its efficiency is bottlenecked by sluggish kinetics [1-3]. Noble-metal-based catalysts, particularly IrO₂ and RuO₂, are the state-of-the-art OER electrocatalysts available today due to their excellent activity but are hindered by high costs and instability issues. In particular, a major obstacle to the large-scale application of Ru lies in its tendency to leach in alkaline media [4, 5].
To address these limitations, extensive research has focused on improving the stability of noble-metal-based catalysts, reducing noble metal usage while retaining high performance, and exploring non-noble metal alternatives with strong OER activity. Transition-metal-based catalysts are promising, affordable alternatives as they are stable in alkaline conditions and have low OER potentials [6, 7]. However, their limited conductivity restricts their catalytic performance in OER applications. To overcome both the instability issues and the OER activity, we propose using MXenes, a rapidly advancing family of 2D transition metal carbides and nitrides, as conductive supports to form NiRu@Ti3C2Tx hybrids [8-12]. While many studies have explored MXene-based materials for applications in water splitting, OER remains relatively less-explored compared to the hydrogen evolution reaction (HER). Moreover, there is limited information on how MXenes influence the stability of metal/oxide during alkaline OER.
In this study, the potential of MXenes to enhance the stability of Ru-based catalysts, which are known for their substantial dissolution in alkaline media during OER, was investigated. Here, a bimetallic NiRu compound was synthesized and incorporated onto the Ti3C2Tx MXene surface at varying concentrations (1%-25%) using a facile hydrothermal method. The resulting NiRu@Ti3C2Tx composites were tested for OER activity in 1 M KOH to examine the impact of Ti3C2Tx content on Ru dissolution and overall catalytic performance. After incorporating Ru into a stable Ni-based environment and functionalizing Ti3C2 with NiRu, the composites showed reduced Ru leaching and increased OER activity compared to pure Ru. Higher Ti3C2Tx content further stabilized Ru. These results highlight the positive impact of Ti3C2Tx functionalization on the stability and efficiency of Ru-based OER catalysts.
2.2-O3
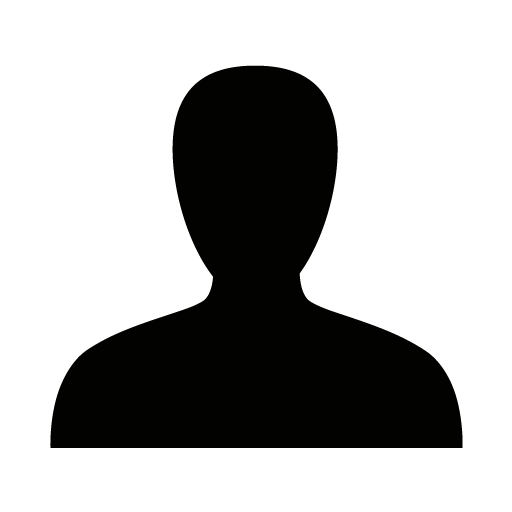
Two-dimensional (2D) materials have attracted significant attention for their high surface area, mechanical flexibility, and tunable electronic properties, enabling applications in energy storage, flexible electronics, optoelectronics, and biomedicine. The discovery of graphene's exceptional properties spurred interest in exfoliating other 2D materials from bulk crystals, uncovering diverse chemical structures and functionalities. Electrochemical exfoliation (ECE) offers a promising approach for mass-producing graphene and other 2D materials due to its mild conditions, fast processing, simplicity, and high yield. While ECE of layered van der Waals (L-vdW) crystals has advanced, research on layered non-van der Waals (L-NvdW) materials is still in its infancy. This work investigates ECE for producing 2D nanoplatelets from L-NvdW crystals, comparing exfoliation techniques for L-vdW and L-NvdW materials and reviewing recent progress in ECE methods for L-NvdW crystals. Key topics include selective extraction of 'M' and 'A' layers from MAX phases, decalcification of Zintl phases, and oxide delocalization from metal oxides. We also present our latest research on the electrochemical exfoliation of layered TM2SC-type MAX phases.
2.2-O4
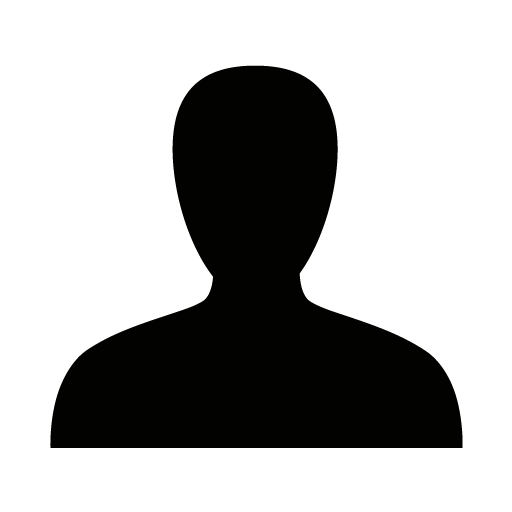
The growing global demand for sustainable energy has heightened the need to develop efficient electrocatalysts for energy conversion processes, particularly the oxygen evolution reaction (OER), which is a key part of water splitting for green hydrogen production. Although a wide range of catalysts has been investigated for use in electrolyser devices, challenges such as cost, conductivity, and stability continue to drive ongoing research in this area.[1]
Transition metal oxide (TMO) materials, despite their great potential as OER catalysts, suffer from limited conductivity, which hampers effective charge transfer during electrochemical processes, and can lead to deactivation due to structural transformations during the OER.MXenes, with their exceptional surface area and conductivity, are promising supports for enhancing the electrocatalytic performance of TMO-based OER catalysts.[2] However, MXenes are susceptible to oxidation under applied potentials, which can alter their structure and properties. By synergistic combination of TMOs with MXenes, a highly efficient OER catalyst could be developed, combining the enhanced conductivity, hydrophilicity, and increased surface area of MXenes with the active OER sites of TMOs.[3] While there have been numerous successful attempts in producing highly performing TMO/MXene composites, the overwhelming number of such reported catalysts is based on the first found and best known Ti3C2Tx MXene.[4] This leaves a wide range of potentially highly performing OER catalysts based on MXenes other than Ti3C2Tx yet to explore.
In our recent investigations we found that coprecipitation of CoFe layered double hydroxides (LDH) with V2CTx MXene at various weight percentages resulted in CoFe/ V2CTx composites that outperformed the separate components and physically mixed components as OER catalysts. Furthermore, we could show that the presence of V2CTx during the synthesis promotes the formation of a highly active CoFe-LDH phase as opposed to the pure CoFe sample. We further investigated the materials’ oxidation states under OER conditions via synchrotron based in-situ XAS to detect the catalytically active species for the OER.
These findings demonstrate the great potential of TMO/ V2CTx composites as OER electrocatalysts and are a step towards understanding the reasons for their improved catalytic activity.
2.2-O5
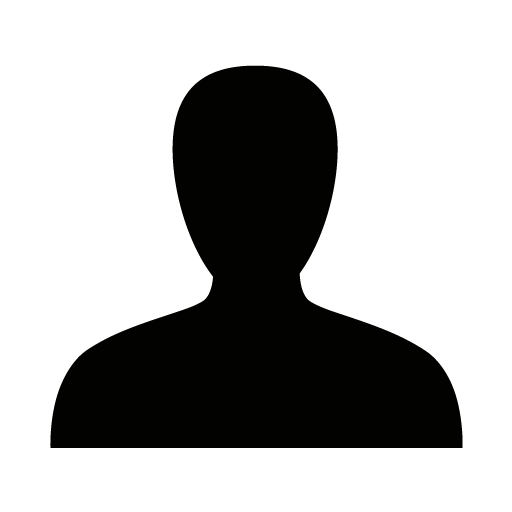
As the world increasingly turns towards sustainable energy solutions, the efficient storage and transport of hydrogen has become critical. Ti3C2 MXenes, with their unique 2D structure and exceptional properties, are at the forefront of this technological revolution. For Ti3C2 MXene as energy storage material using hydrogen it is crucial to understand hydrogen bonding and diffusion, since unexpected properties may arise in terms of interaction with lattice atoms.
In this paper, it is shown that the chemical bonding of hydrogen atoms and molecules extends far beyond the simple picture of covalent, ionic and multicenter bonds. Density functional theory was used for the calculations. The Ti3C2 layers and surfaces were modelled in a slab geometry. The properties of H and H2 were further analyzed by calculating the vibrational eigenmodes and their intensities by employing density-functional perturbation theory.
The results clearly show that in Ti3C2 hydrogen atoms and H2 molecules form multicenter bonds. On the surface and between two Ti3C2 sheets, this bonding is restricted to the formation of Ti−H bonds. However, at interstitial sites, both H and H2 form multicenter bonds. This includes the nearest neighbor Ti atoms and also C atoms. Notably, C−H bonds involve the formation of s−p hybrid orbitals. For H2 molecules the formation of multicenter bonds results in an increase in bond length to 2.07 Å on the surface and to 1.85 Å at the interstitial site. When H2 is accommodated between two Ti3C2 sheets the molecule dissociates. The vibrational eigenmodes for all complexes have been calculated and vibrational frequencies ranging from 890 to 1610 cm−1 were obtained. This indicates that the hydrogen multicenter bonds are stable.
For efficient hydrogen storage and release the understanding of H transport in MXenes is vital for optimizing their performance in applications. Hence, the diffusion properties of H in Ti3C2 MXene were determined from ab-initio calculations. For this purpose, migration barriers, hopping frequencies, vacancy formation enthalpies, and the vibrational entropy were calculated to determine the temperature dependent diffusion coefficients. The data reveal that hydrogen diffusion predominantly occurs via interstitial sites, while vacancy-mediated diffusion plays a negligible role.
2.3-I1
The future of electronics, wearables, healthcare, and smart environments is being shaped by the integration of IoT (Internet of Things) with flexible technologies. A plethora of printed electronic components, including OLED displays, OPVs, TFTs, antennas, and diodes, among others, have already been developed [1], [2], [3]. These can then be integrated into technological platforms with a multitude of applications. When building such platforms, it is essential to address the question of energy autonomy. For flexible electronics, energy is normally harvested from the environment (solar energy, heat, movement, etc.) and stored in supercapacitors (SC) [4] 4. These energy storage devices are the optimal choice for printed and wearable electronics. They offer a long shelf life, seamless integration, and rapid charge and discharge capabilities. From many available options, MXenes have been regarded a good choice for SC manufacturing thanks to their optimal chemical properties. MXenes have a hydrophilic surface due to the presence of hydroxyl, oxygen, or fluorine functional groups (-OH, =O, -F), high electrical conductivity up to ~10,000 S/cm, especially for Ti₃C₂Tx, high flexibility, high capacitance (~1500 F/cm³ for Ti₃C₂Tx), fast ion transport, chemical stability, and more. As such, for the last couple of years, there has been a tremendous focus on MXene synthesis and implementation as SC electrodes [5], [6], [7].
This presentation will prove the vital importance of MXenes in the fabrication of SC electrodes for printed and flexible electronics. It will also show how they compare to other promising technologies and highlight their advantages and shortcomings. [8], [9]. It will also be discussed some strategies for MXene-based SC development to meet different applications requirements, such as voltage window, usable area and stability. However, the full potential of MXenes will only be exploited once the significant hurdles that currently stand in the way have been overcome, namely long-term stability and green synthesis. Nevertheless, due to their rich chemistry and optimal properties for electrochemical energy storage, MXenes are poised to play a significant role on powering up future and printed and flexible electronics.
2.3-O1
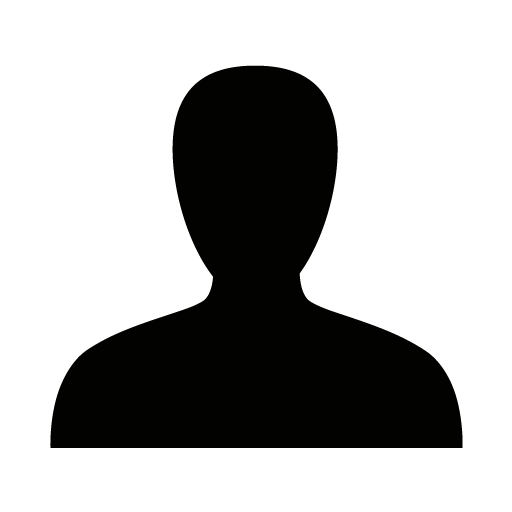
Electrolytic water splitting is a clean and sustainable way of producing hydrogen gas, which has huge potential as a fuel source. There is a vast range of materials that can replace expensive state-of-the-art catalysts, and Transition metal oxides (TMOs)-based catalysts are currently under consideration due to their cost-efficient nature and abundance. However, their poor conductivity presents a major challenge. To address this issue, MXenes have emerged as a promising option to be integrated with TMOs thanks to numerous beneficial properties, such as high conductivity, hydrophilicity, and tunneling capacity, which makes them an excellent choice as a mechanically stable and conductive material [1]. To enhance the electrochemical activity and stability of Ti3C2Tx MXenes, the control of surface functionalization and area is paramount. In this regard, this study utilizes HF in situ generation to remove Al-elements for the direct production of delaminated Ti3C2Tx, and, consequently, exposing their high surface area. In this study, Ni-based MXene composites were fabricated with varying MXene content to investigate the effect of the MXene on the OER. The findings of this study provide a comprehensive understanding of the Ti3C2Tx MXenes influence on OER activity in terms of Ni dissolution, and electro-mechanic-chemical-stability, thus providing valuable insights for their implementation and the importance of MXenes content optimization in hybrid electrocatalysts for OER.
2.3-O2
As a carbon-free energy source and courier, ammonia (NH3) plays an irreplaceable role in agriculture, medicine, and future renewable energy technologies [1,2]. Traditionally, industrial production of NH3 relies on the energy-intensive and large CO2 emission Haber-Bosch process, whereby nitrogen (N2) and hydrogen (H2) are the precursor materials [3]. As a result, a wide-spread of alternative sources and mechanisms, other than the use of N2 for the synthesis of NH3, have recently been explored. Amongst the various routes, electrochemical nitrate reduction reaction (NO3-RR) has received extensive attention due to its efficient synthesis of NH3 under mild conditions. While taking into consideration NO3-RR as a conducive and energy efficient route for synthesizing NH3, identification of non-precious metals and high-efficiency electrocatalysts remains the major challenge towards commercial practicality of NO3-RR and its sister mechanisms. As an emerging member of the two-dimensional materials family, MXenes have received increasing attention for electrochemical energy conversion because of their superior metallic conductivity, large specific surface area, hydrophilicity, and controllable surface functional groups [4,5], thereby possessing great application potential for NO3-RR. Herein, we report the potential application of molybdenum-based Mxene (Mo2TiC2Tx) materials in the field of NO3-RR. At low applied potential of -0.8 V (vs. Ag/AgCl) and in 0.1 M KOH electrolyte containing KNO3, Mo2TiC2Tx exhibited excellent NO3 reduction performance with high Faradaic Efficiency (FE) of ~18.3%, good selectivity for NO3-reduction to N2 (~87.9%), good hinderance to parasitic hydrogen evolution reaction (HER), along with displaying outstanding NH3 yield rate of 176.1 µg.h-1.mgcat-1. The high activity of the Mo2TiC2Tx MXenes was attributed to the abundant surface defects at low coordinated Mo and/or Ti sites, as well as the electrical conductivity and large surface area of the MXenes [4,5]. In general, this study provides a practical pathway into tailoring nanoengineered materials for their potential application in wastewater treatment and environmental remediation.
2.3-O3
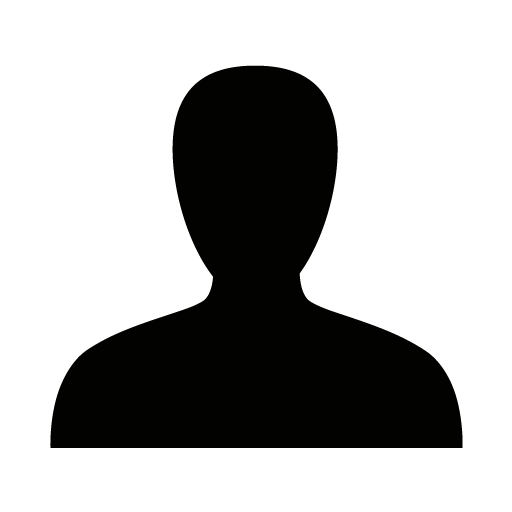
In recent years, Single-Junction Perovskite Solar Cells (SJ-PSCs) have emerged as a competitive alternative to silicon based solar cells in terms of efficiency. However, their stability under humidity and high-temperature conditions remains a major challenge [1]. In this context, the incorporation of 2D nanomaterials, such as MXenes, into SJ-PSCs offers a promising route to enhance both stability and efficiency [2]. Among these materials, Ti3C2Tx MXene stands out due to its excellent electrical conductivity and tunable electronic structure. The latter, along with its work function, can be controlled via surface terminal groups, which depend on synthesis methods and post-treatments [3]. In order to integrate Ti3C2Tx MXene into SJ-PSCs, morphological, chemical, and structural characterization, as well as a dispersibility study in typical organic solvents used during photovoltaic device fabrication, are required.
In this study, Ti3C2Tx MXene (Tx are -F, -O, and -OH terminal groups) was synthesized via wet chemistry under mild conditions from Ti3AlC2 (MAX phase). The resulting highly concentrated aqueous dispersion was used to prepare freestanding films via vacuum assisted filtration. X-ray Diffraction (XRD) analysis of the synthesized material revealed the successful etching of the MAX phase, since a typical pattern with a series of evenly spaced peaks corresponding to the characteristic (00X) (X = 2, 4, 6,…) family of planes of Ti3C2Tx was observed. Control of Ti3C2TX flake thickness was achieved by optimizing centrifugation procedure. As a result, a suspension of single-layer or few-layered structure was obtained (where each layer shows a thickness of 1-1.5 nm and lateral dimensions in the micrometer range (1-10 μm)) as revealed using Atomic Force Microscopy. When studying dispersibility of Ti3C2Tx MXene in solvents involved to SJ-PSC fabrication, it was found that polar solvents promote dispersion, in contrast to non-polar ones, which correlates with the presence of -O and -OH as MXene-terminal groups. Additionally, aprotic solvents showed better dispersibility than protic solvents. UV-Vis Spectrophotometry characterization showed the characteristic 750-800 nm absorption band, attributed to Ti3C2Tx MXene localized surface plasmon resonance (LSPR). The LSPR absorbance as a function of concentration was analyzed in selected organic solvents, enabling the determination of absorption coefficients.
The obtained results lay the basis for obtaining Ti3C2Tx MXene dispersions with controlled concentrations, facilitating their further integration into SJ-PSCs. In addition, the challenges of creating Ti3C2Tx nanocomposites with metal nanoparticles for modulating the work function will be discussed.
1.1-I1
Hamideh Darjazi (Ph.D., 2021) is an Assistant Professor in the Department of Applied Science and Technology at Politecnico di Torino. Her multidisciplinary research encompasses the synthesis of active materials and their chemical, morphological, and structural characterization, with a particular focus on crystallographic properties. She conducts electrochemical analyses to establish correlations between the characteristics of materials and electrodes with their performance in lithium-ion (LIBs) and sodium-ion batteries (NIBs).
Her research also centers on the development and electrochemical evaluation of advanced polymer-based electrolytes—including gels, solids, hybrids, and composites—as sustainable alternatives to conventional liquid carbonate electrolytes.
Renewable energy production is inherently intermittent, necessitating large-scale implementation to enhance energy storage capacity, which presently accounts for less than 1% of global electrical energy production. The pursuit of greener, more affordable, and safer rechargeable batteries is currently recognized as strategically important in advancing electrochemical energy storage technology, addressing environmental concerns and establishing a sustainable energy economy. Li-ion batteries (LIBs) and Na-ion batteries (NIBs) play a crucial role as energy storage devices for electric vehicles and smart grids. It is widely recognized that a detailed analysis of an electrode reveals that each component (active material, conductive carbon, current collector, and binder) contributes to the overall battery performance. It has been demonstrated that the binder, despite its relatively low content, typically a few percent of the total composition, plays a decisive role in determining the electrode performance, which is noteworthy considering its chemical and electrochemical inactivity. In addition, the transformation from liquid- to gel-/quasi-solid or fully solid-state architectures is expected to improve safety by using low-volatile, non-flammable materials and energy density of energy storage devices by enabling the use of lithium metal anodes, particularly if constraints of low ionic conductivity, low cation transport properties and stringent processing conditions are overcome [1].
In this context, here an overview is offered of the recent developments in our laboratories on the development of innovative solutions including recycled or biosourced polymer binders/electrolytes towards the development of sustainable, safe, high-performing post-lithium batteries. These include the possibility of repurposing in batteries the recycled polyvinyl butyral (PVB) from post-consume laminated glasses (from automotive and construction) that cannot be reused in glasses because of degraded optical properties. Three strategies were pursued: using recycled PVB as binder in the electrode composition, transforming it into a membrane to be used as electrolyte separator [2], and utilizing it in advanced solid-state batteries. In the case of PVB as binder, we investigated the electrochemical and structural properties of polymer blends of PVB with standard binders, as polyacrylic acid (PAA) and poly(vinylidene fluoride) (PVDF), demonstrating its effective use in the development of various electrodes, including hard carbon (HC) anodes, and high-energy cathodes (NMC and NVP) showing full capacities even at high C-rates and stable long-term operation at ambient temperature, which pave the way to the development of more sustainable binders/separators from waste products for next-generation, sustainable energy storage. Regarding solid-state batteries, the incorporation of recycled PVB into polyethylene oxide (PEO)-based solid polymer electrolytes was investigated to address both performance and sustainability challenges. This approach enables the design of composite polymer electrolytes with improved mechanical strength, thermal stability, and electrochemical performance while promoting the use of recycled materials to reduce environmental impact.
Overall, in all cases, preliminary results are highly encouraging and pave the way to the development of more sustainable separators and binders from waste products for safe, low-cost energy storage devices.
1.1-I2
Rechargeable aqueous Zinc (Zn) batteries are one of the emerging beyond lithium-ion batteries that could fulfil the requirements of cost-effective, safe, and reliable large-scale storage systems.[1,2] Zn metal is abundant, globally available, and non-toxic, with a promising theoretical capacity of 820 mA h/g.[3] However, aqueous Zn batteries encounter several degradations upon cycling due to the dendrite formation, poor Coulombic efficiency and hydrogen evolution reaction.[4] Additionally, separator technology remains a major bottleneck, with no efficient commercial options. Commonly used glass-fiber and cellulose filters are not optimized for Zn anodes, leading to poor compatibility. Thus, the development of advanced electrolytes and separators is crucial for enhancing aqueous Zn battery performance.
In this study, we have successfully engineered both a high-performing aqueous eutectic electrolyte and a novel chitin-based separator. Our electrolyte formulation is based on the combination of a strongly chaotropic cation, Gua, and a strong kosmotropic anion, Ac, to precisely tailor their strong and weak coordination with water, respectively. This strategy results in a weakly solvated electrolyte with improved ion transport properties alongside stabilisation of the Zn metal anode. On the other hand, the nanochitin-based separator, achieved through surface modification of chitin fibre with amine and carboxylic groups, showcases outstanding mechanical properties and compatibility with Zn anodes. Notably, this separator/electrolyte significantly enhances Zn cycling stability, demonstrating a remarkable increase from a mere 200 cycles with conventional glass-fibre and cellulose separators to 1000 cycles. This combined approach presents a compelling strategy for the advancement of high-voltage aqueous Zn metal batteries, marking a significant step forward in battery technology.
1.1-O1
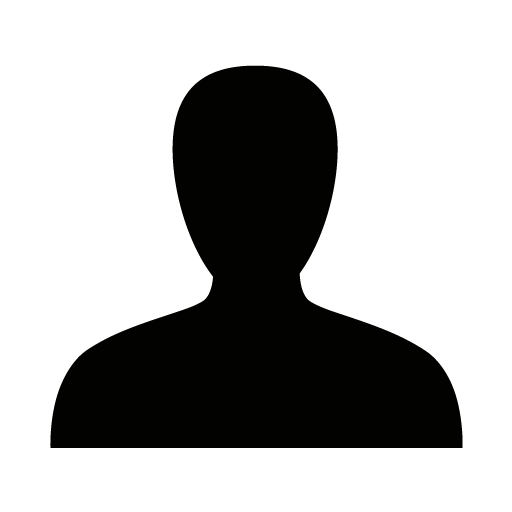
The rapid progress in energy storage technologies demands innovative solutions to challenges related to sustainability, cost, and performance. Aqueous zinc-based energy storage systems, such as zinc metal batteries (AZMBs) and zinc-hybrid capacitors (ZHCs), offer promising possibilities due to their inherent safety, cost-effectiveness, and scalability.[1] Nonetheless, complications like hydrogen evolution reactions, dendritic growth, and water-induced parasitic reactions are major obstacles to be overcome before these technologies can be practically employed on a large scale. The following abstract summarizes findings from three new central studies that place MCEs at the forefront in driving a new generation of research breakthroughs to overcome these challenges and improve performance in aqueous zinc systems.
The first study investigates an advanced MCE containing a combination of Zn(TFSI)₂, LiTFSI, and polyethylene glycol (PEG400) to improve the performance of Zn//LFP hybrid batteries.[2] By confining water molecules and reducing free water activity, the optimized MCE enhances the reversibility of Zn plating/stripping and significantly improves long-term cyclability. The Zn//LFP battery, in turn, showed quite high capacities of 120 mAh g⁻¹ with excellent stability-65% capacity retention after 200 cycles-whereas the self-discharge rate is reduced and parasitic reactions are suppressed compared to conventional electrolytes. These confirm the effectiveness of MCEs for solutions of major anode and cathode problems in AZMBs.
The second investigation is concerned with a Zn//V₂O₅ battery, which works out a newly developed MCE, using Zn(TFSI)₂ as the main salt.[3] The electrolyte formulation in this work enhances ionic conductivity, hence boosting ion transport properties to further ensure superior rate capability and decent cycle stability. It obtains excellent performance metrics of the Zn//V₂O₅ system, such as 78% capacity retention after 1000 cycles and an impressive reduction in self-discharge. Mitigation of free water activity hence improved the stability window, which assured reversible Zn plating/stripping and placed the MCE as a versatile strategy toward the advancement of AZMBs for practical applications.
Another study investigates the use of PEGDME-based MCE in ZHCs as means to solve such Zn anode problems but without sacrificing its practicability.[4] The advanced MCE obtained had a widened electrochemical stability window of up to 2.7 V, which reduced the water activity and hence improved anti-corrosion properties that enabled superior performance in Zn//Cu and Zn//Zn symmetric cells. The hybrid system of Zn/MCE/AC achieved energy density at 138 Wh/kg, perfect capacitance of 281 F/g, and superlative cyclability, with capacity retained up to 100% after 19,100 cycles. These results really underline the big capability of MCEs in providing high-power, long-life energy storage solutions.
Collectively, these works highlight how MCEs will be game-changing in overcoming fundamental challenges in aqueous-based zinc energy storage systems. By taking advantage of novel electrolyte chemistries to suppress parasitic reactions, enhance ionic transport, and stabilize electrode interfaces, MCEs will provide a route toward safe, efficient, and sustainable energy storage systems. This work presents an important milestone toward successful practice application of AZMBs and ZHCs and in particular provides for impactful solutions to global challenges in energy storage.
1.1-O2
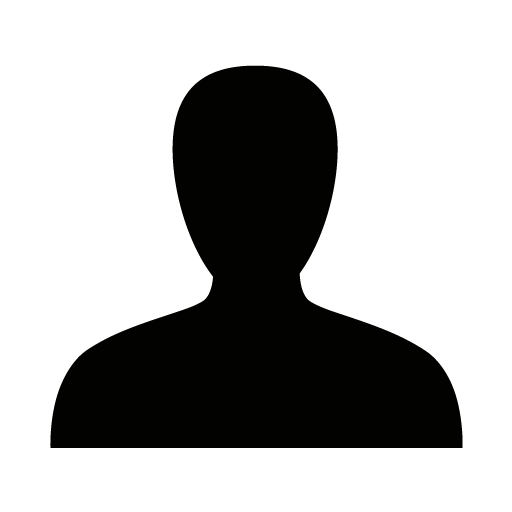
Metal-air batteries are considered a viable alternative to conventional Li-ion batteries as their air-breathing electrode configuration results in higher theoretical capacity and energy density. Moreover, zinc-air batteries (ZABs) take advantage of low cost, environmental-friendliness and safety, since they work in aqueous electrolyte [1].
However, the main issue with ZABs is the limited performance due to sluggish kinetics of oxygen reduction reaction (ORR) and oxygen evolution reaction (OER), resulting in high overpotentials and low round-trip efficiency. The implementation of electrocatalysts helps to accelerate reaction kinetics and make ZABs more competitive for electrochemical storage applications.
Among a variety of electro-catalytical materials (transition metal oxides, heteroatom-doped carbon, metal organic frameworks, etc.) manganese dioxide (MnO2) is one of the most promising for ORR/OER [2].
In this scenario, we optimized nanostructured MnO2-based Gas Diffusion Electrodes (MnO2-GDEs) using different types of MnO2 micro/nanocrystals obtained by hydrothermal synthesis varying precursors and additives (such as CTAB or ionic liquids, e.g. BMIM-BF4). The use of additives allowed us to show a transformation in MnO2 morphology and crystallographic structure passing from beta-MnO2 nanorods (with a diameter of 200 nm) to alpha-MnO2 nanowires (with a diameter of 20 nm) by exploiting the capping agent capacity of additives during chemical synthesis [3].
In the end, we implemented MnO2-GDEs in laboratory-scale Zn-air batteries to investigate the relationship between MnO2 micro-nanostructure and their electrochemical performance in galvanostatic discharge/charge cycles and with electrochemical impedance spectroscopy in a full-device configuration.
1.2-I1
My main research interest is development of the novel post Li-ion battery systems with a specific focus on the application of organic materials as cathode materials in different multivalent metal anode batteries and development of new multivalent electrolytes.
Multivalent (Mg, Ca, Al) metal anodes offer promising gravimetric and volumetric capacities and are a promising option for future high energy density batteries based on sustainable materials. However, their practical application is severely limited by the difficulty of ion insertion, slow diffusion and the presence of side reactions during electrochemical operation. Organic cathodes, with their relatively soft and adaptable structures, offer an alternative to overcome the limitations of inorganic hosts.[1]
In our work we combine organic compounds with different multivalent non-nucleophilic electrolytes. We started our work with polymers from anthraquinone class, which fit well into the electrochemical stability window of multivalent electrolytes due to their moderate working potential. The long-term cycling stability in combination with insoluble organic polymers and suitable non-nucleophilic electrolytes reaches several hundred cycles. However, there are still some challenges in terms of capacity utilisation and cycling stability that need to be addressed. We aim to improve our understanding of these challenges by analysing the electrochemical mechanism in detail using electrochemical impedance analysis and ex-situ electron microscopy, which reveal limitations in the transport of multivalent ions within the active materials.[2] At the same time, we also focus on the development of active compounds with higher energy density, either through increased capacity or redox potential.[3]
1.2-I2
Docent Moyses Araujo received his PhD degree, in Condensed Matter Physics, from Uppsala University (UU). Thereafter, he has held a postdoc position at the Royal Institute of Technology (KTH) in Stockholm with a distinguished scholarship from the Swedish Research Council (VR). As a recognition of his work in Sweden, he has won three research awards, viz. Benzelius prize (from the Royal Society of Sciences in Uppsala), Ångstrom Premium (UU), and Bjurzon’s Premium (the highest award for PhD thesis at UU). In 2011, he has moved for a postdoc in USA, at Yale University, with a prestigious scholarship from the Yale Climate and Energy Institute (YCEI). In 2012, he has returned to Sweden as researcher at UU and in 2014 he has started his independent research group in the same institution with support from VR through the Young Researcher Grant. In 2018 he has become Docent in Physics at Uppsala University. From September 2020, he has joint Karlstad University as universitetslektor/Associate Professor in condensed matter theory.
The organic electrode materials (OEM) are emerging as a promising alternative to develop greener and sustainable battery technologies. However, significant improvements are still required in their cycling stability, rate capability and energy density. This can only be achieved through a fundamental understanding of the electrochemistry at molecular level establishing the structure-properties relationships. To contribute to this end, we are developing methodologies based on evolutionary algorithms (EA) and artificial neural networks (ANN) at interplay with density functional theory (DFT) based calculations. The EA has initially been employed to predict the structure and electrochemistry of a set of dicarboxylates. In a second stage, we have developed a wider database of organic materials for energy applications that contains information about molecular geometries and high-level features extracted from DFT calculations. Based on this database, we have developed a machine learning approach to predict the redox potentials by giving only chemical species and molecular structures, completely by-passing the computer-demanding DFT calculations. A number of learning algorithms based on ANN have been investigated along with different molecular representations. Here we have also included a layer to predict redox-stable compounds. This machinery has been employed to screen a large organic materials library leading to the discovery of novel cathode materials [1,2], which is actually one of the bottlenecks on the development of organic batteries. The computational materials design platform developed here has the potential of significantly contribute to accelerate the discovery of organic electrode materials with superior properties.
1.2-O1
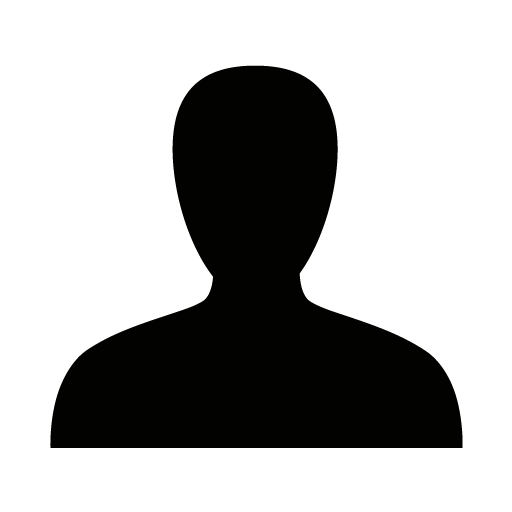
Nowadays, lithium-ion batteries (LIBs) remain the most widely used and manufactured energy storage technology due to their high energy density, long cycle life, and versatility across various applications, including consumer electronics, electric vehicles, and renewable energy systems. However, LIBs face challenges due to limited lithium reserves, toxic cathode materials like cobalt, and unethical mining practices in developing countries, causing environmental and social concerns. Sodium-ion batteries (SIBs) are considered highly promising to replace lithium in storing electrochemical energy from renewable sources and enabling long-range electric vehicles, as sodium is more abundant and widely available. Indeed, sodium-ion batteries face challenges due to the limited availability of suitable cathode materials capable of efficiently hosting sodium ions. Among the promising candidates, covalent organic frameworks (COFs), a class of crystalline porous organic polymers, stand out for their tunable structure, high surface area, and potential to enable reversible sodium-ion storage through redox-active sites.[1] COFs are suitable as electrodes for SIBs due to their insolubility in electrolyte, the possibility to introduce numerous redox-active sites and tunable porosity to facilitate ion diffusion.[2],[3] Herein, we will present the electrochemical performance of an anthraquinone-based COF cathode material in sodium batteries, encompassing cyclic voltammetry, long cycling stability, and analysis of the sodium-ion diffusion mechanism within the material.
Keywords: Sodium-ion batteries, covalent organic framework, organic batteries, electroactive porous materials.
1.2-O2
Dr. Hakan Bildirir obtained his BSc and MSc degrees from Istanbul Technical University (Turkey) and PhD from the Technical University of Berlin (Germany). He worked at Advent Technologies (Greece), TUBITAK (Turkey), Chalmers University of Technology (Sweden) before starting at IMDEA Energy. He developed materials ranging from small molecules to highly crosslinked porous polymeric networks, which were used for separation, latent heat storage, photocatalysis, and organic electronics.
He joined IMDEA Energy in 2021, and is working jointly for the Electrochemical Processes and Photoactivated Processes units to develop materials for energy production and storage.
Developing high-performance electrochemical energy storage systems is one of the most prominent pathways to reach the “net zero emission” goal. For that reason, the demand on batteries has increased exponentially in recent years. Indeed, the conventional batteries based on inorganic materials successfully replied to this request so far. On the other hand, since the R&D efforts have been very intense on such compounds either in academic or industrial level, theoretical limits are almost reached for those conventional systems. Moreover, it is predicted that the increased requirement on such inorganic materials (mostly based on lithium derivatives) may lead scarcity of their raw compounds. It is equally important to mention here that most of these inorganic materials are toxic, unsafe, and unsustainable.[1] Therefore, moving out of the comfort zone to discover novel abundant battery components, which can easily be reached without any geographical limitations, is intriguing. In this context, organic electrode materials (OEMs) are interesting alternatives.
Appearance of OEMs was nearly at the same time with their inorganic competitors. Recently, discovery of molecules carrying redox active side groups (e.g., carbonyls) made OEMs highly interesting in the field.[2] Although their leaching during operations due to their high solubility is always highlighted as a major drawback, forming their polymers neutralized this short-coming.[1] Additionally, going one step further from the traditional 1D polymers to high dimensional (hyper)crosslinked (porous) polymeric backbones make the functional moieties more reachable by the counter ions thanks to the high accessible surface area provided by the empty voids (i.e. pores).[3]
Here we present phenothiazine (PTz)-based hypercrosslinked polymers as high performance and low-cost p-type OEMs, which are rare[4] when compared to the n-types. The syntheses were carried out via a facile method, Friedel-Crafts alkylation (i.e. knitting polymerization),[5,6] in between the low-cost commercially available compounds either to form homopolymer of PTz or its random co-polymers with benzene. The increased benzene portion in the reaction resulted in higher crosslinking, therefore, higher accessible surface areas (BET surface areas from 29 to 586 m2 g-1). Although introduction of the redox inactive benzene reduced the theoretical capacity (from 112 to 77 mAh/g), such addition yielded OEMs with enhanced practicability, including increased durability, operationality in higher C-rates, propensity to increase both polymer content and its mass loading in the electrode. In conclusion, the possibility of such trade-off via macromolecular engineering can be a guide to produce advanced materials with high performance and practicability not only for application in batteries but also in complementary electrochemical techniques.
1.2-O3
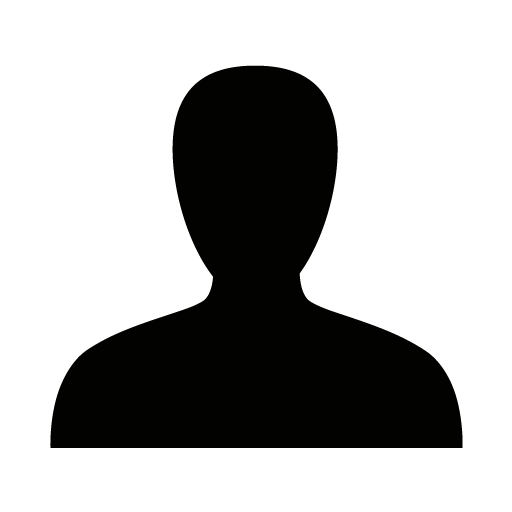
The stability of electrode materials in aqueous electrolytes is crucial for the longevity and efficiency of energy storage systems, especially under conditions that promote water electrolysis. This study examines the self-discharge characteristics of polyimide (PI)-based electrodes in aqueous environments. By systematically examining key factors such as electrolyte type, concentration, operational parameters, and environmental conditions, we aim to identify the underlying causes and elucidate the mechanisms driving self-discharge in organic polymer electrodes. Our findings reveal that water reduction at the electrode not only contributes to reversible self-discharge but also significantly influences irreversible capacity loss. Comparison with nonaqueous systems highlight the pronounced sensitivity of organic electrodes to even minimal water content, which exacerbates degradation. These observations underscore the urgent need for strategies to mitigate both reversible and irreversible degradation processes in aqueous battery systems. Our presentation will discuss these phenomena and potential mitigation strategies in detail, offering new perspectives on enhancing the stability and performance of organic electrodes in aqueous conditions.
1.3-I1
In the first part of the talk, I will introduce recently developed methodology for elucidation of the most important electrochemical performance parameters for high energy density battery materials, including electrode/electrolyte interphase conducitivity and cationic transference numbers from electrochemical impedance spectroscopy, and bulk ion diffusion coefficients in electrolytes and electrodes from galvanostatic polarization. Here, detailed elucidation of spectra and equivalent circuit developement allows for extraction of charge transfer resistances and compext time/temperature behavior. In the second part of the talk, I will focus on application of such methodology on most promissing materials, such as Li/Na/K/Mg/Al metals in contact with contemporary liquid electrolytes, concentrated liquid (e.g. containing triple ions) and polymer electrolytes (above 40 wt% of salt), sustainable sodium cathodes and hard carbon anodes. In the case of metals, the findings show diversity of materials chemistry, with relevance of existence and potential corrosion of native oxide films, as well as dendrites. Finally, I will give an outlook on how to potentially develop future sustainable high performance battery materials.
1.3-I2
Lee Johnson received his first degree from Newcastle University, after which he completed a PhD and post-PhD Fellowship in physical chemistry and electrochemistry at the University of Nottingham. He then joined the research group of Prof Sir P.G. Bruce FRS at the University of Oxford, where he studied the elementary processes taking place within the lithium-O2 battery. In 2017, he was awarded a Nottingham Research Fellowship, University of Nottingham, followed by an EPSRC Fellowship in 2018, both to support study of next-generation batteries. In 2019 he was promoted to Associate Professor in the School of Chemistry. His current research interests focus on understanding interfacial reactions, degradation, and charge transfer, in electrochemical energy devices.
Lithium-sulfur (Li-S) batteries are among the most promising of the ‘beyond Li-ion’ battery technologies, due to their high theoretical gravimetric capacity (1675 mAh g-1S) and energy density (2500 Wh kg-1S), which far exceed those of Li-ion batteries (LIBs). Li-S batteries benefit from the use of sulfur as an earth-abundant, low-cost and geographically widespread positive-electrode material. These systems exploit the reversible 16-electron interconversion of S8 and Li2S at the positive electrode, coupled with the plating and stripping of Li at the negative electrode.
Despite the promise of the Li-S battery, practical cell performance is often limited due to poor interconversion of S8 and Li2S, which is exacerbated at higher rates of discharge and charge. While there are many reasons for this performance limitation, it is generally accepted the battery requires a catalyst at the positive electrode to achieve high energy efficiency and rates. Despite this, the exact role of the catalyst in the Li-S battery remains elusive and the nature of the rate limiting steps is unknown. Here we will discuss the fundamental reaction routes within the lithium sulphur battery. Using a combination of analytical electrochemical techniques we have identify those steps which are rate limiting during discharge of the cell. In addition, we will discuss the development of molecular catalysts able to drive the interconversion of S8 and Li2S. Two types of molecular catalysts will be described; 1) simple redox shuttles and 2) homogeneous catalysts able to trigger disproportionation reactions. A variety of electrochemical and analytical techniques have been utilised to assess the role of the molecular catalysts in sulfur redox chemistry. Using galvanostatic cycling, we are able to demonstrate the impact of these molecular catalysts in cells, which display enhanced cycling performance. Finally, we present an alternative statistical approach to cell data analysis.
1.3-O1
Husam Alshareef is a Professor of Materials Science and Engineering at King Abdullah University of Science and Technology (KAUST). He is also the Director of the newly-established Center of Excellence in Renewable Energy and Storage Technologies at KAUST. He obtained his Ph.D. at NC State University followed by a post-doctoral Fellowship at Sandia National Laboratories, USA.
He spent over 10 years in the semiconductor industry where he implemented processes in volume production for chip manufacturing. He joined KAUST in 2009, where he initiated an active research group focusing on the development of nanomaterials for energy and electronics applications. His work has been recognized by over 25 awards including the SEMATECH Corporate Excellence Award, two Dow Sustainability Awards, the Kuwait Prize for Sustainable and Clean Technologies, and the KAUST Distinguished Teaching Award. He has published over 600 papers and 80 issued patents. He is a Fellow of several prestigious societies including the American Physical Society (APS), Institute of Electrical and Electronics Engineers (IEEE), US National Academy of Inventors (NAI), Institute of Physics (IoP), Royal Society of Chemistry (RSC), and the Institute of Materials, Minerals and Mining. He has been a Clarivate Analytics Highly-cited Researcher in Materials Science for several years.
Ammonium-ion batteries have emerged as a promising energy storage technology for certain applications, offering advantages such as environmental friendliness, cost efficiency, and abundant resource availability. However, the side reactions originating from electrolytes, such as the water decomposition, and host material dissolution preclude their practical applications. Unlike the traditional metal-based aqueous batteries, the idea of “ultrahigh concentrated electrolyte” is not feasible due to the strong hydrolysis reactions of ammonium ions at high concentration.
Our group has been developing innovative strategies including hydrogen bond engineering, electrolyte engineering, and electrode engineering to overcome the challenges faced by aqueous ammonium-ion batteries. The role of hydrogen bond chemistry in stabilizing ammonium ions and improving ionic transport is particularly important, as it affects both electrode–electrolyte interfaces and bulk electrolyte performance.
In this work, we introduce sucrose molecules as electrolyte additive into the ammonium trifluoromethanesulfonate electrolyte. The quaternary hydrogen bond network is formed among cations (NH4+), anions (OTf-), solvents (H2O) and additives (C12H22O11). Such unique hydrogen bond network can inhibit the water decomposition. More interesting, we found that the weak hydrogen bond coordination of NH4+ ions and sucrose molecules allows faster ion diffusion in the bulk electrolytes. This is a new discovery which is very different from metallic charger carriers used in aqueous batteries.
Utilizing such designed electrolytes, the assembled ammonium full battery shows remarkable cycling stability of 2000 cycles at 20 °C and 10000 cycles at −20 °C, respectively.
We believe this work presents a new electrolyte modulating strategy for advancing the practical potential of aqueous ammonium ion batteries.
1.3-O2
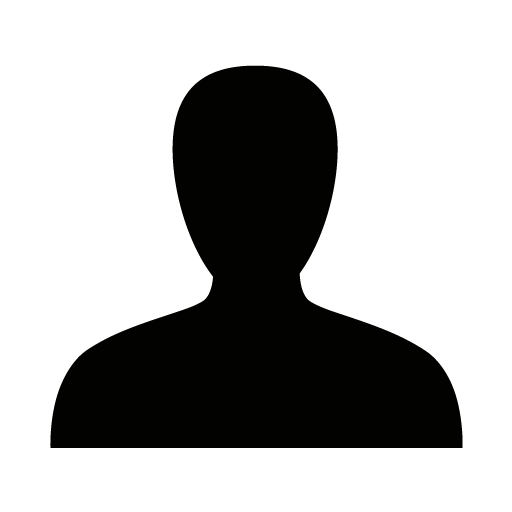
The growing demand for portable devices and electric vehicles has significantly accelerated the development of lithium-ion batteries, driving innovation towards achieving higher energy density, greater power output, and enhanced safety.[1] Currently, graphite anodes, with a theoretical capacity of 372 mAh g⁻¹, are widely used but remain a limiting factor. In contrast, lithium metal anodes offer a much higher theoretical capacity of 3860 mAh g⁻¹, making them promising candidates for next-generation lithium batteries that prioritize high energy density and extended cycle life.[2] However, challenges such as the irreversible loss of lithium due to dendrite formation, whisker growth, and the generation of "dead lithium" pose significant safety risks.
To address these issues, anode-less lithium battery design has emerged as a promising alternative, offered the highest achievable energy density while mitigating safety concerns.
In this study, an ultrathin layer was deposited onto a copper foil using physic-chemical methods, enabling its use as negative electrode in a quasi-anode-less lithium metal battery configuration. Electrochemical testing in a coin cell setup demonstrated notable performance improvements compared to traditional anode-less systems. Detailed morphological and compositional analyses of the thin layer, functioning as a solid electrolyte interphase, were conducted using SEM and XPS techniques. These findings lay a robust foundation for further research, aiming to enhance stability and extend the lifespan of lithium metal batteries through practical design optimizations.[3]
2.1-I1
The European Union has established a policy to achieve climate neutrality by 2050. Therefore, one of the strategies is to increase renewable energy sources drastically and, in turn, develop efficient energy storage devices and batteries of particular interest. For large-scale stationary applications, sustainability and cost-effective batteries are more attractive. In this context, lithium-ion batteries (LIBs) are not the most interesting battery chemistry due to their mass production comes with environmental and social challenges, which will even increase more as the market expands. Indeed, lithium-based technology production involves several critical raw materials, where suppliers concentrate in third countries and conflict zones, and expensive elements (i.e., natural graphite, cobalt, lithium, nickel, etc.) [1,2]. In this scenario, the development of alternative energy storage devices based on sustainable and cost-effective materials is crucial [3].
Sodium-ion batteries (NIBs) are postulated as a complementary technology to lithium-ion batteries (LIBs) for stationary applications and light electromobility due to their lower cost and sustainability, as they are made of non-critical raw materials [3]. Recently, several companies have been presented with potential applications of SIB to bolster their commercialization. For example, CATL, one of the biggest battery manufacturers in the world, recently announced on production of SIBs. Potassium-ion batteries (KIBs) might be another attractive alternative due to they keep the sustainability and cost aspects, with several advantages over NIBs. Potassium, as sodium, is abundant and widely distributed on the Earth’s crust and ocean, while it exhibits lower reduction potential than Na (i.e., -2.71 V and −2.93 V vs. SHE for Na and K, respectively). Moreover, K ions diffuse faster in liquid than Na ions -and Li ions, leading to higher energy density and higher power density than NIBs. The Group 1 start-up has recently released a KIB prototype, and they announced a large-scale production of KIBs by 2027, demonstrating that KIBs could be a reality. Unfortunately, the current performance of KIBs is inferior to that of both LIBs and NIBs. Therefore, further advances are necessary to enhance the viability of KIBs [4]. Thus, this work will be focused on the comparison of Na- and K-based electroactive materials (electrode and/or electrolyte) and identify the key parameters affecting the electrochemical properties to achieve high-performing NIBs and KIBs.
2.1-I2
Na-ion battery technology, developed as a cost-efficient and sustainable alternative to the widely deployed Li-ion batteries (LIBs), has experienced unprecedented growth over the past years. Such rapid development greatly benefited from the earlier advancements in materials chemistry for LIBs, and, therefore, the general understanding of materials could be easily adopted by Na-ion chemistry. However, the use of conventional anode materials from LIBs is challenging, as the most common examples from LIBs (graphite and silicon) do not perform well in Na-ion batteries (NIBs). For that reason, hard carbon was adopted as state-of-the-art and the most common anode material for NIBs. Further improvement of Na storage capacity of anodes required alternative materials, such as alloying materials; however, their cycling stability represents a major drawback for their efficient implementation in NIBs.
The materials operating under conversion/alloying mechanism (where the alloying reaction is triggered by the conversion occurring during the first sodiation) represent a promising class of materials, which can deliver high Na storage capacities without sacrifice of cycling stability. However, the complexity of their operating mechanism(s) severely impedes their development. This presentation will be focused on development and implementation of operando methodologies for characterization of the operation mechanism of these materials and correlating the mechanisms with the electrochemical performances. The challenges associated with the design and characterization of these anode materials will be highlighted.
2.1-O1
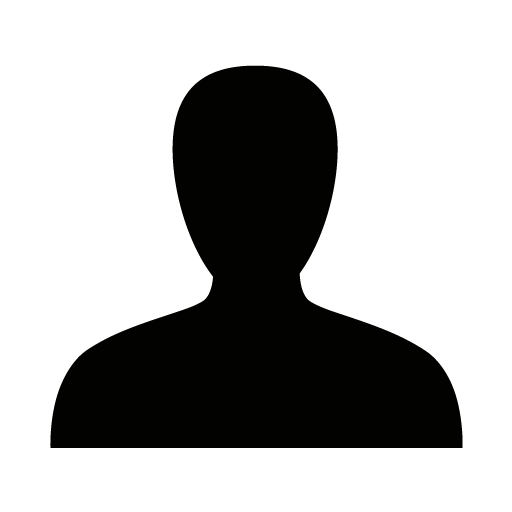
Global population growth and the expansion of electric vehicles have led to a surge in energy demand, stimulating the search for alternatives to fossil fuels and accelerating the transition to renewable energy sources. In this context, metal-sulfur batteries (Li-S or Na-S) have become a promising area of research. Sodium batteries are characterized by their lower cost and abundance compared to lithium, making them an attractive candidate for large-scale applications. However, Na-S batteries face significant hurdles, including sluggish reaction kinetics, the insulating nature of sulfur, and the dissolution of polysulfides resulting in the well-documented "shuttle effect" [1].
To overcome these obstacles, advanced materials for Na-S batteries are being investigated, with sulfurized polyacrylonitrile (SPAN) showing remarkable potential. This carbon-based polymer has favorable electrochemical properties. Through simple and efficient synthesis methods, sulfur is covalently bonded to the polyacrylonitrile (PAN) backbone, eliminating the formation of long-chain polysulfides. This innovation largely mitigates or even eliminates the shuttle effect, resulting in improved stability and capacity in Na-S cells [2,3].
In this study, the textural and morphological properties of SPAN were extensively analyzed after a facile synthesis. Its electrochemical performance was tested using CR2032-type Na-S button cells, and it demonstrated exceptional long-term stability over more than a thousand charge-discharge cycles. Furthermore, it achieved impressive specific capacity values at high charge and discharge rates, surpassing many conventional materials.
This work introduces SPAN as a promising cathode material for Na-S batteries. Its combination of low cost, high sustainability, and scalability positions it as a strong candidate for next-generation energy storage solutions, addressing global energy demands while contributing to environmentally friendly technologies
2.1-O2
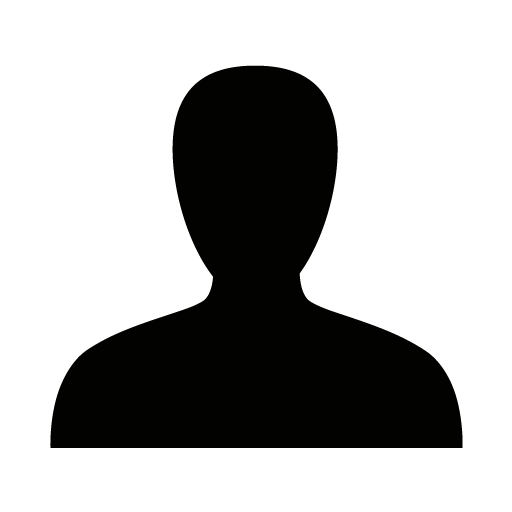
Due to abundant raw materials, low costs and promising high reversible specific capacities, hard carbons (HCs) are a common choice for commercially manufactured anodes in sodium-ion batteries (SIBs). Despite their potential and extensive use, the storage mechanism is still under debate. The non-stoichiometric adsorption mechanism also means that the search for an upper limit for the reversible capacity is ongoing. There is a strong requirement for synthetic anodes that enable a better understanding of the theoretical capacity associated with HC-anodes. We have developed core-shell carbon anodes consisting of a highly porous carbon core and (almost) non-porous shell. Thus, the reversible capacity can be deconvoluted from irreversible capacity losses, arising from the formation of the solid electrolyte interphase (SEI). Moreover, the porosity of the carbon-core can be linked to the reversible capacities gained.
A range of microporous activated carbons were coated via an optimized chemical vapour deposition technique.[1][2] These materials were characterised using a range of techniques including powder x-ray diffraction, small angle x-ray diffraction (SAXS), gas physisorption (N2, CO2) measurements and electrochemical characterisation at coin cell level.
After coating, the material showed a significant reduction in detectable surface area (up to a factor of 192x) by N2-physisorption. The tailor-made shell allows the stable cycling of a carbon anode vs metallic Na-electrode in coin cells at room temperature. For the best performing material, the reversible capacity increased from 139 ± 2 mAhg-1 to 396 ± 2 mAhg-1 while irreversible capacity is decreased from 636 ± 3 mAhg-1 to 89 ± 3 mAhg-1 (see Figure 1). After initial stabilization, a CE of 99% was achieved. The coating technique was usefully applied to a range of materials.
The successful formation of core-shell structures with high capacities enables separation of the storage mechanism from SEI-formation. In turn a proposed calculation to rank the contributions of surface adsorption and pore filling capacity can be confirmed. The materials also create the opportunity to conduct a range of operando experiments (e.g., SAXS) that can shed further light on the sodium storage mechanism.
2.2-I1
Potassium-ion batteries (KIBs) are emerging as a promising option for large-scale energy storage. Compared to Li-ion batteries, KIBs offer advantages such as the abundance of potassium resources and the potential for cobalt-free cathode materials, leading to lower costs. The fast diffusion kinetics of K+ ions in liquid electrolytes may also enable faster charge and discharge processes. Key electrode materials for KIBs include Prussian blue analogues (PBAs), vanadium fluorophosphates such as KVPO4F, and transition metal oxides at the cathode, while graphite and hard carbon are the
most promising anode materials.
This study highlights the use of electrochemical characterization, along with a range of experimental techniques such as X-ray diffraction, Mössbauer spectroscopy, and Raman spectroscopy, supported by density functional theory (DFT) calculations, to analyze the electronic structure and electrochemical mechanisms of these materials for KIBs.
On the anode side, research shows that the mechanism of K+ intercalation into graphite in glyme-based electrolytes changes from co-intercalation to intercalation with increased electrolyte salt concentration, influenced primarily by K+ ion solvation rather than solid electrolyte interphase (SEI) formation. Graphite experiences a significant volume expansion (around 60%) during K+ intercalation, while hard carbon shows limited volume change, leading to superior performance in terms of initial coulombic efficiencies (ICEs) and specific capacities. This can be attributed to the uniform morphology and
higher interplanar distance of hard carbon structures.
On the cathode side, systems such as Mn-Fe PBAs and potassium manganese oxides like K3MnO4 were tested. While these materials are cost-effective and non-toxic, they suffer from Mn dissolution at extreme oxidation states, limiting stable cycling. Similarly, the instability at high potentials of KVPO4F restricts its electrochemical capacity.
These insights, enabled by advanced characterization techniques, especially under operando conditions, underscore the need for a detailed understanding of electrochemical properties and cycling mechanisms to develop new, effective materials for KIBs
2.2-I2
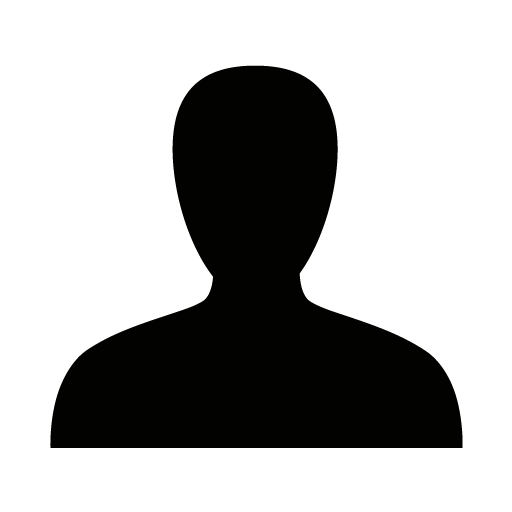
The concept of concentrated aqueous solutions, referred as water-in-salt electrolytes (WISEs),1 brought back recently the interest in aqueous batteries. These electrolytes can be described as few water molecules surrounded by cations and anions. The limited amount of free water molecules, the particular interfaces created,2 as well as the change of the solvation structure strongly modify the solutions behavior. Consequently, the electrochemical stability window is largely extended up to more than 3 V. Multiple strategies have been implemented to extend further the stability window by occupying the water molecules, with the addition of a co-salt (bi-salt electrolyte)3 or the presence of a molecular crowding agent such as a polymer.4 These strategies strongly impact the organization of the water molecules in solution. Similarly, the kosmotropic or chaotropic character of the salt anion5 affects the water network, with the chaotropic anions being more efficient in disturbing the water molecules. Yet, the importance of such strategies on the long-term reactivity of aqueous batteries,6 particularly on the challenging limitation of the hydrogen evolution reaction (HER) at the cathodic side, has yet to be fully demonstrated.
In this context, we performed a screening of the gas production in full magnesium cells as a function of the electrolyte’s nature: imide,7 acetate or perchlorate-based electrolytes, bi-salt electrolytes and polymer-based electrolytes. In particular, we focused on H2 production, used as a universal criterion to assess the relevance of the solutions considered.
At low molalities, we found that the production of H2 follows the kosmo/chaotropic classification of the anions. However, at the highest molalities (or lowest water-to-anion ratios), a similar trend for the production of H2 is observed, whatever the electrolyte considered. While the nature of the anion has a major influence on the solution’s organization, ultimately the H2 production and reactivity seems only guided by the quantity of water in solution.
2.3-I1
Li-ion battery technology is nowadays the selected technology for energy storage of electric vehicles and portable electronics. However, its sustainability and scalability are questionable due to the use of rare elements, such as Co, Ni and Li. Alternatives to the current Li-ion battery technology are under development, such as Li- S, Li-O2, beyond-Li technologies (like Na-ion, Mg and Al batteries) and various organic-based batteries. [1,2]
Redox polymers that can be reversibly oxidized and reduced are gaining much attention as sustainable organic electrode materials to replace the aboved mentioned inorganic scarce materials. The main advantages are that redox polymers can be chemically tuned and biobased, thus enabling materials for new battery technologies such as paper batteries, organic redox flow batteries, polymer−air batteries, or flexible organic batteries. In this talk, we will present the synthesis and characterization of redox polymers having radical groups and a family of bio-based polyhydroxyanthraquinones. Their application as organic electrode materials in Li-Organic batteries will be also presented. [3,4]
2.3-O1
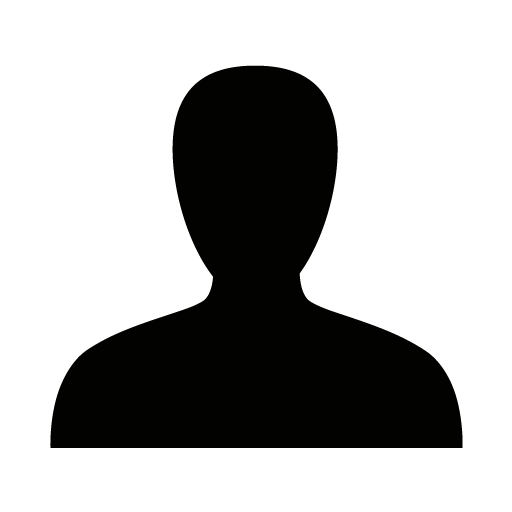
The global demand for electrochemical energy storage has been drastically growing and the projections are a challenge for the entire value-chain of lithium ionlithium-ion batteries.[1] The related challenges in feedstock supply and cost fluctuations waswere driving the research and development on sodium-ion batteries, a drop-in technology using much more abundant raw materials.[2] First commercial SIB cells from HAKADI® Shenzhen Zhonghuajia Technology Co.,Ltd. were available in September 2023 for private users in Europe. The objective of this study is to safely disassemble commercially available sodium-ion batteries (SIBs) and analyse the cell components—cathode, anode, separator, and electrolyte—in terms of their material composition. In this work, two types of SIB cells from different manufacturers were disassembled, examined, and compared. Similar approaches have been pursued by Waldman et al. and Sauer et al.[3,4] However, different cell types are investigated in the current study.
Given the varied nature of the cell components, a comprehensive characterisation requires multiple analytical techniques. After successfully opening the cells, the electrodes were initially measured for dimensions, weight, and thickness. Scanning Electron Microscopy (SEM) was employed to investigate the particle morphology and size of both the cathode and anode. Additionally, Energy Dispersive X-Ray Spectroscopy (EDX) was used to determine the elemental composition of the active materials of anode and cathode. The specific surface area of the active material powders was also analysed.
Thermogravimetric Analysis (TGA) and Differential Scanning Calorimetry (DSC) enabled the determination of the thermal properties of the components. TGA allows for the assignment of characteristic weight losses to specific components, thereby enabling quantitative statements on material composition. DSC, in turn, allows for the identification of characteristic phase transitions associated with specific materials.
The analysis revealed very similar compositions at the material level for both cell types. Only in the electrolyte a difference was observed: one cell type contained three organic carbonates, while the other contained four organic carbonates. Intriguingly, the SEM data for the cathode demonstrated notable differences in particle size and morphology, despite both cathode active materials being composed of the same transition metal oxide, i.e., NaNi0.33Fe0.33Mn0.33O2.
The two cell types (SIB_A and SIB_B) displayed significant differences in cycling stability. SIB_B could be charged and discharged stably over several hundred cycles, while SIB_A lost nearly 10% of its initial capacity after approximately 20 cycles. These differences may stem from cell design, such as insufficient electrode contact or uneven coatings. Alternatively, the discrepancies in cycle stability could arise from material-level differences, such as electrolyte composition. The data obtained so far suggest that the differing cell performance observed may be linked to variations in particle size and morphology of the cathode active materials. These findings provide important insights into which material compositions, particle sizes, and morphologies may be advantageous for the further development of specific SIB cell components.
2.3-O2
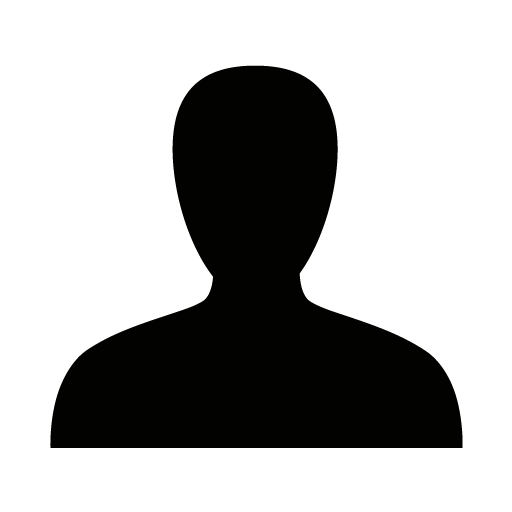
The global publishing landscape has been evolving in recent years, with a notable shift towards openness and accessibility. This has been driving scientists to more openly sharing their discoveries, and undeniably accelerating towards an Open Access world. The Open Access movement has been grounded in key shared declarations and supported by several policy changes at institutional, national, and international levels. However, its influence expands beyond policy. The Open Access movement plays a vital role in addressing the needs of researchers and society by ensuring that knowledge is accessible to all.
In this publishing workshop, we will provide an overview of the Open Science movement and its origins; we will discuss the motivations behind the Open Access movement, highlighting its benefits, both to scientists and the community. Additionally, we will learn about the differences between green, bronze, gold and diamond Open Access, and discuss how they differ in terms of accessibility, costs, and publishing requirements. Finally, we will present the publisher's (Wiley) approach to Open Access, including an overview of our waiver program and our transformational agreements, which aim to make Open Access publishing more accessible.
1.2-I1
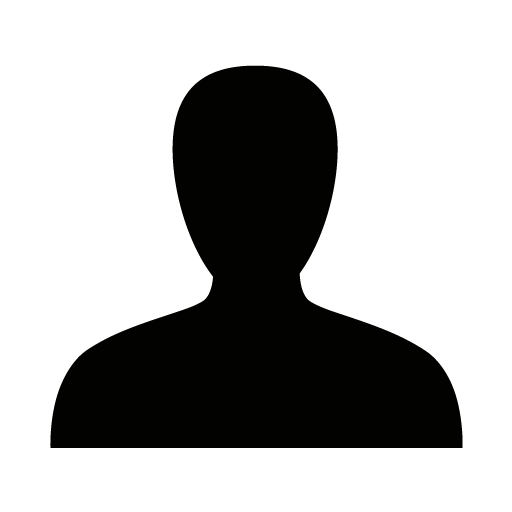
Unlocking the secrets of electrochemical interfaces is key to advancing energy storage materials, yet these processes remain a complex and critical challenge. Among the tools for probing these elusive regions, X-ray photoelectron spectroscopy (XPS) stands out for its ability to analyze electronic structures and characterize the solid-electrolyte interphase (SEI) formed between electrodes and electrolytes. Traditional ex-situ studies (conducted postmortem on electrodes cycled in liquid electrolytes) have showcased XPS as the go-to technique for SEI analysis, thanks to its probing depth (up to 10 nm) matching typical SEI thicknesses [1, 2]. However, these methods often miss transient or intermediate species, crucial for unraveling charge transfer dynamics.
For solid-state batteries, the challenge deepens: the SEI formed between lithium metal anodes and solid electrolytes is buried, inaccessible to direct analysis. Preparing ex-situ samples without disrupting the SEI [3] further complicates the picture. Understanding this buried interphase demands alternative approaches that can monitor its formation and evolution in real time.
Herein, a virtual charging operando XPS (OpXPS) technique was deployed to electrodeposit lithium directly on a halide-based solid electrolyte within the XPS chamber, enabling real-time tracking of SEI formation. Halide solid electrolytes are promising for next-generation all-solid-state batteries, thanks to their outstanding properties such as high ionic conductivity, oxidative stability and ductility. However, their reactivity with lithium metal remains a major challenge [4].
OpXPS revealed the formation of a dynamic mixed ionic-electronic conductive (MIEC) interphase between the halide electrolyte and lithium metal anode. Complementary Electrochemical Impedance Spectroscopy (EIS) and Distribution of Relaxation Times (DRT) analyses provided critical correlations between interfacial resistance and evolving chemical composition. These techniques demonstrate the powerful capabilities of OpXPS in decoding the complex dynamics of buried interfaces, paving the way for designing stable and efficient solid-state battery systems.
1.2-I2
Zinc metal batteries (ZMBs) are promising candidates for low-cost, intrinsically safe, and environmentally friendly energy storage systems. However, the anode is plagued with problems such as the parasitic hydrogen evolution reaction, surface passivation, corrosion, and a rough metal electrode morphology that is prone to short circuits. One strategy to overcome these issues is understanding surface processes to facilitate more homogeneous electrodeposition of zinc by guiding the alignment of electrodeposited zinc. Using Scanning Electrochemical Microscopy (SECM), the charge transport rate on zinc metal anodes was mapped, demonstrating that manipulating electrolyte concentration can influence competing surface reactions and solid electrolyte interphase (SEI) formation in ZMBs. This work show that more extended high-rate cycling can be achieved using a 1 M ZnSO4 electrolyte, and that these systems have a reduced tendency for soft shorts. Using XPS and Raman spectroscopy, it is demonstrated that an SEI is formed on zinc electrodes at neutral pHs, composed primarily of a Zn4(OH)6SO4.xH2O species attributed to local pH increases at the interface. This experimental methodology studying metal battery electrodes is transferable to lithium metal and anode-free batteries, and other sustainable battery chemistries such as sodium, magnesium, and calcium.
1.2-I3
Understanding the electrolyte-electrode interface under realistic operating conditions is critical to designing new-generation battery materials. The past few decades of research have focused on developing a range of spectroscopic and imagining tools to enable the study of the chemical and structural changes at the electrolyte-electrode interface. Here, we introduce operando surface-enhanced infrared absorption spectroscopy (SEIRAS), a promising lab-based tool for gaining a molecular-level understanding of interfacial electrochemical processes.
This talk will bring the most recent understanding of the electrochemical interface during the operation of energy storage applications using operando SEIRAS. We will highlight how the holistic information about the nature of the electrolyte-electrode interface can provide additional knobs to tune the battery performance, leading to further improvements in efficiency and stability for energy applications. Furthermore, fundamentals and experimental tips for operando SEIRAS will also be explained, which is essential for those interested in introducing operandoSEIRAS to probe your electrochemical system, especially battery applications.
1.3-I1
In many applications device performance can be significantly influenced by defects, surfaces, and interfaces, all of which may evolve during operation. Alkali metal ion batteries are no exception, and understanding of how individual atomic scale motifs affect their performance is crucial for development of next generation battery materials. For this, atomic scale modelling play an important part, linking the experimentally observed behaviour to atomic scale mechanisms. In this talk, I will present how we can use the atomic scale modelling toolbox, and discuss the latest results from our group on tackling these challenges, with examples taken from our work on lithium, sodium and potassium ion batteries. Focusing on hard carbon anodes materials, and their interfaces with electrolyte, we will see how surface defects can lead to irreversible capacity and dendrite formation, the effect of pore structure on metal intercalation, and then extend this treatment to investigate the initial stages of the solid electrolyte interphase (SEI) formation. Finally, we will assess how changing the alkali metal ion affects the electrochemical behaviour, what this means for future battery design, and how atomic scale modelling can play an important role in battery manufacturing.
1.3-I2
In-situ and operando atomic force microscopy are powerful tools to investigate various energy storage and energy conversion systems such as batteries, fuel cells, or electrocatalytic systems. By utilizing this method, the solid electrolyte interface (SEI) formation as well as Li intercalation and deposition on anode materials have been elucidated.[1,2,3] Various degrees of heterogeneity are found depending on the exact system under investigation. Importantly, the local mechanical properties of the interfaces that are obtained simultaneously with the topography and are critically discussed in this presentation. Furthermore, the dependency of mechanical properties on the state-of-charge is outlined.[4]
Next to morphological and mechanical information, a full understanding of the local electronic conductivity of electrode materials is of utmost importance. In this contribution, limitations of the conductivity of electrospun carbon nanofibers (CNFs) are presented with respect to the carbonization temperature.[5] A large fraction of the surface of CNFs are found to be not conductive, critically depending on the carbonization temperature. The detected current signals indicate electrically well-interconnected fibers; hence, poor interconnections or heterogeneities of CNF mats are not the limiting factor for an ideal macroscopic conductivity.
1.3-I3
Heather is a Royal Society University Research Fellow in the Department of Chemical Engineering at Imperial College London.
She obtained her PhD in 2017 from Imperial College developing covalent modification strategies on carbon nanomaterials. She was a postdoctoral research associate at Queen Mary University of London and Imperial College, where her research interests shifted to investigating charge storage mechanisms in sodium-ion battery anodes, and later a Faraday Institution Research Fellow, working on the development of engineered carbon hosts for sulfur cathodes in lithium-sulfur batteries.
Heather was awarded a Royal Society University Research Fellowship in 2023, allowing her to establish an independent research team exploring sustainable materials for structural energy storage.
Batteries are expected to play a pivotal role in the electrification of a range of sectors, including transport, aerospace and grid-scale storage. Of the next generation battery chemistries, Li-S batteries are a particularly attractive option due to their projected high energy density, low cost, and operating temperature range. However, the chemistry of such systems is complex, involving the electrochemical conversion between two insulating species (S and Li2S), dissolution and shuttling of soluble intermediates, uncontrolled deposition of cathode and anode species, and large volume expansion; if not carefully regulated, these processes can lead to gradual capacity fade at best, explosive cell failure at worst. Here, we discuss the development of free-standing carbon fibre electrodes that are conductive, lightweight and mechanically robust, and their role in addressing degradation mechanisms arising from volume expansion, polysulfide shuttling, dendrite formation and inventory loss. The fibres were prepared via electrospinning of biomass precursors followed by further heat treatment. The porosity, functionality and conductivity can be tailored by varying the precursor and carefully tuning the treatment conditions, to enable free-standing cathodes with high sulfur loadings, improved redox kinetics and enhanced polysulfide interactions to suppress shuttling and sulfur inventory loss. As anode supports, the carbon fibres provide a lithiophilic substrate for a homogeneous lithium-ion flux and low deposition overpotential, which favours large, uniform and low surface area lithium deposits. Employing UV/vis spectroscopy and optical microscopy to observe operando cell processes, we correlate the surface and structural properties of the carbon fibre electrodes with the ability to suppress polysulfide shuttling and control Li deposition and plating, to allow us to further tune the fibre properties to optimise cycling performance. The assembled cell demonstrates greater capacity retention over long-term cycling than conventional Li-S cathode and anode substrates; additionally, the free-standing configuration allows significant gains in energy density by dispensing with traditional electrode components including the binder, conductive additive and metallic current collector, making this process a promising route to achieving new high-energy-density electrode materials for Li‑S technologies.
1.3-I4
After a master’s in physics, he obtained his doctoral thesis at the Sorbonne University in Paris on nanomaterials and electron microscopy. Then, he worked for 5 years on energy materials and in situ experiments at the Argonne National Laboratory in Chicago. Since 2015, he has been a researcher at the CNRS in the LRCS laboratory and RS2E network (French Battery Network) in Amiens, France. As senior scientist, he studies the dynamics of lithiation in Li-ion battery materials in multi-scale and multimodal modes based on the development of in situ/operando methods via TEM (liquid & 4DSTEM) and X-ray techniques (STXM-XANES). His research is now heavily involved in the use and development of machine/deep learning tools for computer vision, image processing and big data analysis. He is also responsible for the electron/X-ray microscopy platform of the RS2E network and recently co-founder of the startup PreDeeption on predicting battery life, for which he was awarded the CNRS innovation RISE prize.
The structural accommodations, essential to the processes of insertion of the Li-ion inside the materials of the battery, are strongly dependent on the kinetics of the electrochemical reaction. Thus, the quantification of these structural inhomogeneities, their interfaces and their evolutions according to the state of charge and the electrochemical cycling regime is the key to better understand the processes at the origin of the degradation of the retentions in capacity for the Li-ion batteries. For example, knowledge of the spatial distribution of phase, orientation, grain boundary, and strain is crucial to obtain a complete picture of the phenomena occurring during material operation.
Recently, new in situ/operando analytical tools have been developed to monitor structural and chemical transformations, which have allowed important advances in the knowledge of dynamical processors. Liquid cell TEM is a developing technique that allows us to apply the powerful capabilities of the electron microscope to image and analyze materials immersed in liquid. The liquid/bias cell (Protochips) consists of silicon nitride windows on silicon support called E-chip, which separates the liquid from the vacuum of the microscope and confines it in a thin enough layer for TEM imaging. The importance of liquid cell microscopy in electrochemistry is that liquid cell experiments allow direct imaging of key phenomena during battery operation and relate structural and compositional changes to electrochemical behaviors. Different interesting results on monitoring dynamical processes occurring in a wide variety of electrochemical systems, such as LiFePO4, NMC811, LMNO and solid state will be presented here.
4D-STEM techniques yielding structure and strain maps were used to locally probe the crystallographic information of active material crystals and correlate it to the spatial occupancy of Li-ion during the electrochemical cycle. Liquid mass spectrometry analysis was also used to monitor the evolution of the liquid electrolyte during the formation of SEI on the surface of the negative electrode. Structural refinement of an individual cathode grain was achieved using 3DED (electron diffraction tomography) techniques revealing changes in lattice parameters after an in situ electrochemical delithiation process. The ability to couple emerging analytical techniques with liquid electrochemical cell TEM paves new way in energy material characterization, in particular in the study of the dynamic phenomena occurring during the operation which are until now inaccessible to the primary particle scale.
1.2-I1
In this talk I will present our research on developing susianable anode free Na and Li batteries. I will present fundamentals of Li/Na nucleatrion on various curent collectors along with SEI characterisation in different electrolytes and full cells at coin and pouch level. In particular several characterisation techniques such as in operando optical microscopy, solid state NMR, ToF-SIMS, Hard ray XPS will be discussed. The impact of the chathode choice and SEI and full cell performance will also be discussed. In particular for Li we will discuss pairing anode Free Li with a Li2S8/carbon composite and full cell performance.
If time allows will also doscuss our reseacher on K ion batteries snd the inetrcalation into graphite with new insights provided into thickness change, SEI, intercalation mechanism and stage compounds. Characterisation methods such as in operando XRD, Rama and surafec characterisation via ToF-SIMS will be presented along with DFT calculations to back up the Raman results on intercalation compounds with geaphite.
1.2-I2
Marta Sevilla is a Scientific Investigator at the Institute of Carbon Science and Technology, which belongs to the Spanish National Research Council (CSIC). She obtained her PhD degree from the University of Oviedo/INCAR in 2008 working on the development of novel carbon materials for energy storage (supercapacitors) and energy conversion (electrocatalysts for the anode of fuel cells). After several research stays in the University of Nottingham, Max-Planck Institute of Colloids and Interfaces, and Georgia Institute of Technology, she got a permanent position at INCAR-CSIC.
Since 2012 se has focused her research on the development of advanced carbon-based materials through sustainable routes for their use in energy storage devices, including supercapacitors, Li-S batteries and hybrid ion capacitors, energy conversion (ORR electrocatalysts) and gas storage (CO2 and H2). She has co-authored ca. 130 peer-reviewed papers.
Na-ion batteries have entered the energy storage field to confront the problems posed by the exponential demand of Li-ion batteries, i.e., price rise, materials scarcity, geopolitical dependence, and mining and extraction environmental impact. Luckily, Na-ion batteries commercialization has just begun. However, they still have a long way ahead of them for further improvements in energy and power density, as well as cycling stability, in order to shorten the performance gap with Li-ion batteries. Similar to their Li-counterparts, carbon is the star material in the anodes. In this case, hard carbon is the material showing the best performance, although still allowing for optimization in terms of capacity, stability and synthesis sustainability. Indeed, innovations in materials design and performance advances should go hand in hand with technical feasibility, as well as health and environmental protection. Accordingly, to ensure their potential commercial viability and comply with growing requirements on sustainable manufacturing and use of resources, research must focus on the development of greener, simple synthetic approaches that exploit abundant and renewable carbon sources, as well as benign or low-toxicity chemicals.
In this communication, we show several sustainable synthesis strategies towards S-doped disordered carbons with a high-rate performance in sodium storage [1-3]. S-doping has been selected as a tool to boost the pseudocapacitive storage of Na and coupled with nanostructuring to minimize the solid-state Na diffusion distances. As carbon precursor, renewable biomass or biomass-based substances have been used, such as glucose, tannic acid, cork or pistachio shells. As S dopant, environmentally benign sulfur or magnesium sulfate have been selected, while nanostructuring has been achieved by using benign and water-removable templates such as sodium salts (carbonate or chloride) or harnessing the intrinsic structure of the biomass. It is shown that S-doping promotes not only the redox activity at high potentials, but also the low potential capacity exploitable in Na-based technologies.
1.2-O1
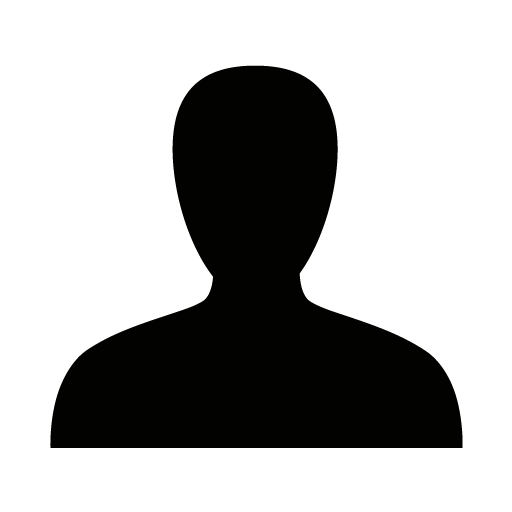
Sodium-ion batteries (SIBs), as one of the alternatives to lithium-ion batteries (LIBs), have developed fast in the last ten years, and some companies have started commercialising SIBs. To achieve ecological sustainability, it is necessary to recycle spent SIBs. However, there are limited studies about the direct recovery of SIB’s anode and cathode active materials. For anode materials, hard carbon (HC), has comparable capacity with graphite in LIBs, and is widely used in commercial SIBs. However, due to its low yield and high energy consumption, HC is expensive than graphite and produces CO2 during its manufacture. It is, therefore, useful to recover HC from end-of-life (EOL) cells and reduce CO2 emissions.[1] In this work, HC was reclaimed from scrap coatings and EOL cells and recovered by low temperature annealing with N2 protect. The HC structure was evaluated by Wide Angle X-rays Scattering and the results confirmed the effect of heating temperature on the graphene layers in the HCs. The electrochemical measurements confirmed that the recovered HC from the scrap after 300°C annealing retained a similar capacity level with pristine HC after 50 cycles at 100 mAg-1. HC recovered from EOL cells showed a reversible 218 mAhg-1 capacity after 50 cycles. In full-cell configurations, HC reclaimed from scrap and EOL cells retained 86% and 89% of their initial discharge capacity after 200 cycles, respectively, and exhibited better cyclability than pristine HC. This research demonstrates a simple and effective single-step direct recovery method for HC recycling.
1.3-I1
Dr Rosa M. Cuéllar-Franca is a Senior Lecturer (Associate Professor) in the Department of Chemical Engineering at The University of Manchester. Prior to her appointment, she held a postdoctoral research position at the same institution for 2.5 years, working on the programme grant “A coordinated comprehensive approach to carbon capture and utilisation” led by the University of Sheffield and funded by the UK’s Engineering and Physical Sciences Research Council. She received her PhD in Chemical Engineering and Analytical Science in 2013 and her MSc in Environmental Technology in 2008 from The University of Manchester, and her Bachelor’s Degree in Chemical Engineering from the Technological Institute of Tijuana, Mexico in 2007.
Her research focuses on the sustainability assessment of novel technologies for climate change mitigation on a life cycle basis, providing quantitative evidence that enables targeted improvements at various system levels, such as molecular and process design, process operation, and policy making. Her work has centred around developing more environmentally sustainable ionic liquids, catalysts, bio-based chemicals and nanomaterials for cleaner technology development. She is an expert in life cycle assessment (LCA), carbon footprinting, and life cycle costing. She is author of over 30 peer-reviewed papers and her recent article on ionic liquids “A life cycle approach to solvent design: Challenges and opportunities for ionic liquids – application to CO2 capture” has won the Reaction Chemistry & Engineering 2021 Outstanding Early Career Paper Award in recognition of her potential to influence future directions in the field.
The development of carbon capture, storage, and utilization (CCS&U) technologies is critical for mitigating climate change and reducing reliance on fossil-based feedstocks. To ensure these technologies deliver sustainable solutions, it is essential to adopt sustainability assessment tools early in the design process, enabling timely identification of improvement opportunities and potential unintended consequences. Innovation efforts have focused on alternative solvents for CO₂ capture and novel catalysts and reactive systems for CO₂ utilization, addressing key technical and sustainability challenges in CCU.
This presentation highlights examples where life cycle assessment (LCA) was applied in early design stages to guide the sustainable development of carbon capture sorbents and CO₂ utilization systems. The first case study evaluates the environmental impacts of 1-butyl-3-methylimidazolium acetate, an ionic liquid (IL), used as a post-combustion CO₂ capture solvent in a power plant with CCS. Key impact contributors and design parameters that could enhance IL performance in CCS processes were identified, revealing molecular-level weaknesses and opportunities for improvement. While the findings focus on a specific IL, the approach is broadly applicable to other ILs if process design data is available.
The second case study demonstrates the use of LCA to guide the design of a non-thermal plasma (NTP) reactor for CO₂ hydrogenation to methanol. By integrating climate impact metrics with reactor design parameters, product selectivity and CO₂ conversion, optimal operating conditions were determined from a sustainability perspective. The reactor, packed with a novel copper-zinc catalyst on a zeolite support, was tested under various voltages. Results showed that methanol selectivity had the greatest influence on the reactor’s environmental performance.
These case studies illustrate that addressing trade-offs early when designing solvents and catalysts applied to CCS&U technologies, can significantly enhance their environmental sustainability performance and drive innovation.
1.3-I2
Dr. Lathe Jones is a Principal Scientist (Investigador Cientifico) at CSIC-ISQCH in Zaragoza (Spain).
His background is in aplied electrochemistry, inorganic chemistyr, and the recovery of metals from ores and waste.
The global increase in solar panel production has outpaced the development of effective recycling processes for end-of-life (EoL) panels. With only a 25-30-year life span, Australia alone will generate around 1500 kilotonnes of solar panel waste by 2050. The substantial quantity of waste generated diminishes the environmental benefits. To tackle this issue, product stewardship schemes have been implemented in Australia, the EU and several states of the USA, establishing an economic imperative to ensure, at minimum the sustainable disposal of the waste.
Silicon solar modules are composed of glass aluminium polymers and metals/semiconductors (including relatively valuable Cu and Silver), as well as hazardous materials such as lead, tin, copper and arsenic.
This seminar will present the recent status of recycling procedures for solare cells, and details of our international collaborations on the identification of strategies to recycle solar cell components into useful end use products, as well as recovery of valuable components.
1.3-I3
Nicolas Schaeffer is an assistant researcher at CICECO - Aveiro Institute of Materials (University of Aveiro, Portugal). He received his PhD in Environmental Engineering from Imperial College in 2017 and is now working as an assistant researcher at CICECO. He is the recipient of an ERC starting grant (DESignSX - Grant agreement ID: 101116461) and his leading a work package on the recycling of LIBs in collaboration with industry. His research focuses on the understanding and development of new solvents for improved hydrometallurgical separation of metals, with a focus on solvent extraction and the forces driving selectivity.
The increasing electrification of modern society is heavily dependent on electronic and energy storage devices, with lithium-ion batteries (LIBs) playing a pivotal role. Since their introduction to the market, their features — such as high energy density, large capacity, long cycle life, and minimal maintenance requirements — made them indispensable to the energy industry. As a result, the demand for LIBs is expected to grow by over twenty percent yearly by 2030,[1] stimulating demand for critical metals like lithium, cobalt, nickel, and manganese. End-of-life (EoL) LIBs present a valuable opportunity as a source of these metals, often offering higher concentrations compared to traditional mineral ores,[2] whilst their adequate management minimises environmental pollution, resource losses, and supply chain risks.[3] Although battery repurposing will also play a relevant role, the recycling industry will continue growing in the coming years driven by recent EU legislation (Regulation (EU) 2023/1542), with batteries from 2031 onwards requiring a minimum recycled content of 6 % Li, 16 % Co and 6 % Ni and further increased by 2036.
This presentation showcases the recent work at CICECO exploring the transition of EoL LIBs down the waste hierarchy, exemplifying reuse strategies for the cathodic black mass before advancing to hydrometallurgical recycling with a focus on exploiting non-aqueous solvents for the design of alternative recovery processes.
2.1-I1
Professor Emma Kendrick, CChem FIMMM FRSC FIMMM - Chair of Energy Materials, School of Metallurgy and Materials, University of Birmingham.
Prof Kendrick’s career to date has included industrial and academic roles leading to her current role as Chair of Energy Materials, where in addition to group lead of the energy materials group (EMG), she is co-director of the Centre for Energy Storage (BCES) and part of Birmingham Energy institute (BEI) and Birmingham Centre for Strategic Elements and Critical Materials (BCSECM). The EMG investigates sustainability in novel battery technologies from materials, manufacturing, performance and parameterisation, and recycling. Her recent work has led to a 2021 joint UoB - Imperial College London (ICL) spin out company, based around the methods of experimental parameterisation of applied multi-physics cell models, called About:Energy, for which she is founder and director.
Prior to UoB, she spent two years as Reader in WMG, University of Warwick. Before academia, she led innovations in the battery industry, latterly as Chief Technologist in Energy Storage at SHARP Laboratories of Europe Ltd (SLE) and prior to that for two lithium-ion battery SMEs, Fife Batteries Ltd and Surion Energy Ltd.
She is fellow of the Royal Society of chemistry (RSC) and Institute of Metals, Mining and Materials (IoM3). Recently, she has been recognised through several awards; 2021 Faraday Institution (FI) Researcher Development Champion, RSC 2021 Environment, Sustainability and Energy Division Mid-Career Award, and the 2019 Hothersall Memorial Award for outstanding services to Metal Finishing.
Prof Kendrick holds a PhD from Keele University, obtained as part of a postgraduate transfer partnership (PTP) scheme with CERAM Research, a MSc in new materials from the University of Aberdeen and a BSc in chemistry from the University of Manchester.
The growing demand for lithium-ion (LIBs) and sodium-ion batteries (SIBs) to support the global energy transition has made end-of-life (EOL) management a critical challenge. Conventional recycling methods, including pyrometallurgy and hydrometallurgy, are energy-intensive and environmentally taxing and often fail to efficiently recover all critical materials. Direct recycling presents an innovative alternative, enabling the recovery and reuse of active materials with reduced energy input and environmental impact.
This presentation highlights cutting-edge research on the direct recycling of lower value and lower cost active materials in batteries. For example, hard carbon (HC) and Prussian white from Sodium-ion Scrap and EOL cells, graphite and lithium iron phosphate from scrap and end-of-life lithium-ion batteries, utilizing low-energy and low environmental impact approaches for delamination and relithiation or sodiation.
The discussion will also explore criticality assessments for materials such as lithium, sodium, and cobalt, evaluating their environmental and geopolitical risks in battery manufacturing. Direct recycling emerges as a scalable solution to mitigate these risks, decrease reliance on raw material extraction, and establish closed-loop systems for battery components. Life cycle assessments (LCA) demonstrate significant reductions in greenhouse gas emissions and energy consumption compared to conventional techniques.
2.1-I2
Rechargeable alkaline zinc–air batteries (ZAB) hold great promise as a viable, sustainable, and safe alternative energy storage system to the lithium-ion battery. However, the practical realization of ZABs is limited by their intrinsically low energy trip efficiency, stemming from a large charge and discharge potential gap. This overpotential is attributed to the four-electron oxygen evolution (OER) and reduction (ORR) reactions and their sluggish kinetics.
In this talk, I will show our new concept based on two-electron generation and consumption of hydrogen peroxide at the air electrode. The O2/peroxide chemistry, facilitated by a newly developed low-cost Ni-based bifunctional electrocatalyst, enables fast peroxide generation/consumption, and high energy efficiency, durability, and capacity. The proposed strategy profoundly relies on the availability of bifunctionally active ORR and POR electrocatalysts. Therefore, we developed an efficient, highly stable, inexpensive ORR and POR bifunctional catalyst based on NiNxCy single sites and Ni-based nanoparticles (Ni(OH)2 at the surface under operational conditions in an alkaline environment) engulfed in crystalline carbon. The new electrocatalyst exhibits state-of-the-art activity, selectivity (96 ± 2%) in the 0.15–0.79 V vs. RHE range, and long-term stability (>100 h) for both hydrogen peroxide synthesis (ORR) and oxidation (POR) in alkaline medium. The integration of the bifunctional catalyst in the ZPB results in an ultra-low initial charge potential of 1.28 V at a current density of 2 mA cm–2 (fixed capacity: 20 mAh cm–2) and 1.48 V at a high current density of 50 mA cm–2, corresponding to 97.3% and 74.8% energy efficiency, respectively. The new ZAB operates for at least 1000 h at a capacity of 50 mAh cm–2, demonstrating high durability. In situ measurements combined with theoretical calculations reveal that the bifunctional catalyst stabilizes an adsorbed hydroperoxyl intermediate, which constitutes a crucial step for both peroxide generation and oxidation reactions, allowing the battery’s unique performance. The new design offers substantial progress toward practical ZAB and green, cheap, and efficient electrochemical production of hydrogen peroxide, providing a step toward replacing the existing industrial energy-intensive and toxic process.
2.1-I3
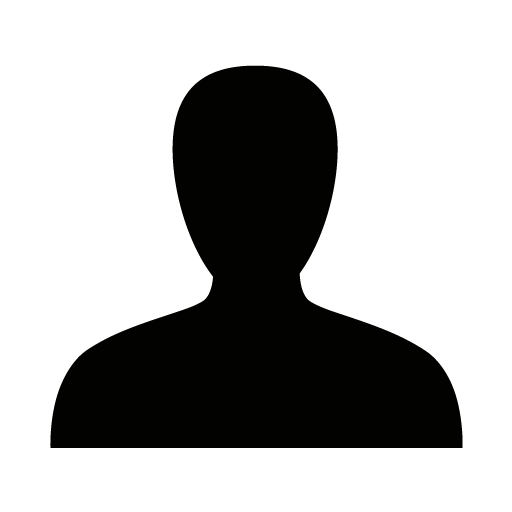
Sodium-ion batteries (SIBs) is one of the more promising battery technologies that are starting to see commercialisation, were the low cost, abundance of necessary raw materials, higher safety and power and similar energy densities compared to lithium-ion batteries (LIBs) are often mentioned as their key advantages. SIBs, due to their great similarities with LIBs have seen a rapid development. One of the key differences, however, is the carbonaceous negative electrode, where in the case SIBs is not possible the use of graphite. This is due to Na+ not forming stable intercalation compounds with graphite unlike for LIBs. But, it was discovered by Jache et al. 1 that Na+ can form stable ternary graphite intercalation compounds by using ethers as the solvent electrolyte through a solvent co-intercalation mechanism, thus enabling the use of graphite in SIBs. Although the co-intercalation of solvent molecules with sodium cation leads to a large increase in the graphite framework, the cycle life and rate capability of the reaction are excellent. 2, 3
This sparked additional interest in the phenomenon, where many electrolyte solutions have now been explored for several metal-ions (Li+, Na+, K+ as well as Mg2+ and Ca2+) showing that when electrochemical solvent co-intercalation occurs the redox reaction, both capacity (stoichiometry) and potential, becomes dependent on the exact electrolyte formulation.4 Hence systems with solvent co-intercalation offers an extremely diverse chemistry.2, 4
This presentation aims to summarize the ongoing research endeavors concerning electrochemical solvent co-intercalation phenomena. Our exploration spans from fundamental inquiries regarding the nature of the reactions involved to methodologies for detecting solvent co-intercalation.5 We also present other electrode materials (in the transition dichalcogenide family) that can be identified as “co-intercalation electrode” when using a specific electrolyte composition (otherwise, conventional intercalation process will occur).6
2.2-O1
Doctoral researcher in the SE-ALM group, with a focus on environmentally sustainable upscaling and recycling of perovskite solar cells. Investigating innovative recycling pathways and applying synchrotron-based techniques, such as EXAFS, to analyze material degradation, reusability, and recovery processes.
The rapid development of perovskite solar cells (PSCs) has significantly advanced the field of renewable energy, offering high efficiency and cost-effectiveness. However, the widespread adoption of PSCs has raised serious environmental concerns, mainlydue to toxic lead-based components, such as lead iodide (PbI₂), which can pose substantial risks if not properly managed at the end of the product’s life cycle. As the need for sustainable practices in the disposal and recycling of these materials becomes more urgent, there is an increasing focus on finding efficient, environmentally friendly solutions for the recovery and reuse of lead from PSCs [1].
In this study, we introduce a novel and sustainable approach for up-cycling lead iodide (PbI₂) from PSCs using environmentally friendly functional liquids. Unlike conventional recycling techniques, which often rely on harsh chemicals and processes that can harm the environment [2],[3],[4]; our method focuses on harnessing green solvent systems to facilitate the efficient recovery and purification of PbI₂, making it suitable for reuse in the production of new PSCs or other applications, thereby closing the material loop and reducing waste.
By employing green solvent systems, the process mitigates the environmental impact typically associated with the extraction and purification of lead but also maximizes the material recovery rate. The effectiveness of the recovery process will be rigorously validated through comprehensive analyses, including structural, chemical, and morphological characterization of the purified PbI₂ to confirm the quality and yield of the recovered material, and demonstrating its potential to meet the stringent requirements for reuse in PSC manufacturing.
This methodology offers a practical and scalable solution to address the toxicity concerns associated with PSC waste [5],[6], and it represents a significant advancement in sustainable recycling practices, providing a pathway to reduce the environmental footprint of PSCs while promoting a circular economy. By improving the recyclability of essential materials like lead—while mitigating its toxicity concerns—this research contributes to developing environmentally responsible solar energy technologies, helping to pave the way for a more sustainable future in renewable energy [7].
2.2-I1
Li-ion battery (LIB) technology is a cornerstone of the transition to a carbon-neutral economy, powering advancements in renewable energy storage and electric mobility. While LIBs have been transformative, achieving full environmental and economic sustainability requires addressing challenges across the entire value chain, including the recycling of spent Li-ion batteries (SLIBs). Current industrial recycling processes primarily focus on recovering valuable cathode metals from the black mass. However, graphite, a critical raw material present in significant quantities in SLIBs, is largely discarded, despite its designation as a critical material by the EU. To fully capitalize on the circular economy potential of LIBs, innovative strategies for the recovery and upcycling of spent graphite are urgently needed.
Recycling graphite from black mass leach residue faces significant challenges due to impurities, structural defects, and contaminants that make traditional recycling methods economically unfeasible. This presentation outlines a novel approach utilizing hydrometallurgically-leached black mass residue as a raw material for producing bifunctional oxygen electrocatalysts. By exploiting the residual metal content and defects in the waste graphite we are able to turn a disposal challenge into an opportunity for creating high-value materials. Our research [1, 2] demonstrates the potential of SLIB recycling residue as a sustainable resource for high-performance M-N-C catalyst materials. These novel catalysts, tested in Zn-air batteries, show high power density and long cycling stability, highlighting their applicability in next-generation energy devices, offering a pathway toward mitigating climate change while advancing the circular economy for battery technologies.
2.2-O2
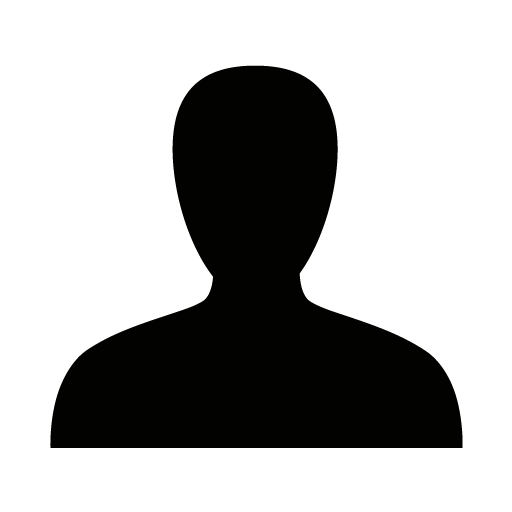
The exponentially growing demand for energy storage has reached a point where it outpaces the energy density of the lithium-ion battery, which is currently the dominant commercial option (~200 Wh kg-1). This has led to an intense search for viable alternatives. Metal-sulfur technology has emerged as a promising candidate for the next generation of rechargeable batteries, offering a high theoretical gravimetric energy density and the additional benefits of low cost and non-toxicity of sulfur [1][2].
Notwithstanding, sulfur presents a number of challenges that impede battery performance, with the shuttle effect and slow reaction kinetics being the most extensively studied. Among the various strategies proposed to address these issues, the chemical trapping of polysulfides (LiPSs) has demonstrated considerable promise [3]. Furthermore, recent studies indicate that applying a magnetic field to materials with ferromagnetic properties can enhance cycling performance [4].
This study presents a novel approach that demonstrates the efficacy of combining recycled ferrite with an external magnetic field generated by a permanent magnet in significantly improving reaction kinetics and polysulfide adsorption, thereby enhancing electrochemical stability. A comprehensive kinetic analysis indicates that the external magnetic field reduces polarization, increases the Li+ diffusion coefficient, and reduces the activation energy between electrochemical stages. The electrode demonstrates a capacity retention of up to 40% and a capacity loss per cycle of only half that observed at a high rate of 1C. At an ultra-high rate of 10C, it maintains a capacity of 507 mAh g⁻¹ after 150 cycles and delivers an areal capacity of up to 3 mAh cm⁻² with an ultra-high loading of 13 mg cm⁻². In addition to its impressive electrochemical performance, this method is more sustainable, utilizing recycled electronic waste processed through dry milling, thus eliminating the need for fossil-derived carbons.
2.2-O3
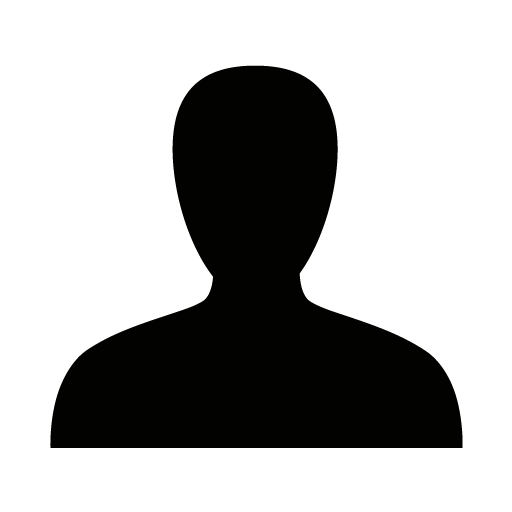
Widespread adoption of alkali metal ion batteries poses a challenge for the recycling industry. Efficient recovery and reuse of valuable metals from end-of-life batteries and manufacturing scrap is paramount. A novel, cost-effective, fast, and scalable with high purity electrode delamination approach, 'ice-stripping,' is proposed. An electrode is wetted with water and frozen using a cold plate, then peeled. Volume expansion and the increased cohesive strength of the ice over the electrode adhesion results in 100% delamination from the current collector and recovery of electrode coatings with minimal water use, material waste, or damage, in stark contrast to conventional high-temperature methods. Its effectiveness is illustrated with Li-ion and Na-ion battery electrodes comprised of different binder systems, and the scalability is considered for scrap. Direct recycling cases study for Na-ion, Li-ion and manufacturing scrap are presented. This innovation holds promise in meeting the escalating demand for efficient and sustainable battery recycling.
2.3-I1
The role of binders is crucial to achieve high performance and long cycle lifes in next generation electrodes for lithium batteries. Poly(vinylidene difluoride) (PVDF) and its copolymers are the most commonly used binders for the processing of cathodes in Li-ion batteries, due to their good electrochemical stability. However, PVDF requires the usage of toxic and expensive organic solvent N-methyl-2-pyrrolidone (NMP) for the electrode processing and elevated temperatures for drying step, making the cathode manufacturing process environmentally unfriendly. In order to overcome these issues, it is necessary to develop cost effective and eco-friendly binders as alternatives to PVDF.
For this reason, the use of bio-polymers and water-processable polymeric binders is increasingly investigated. In this talk, we will present the development of water processable polymeric binders, such as carrageenan biopolymers [1] and fluorine-free poly(ionic liquids) [2], together with their application as binder in high-voltage NMC811 cathodes. Moreover, polymer binders that can provide additional functionalities, such as lithium mobility and/or electronic conductivity, are important for both lithium-ion and lithium-metal batteries. Here, organic mixed ionic-electronic conducting (OMIEC) binders will be presented based on the conducting polymer PEDOT and ionically conductive poly(ionic liquids) [3] and organic ionic plastic crystals [4], which improve the rate capability and cycling stability of Li-ion batteries.
2.3-I2
Dr. Camélia Matei Ghimbeu is a Research Director at Material Science Institute in Mulhouse (IS2M), CNRS, France. She received in 2007 her PhD from University of Metz, France and TU Delft, The Netherlands and her Habilitation in 2015 from University of Haute Alsace, France. She was awarded in 2017 the CNRS Bronze Medal, in 2018 the Award "Solid-State Chemistry Division" (French Chemistry Society) and in 2019 the award Guy Ourisson (Gutenberg Cercle), for her research works devoted to the design of carbon-based materials with controlled characteristics for energy storage and environmental applications. Author of more than 100 articles and about 150 communications, she is leading the “Carbon and Hybrid Materials” group at IS2M, and she is member of French network of Electrochemical Storage of Energy (RS2E).
Among the anode materials for sodium-ion batteries (NIBs), hard carbon (HC) is attracting particular attention thanks to its advantages: low cost, availability, sustainability and theoretical capacity close to graphite (in lithium-ion batteries, LIBs) [1]. HCs are generally synthesised from eco-friendly precursors, such as bio-polymers and biomasses, ensuring local resources' utilisation. For electrode manufacturing, HC is usually mixed with a polymer binder to be cast onto the current collector and to improve its mechanical stability. The binder plays an essential role in the formation of the electrode/electrolyte interface (known as the SEI), which affects the efficiency, capacity and cycle life of the battery. However, the binder can also cause inconveniences related to its weight, stability and inactive electrochemical storage, reducing sometimes performance. In addition, most of the binders currently used contain fluorine, i.e. polyvinylidene fluoride (PVDF), which is not only difficult to recycle, but also requires toxic and volatile solvents (N-methyl-2-pyrrolidone, NMP) to dissolve it, raising environmental concerns. To overcome this problem, sustainable and green binders/solvents are explored in this work to obtain environmentally responsible and biodegradable electrodes, that can be recycled [2]. The results obtained show that significant optimisation of the electrode formulation is required for each individual binder and that the performance vs. Na/Na+ is strongly dependent on the binder used. In addition, the formation of SEI and its chemical composition is also influenced by the binder [3]. Furthermore, binder-free self-supporting electrodes (SSE) are proposed as a promising alternative to avoid the use of binder/solvent while reducing the price, toxicity and weight of the electrodes [4]. The synthesis of such SSE is quite challenging and, in particular, their performance is closely linked to their properties and the electrolyte used.
1.1-I1
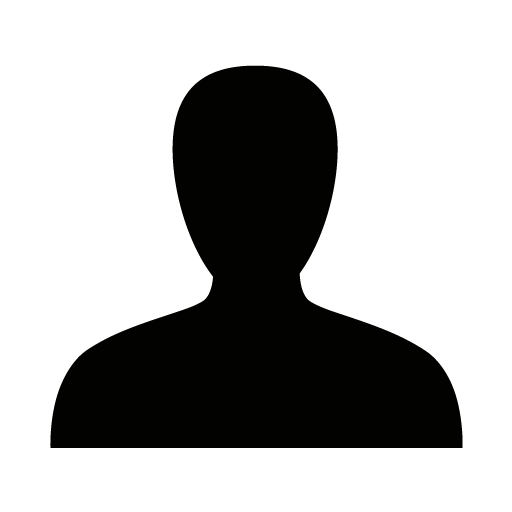
Cs+ and Rb+ have previously been shown to enhance C2+ product selectivity during CO2 electrolysis on Cu catalysts. In a membrane electrode assembly (MEA) electrolyzer, these cations migrate to the cathode surface from the anolyte through an anion exchange membrane (AEM). The long-term durability of the electrolyzer depends significantly on the type of AEM used, as cation transport indirectly influences durability through its role in water transport. Various membrane properties, such as the nature of cationic head groups, ion exchange capacity, and water uptake, play critical roles in determining the diffusion and migration of cations across AEMs. Understanding these transport mechanisms is essential for designing membranes that prevent flooding of the gas diffusion electrode (GDE) while enabling optimal cation transport. In this work, we investigate the transport mechanisms of two specific cations Cs+ and Rb+, across five different ion exchange membranes. We use in-situ wide-angle X-ray scattering (WAXS) measurements to study the dynamic changes in the catalysts, flow field, membranes, and water transport during electrolysis. The transport of cations is understood from X-ray fluorescence (XRF) spectroscopic measurements performed simultaneously. Overall, we map the key components of the electrolyzer during electrolysis using high-energy X-rays including the electrodes, membrane, water, and cations, and how they change as a function of time. Our findings reveal significant changes when different membranes are used, as well as a strong dependence between the Donnan potential and cation transport. A comparative analysis indicates that structural and compositional differences among membranes influence Donnan potential, preferential cation transport, and solvation shell dynamics, thereby impacting the overall electrochemical durability. The insights from this study are expected to guide the design of ion-selective and durable AEMs for advanced electrolysis systems.
1.1-O1
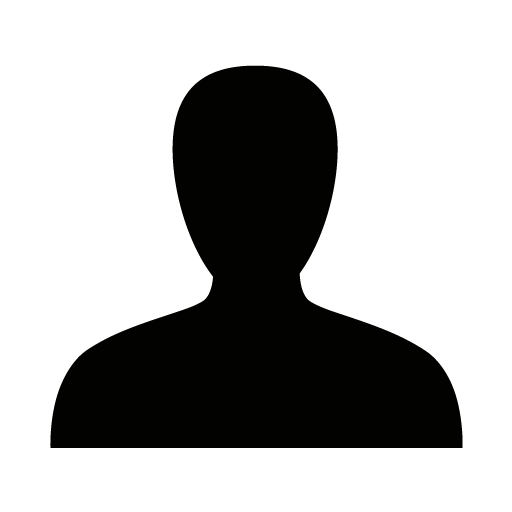
Electrochemical CO2 reduction is one of the potential negative emission technologies for mitigating climate change effects.[1] By synchrotron wide-angle X-ray scattering (WAXS) it is possible to follow transient changes in overall crystallinity and specific crystal phases of the catalyst phase, as well as to monitor background intensity attributed to the electrolyte.[2] By employing a specialized electrolyzer cell, gas diffusion electrodes on top of a microfluidic channel can be investigated along their z axis, delivering a bigger picture of processes in a 2D or even 3D scale down to μm resolution.[3] Precision and interpretation of such measurements, however, depend strongly on the algorithm used to process the vast amount of diffraction patterns. In this contribution, the challenges and possibilities are discussed, including baseline removal and peak picking for complex mixtures in electrolyzer operation. Different methods of baseline removal deliver varying results, indicating the need to optimize data processing strategies. By application of the right analysis method, different Bi-based catalyst materials or perspirating species present at operating conditions.
1.1-O2
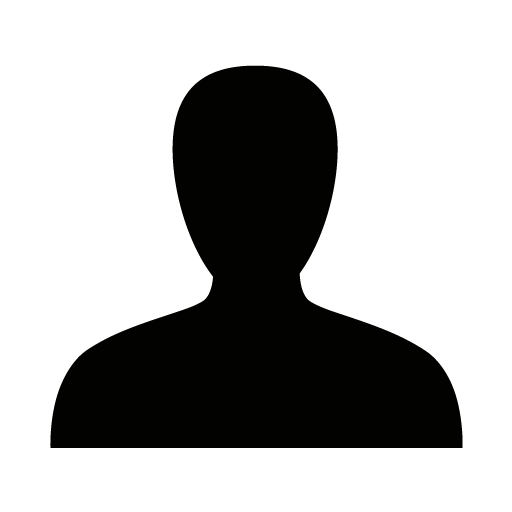
A decarbonized society is essential to maintain and further improve the standard of living for humankind. While the residential sector and short-distance transport have available solutions for electrification, several processes in the chemical industry (such as plastic manufacturing and base chemicals) still require carbon-based fuels as a resource [1].
One of the carbon-neutral technologies to produce base chemicals is electrochemical carbon dioxide reduction (CO2R), powered by renewable electricity. CO2R targets multiple base chemicals ranging from C1- products like carbon monoxide (CO) to C2+-products like ethylene (C2H4) [2].
CO2R is already established at a lab scale. Implementation of gas-fed membrane electrode assembly (MEA) ‘zero-gap’ cells, as the state-of-the-art reactor design, demonstrates promising potential for a scale-up onto the commercial level [3,4]. However, the potential upscale MEA CO2R electrolyzers are inevitably exposed to elevated temperatures, as a substantial part of the input energy dissipates as heat during the CO2R process. Despite the higher temperatures once scaled up, limited research is available on the phenomena and bottlenecks present in CO2R at industrial-relevant temperatures exposing a major knowledge gap in the field [5, 6].
This study presents the effects of industrial-relevant temperatures in MEA-based CO2 electrolysis cells. In the first step, heat balance investigations are shown, proving that industry-size electrolyzers will require heat management and cooling. This acts as a basis for the main motivation why higher than ambient temperatures inevitably need to be investigated. We briefly demonstrate that a corridor of 40°C to 70°C is the most likely operatable range for low-temperature CO2 electrolysis if industry-scale is achieved.
To investigate higher-than-ambient temperatures the usually present experimental setup has to be extended to provide temperature control for lab-scale MEA cells, the input gas, and the liquid electrolyte input. Thus, we will briefly introduce our experimental setup using a heating oven for the MEA cell and external heating of the anolyte reservoir. Additionally, the setup utilizes several devices to enhance the control over the humidity of the incoming gas-feed CO2 to increase the control over the water balance of the system. We explicitly share our experimental setup to lower the entree bar to motivate other groups to expand their ambient temperature testing to industry-relevant temperatures.
Extensive flooding and salt formation are two of the main bottlenecks in the current state of the art for CO2 electrolysis often occurring at the same time or briefly after each other [7-9]. To investigate the effect of higher temperatures on flooding and salt formation experiments in an AEM-based MEA cell, using Ag as the cathode-side catalyst partnered with an oxygen-evolving IrO2 catalyst at the anode, are executed in a current density range of 100 mA/cm2 to 400 mA/cm2 in a temperature corridor from 25°C to 70°C degrees using KOH and KHCO3 as anolytes. Our data shows that elevated temperature benefits the CO2R runtime in MEA cells by diminishing the issue of salt formation, enabling operation at current densities of >200 mA/cm2 even under high electrolyte concentration at temperatures of 50 °C and above. We link this to better solubility at higher temperatures combined with a smaller cation crossover rate. However, flooding remains an issue, especially at current densities higher than 300 mA/cm2. Nevertheless, higher temperatures also mitigate cell flooding time. The exact mechanisms transporting water from the anode to the cathode are currently debated. We suggest osmotic water drag as the main transport mechanism for water transport to the cathode side, backed up by our experimental temperature data with additional control experiments for the osmotic-pressure-relevant variables like anolyte concentration and cation type.
1.1-I2
Sophia Haussener is a Professor heading the Laboratory of Renewable Energy Science and Engineering at the Ecole Polytechnique Federale de Lausanne (EPFL). Her current research is focused on providing design guidelines for thermal, thermochemical, and photoelectrochemical energy conversion reactors through multi-physics modelling and experimentation. Her research interests include: thermal sciences, fluid dynamics, charge transfer, electro-magnetism, and thermo/electro/photochemistry in complex multi-phase media on multiple scales. She received her MSc (2007) and PhD (2010) in Mechanical Engineering from ETH Zurich. She was a postdoctoral researcher at the Joint Center of Artificial Photosynthesis (JCAP) and the Lawrence Berkeley National Laboratory (LBNL) between 2011 and 2012. She has published over 70 articles in peer-reviewed journals and conference proceedings, and 2 books. She has been awarded the ETH medal (2011), the Dimitris N. Chorafas Foundation award (2011), the ABB Forschungspreis (2012), the Prix Zonta (2015), the Global Change Award (2017), and the Raymond Viskanta Award (2019), and is a recipient of a Starting Grant of the Swiss National Science Foundation (2014).
Multi-physical transport processes on multiple scales occur in electrochemical devices and components for CO2 electroreduction. These complex coupled transport processes determine the local environment in the catalyst layer and subsequently also the reaction rates at the catalytic sites. I will discuss how the coupling between atomistic- and molecular-scale models, microkinetic models, and continuum-models can help in improving the understanding of the elecotrchemical CO2 reduction. I will focus on silver as catalysts and discuss how (i) the presence of reaction products can affect the performance, (ii) how the electrolyte nature can affect the selectivity and activity [1], (iii) how pulsed operation can help to improve performance, and (iv) how the mesostructure of the electrode can affect the local conditions [2,3]. All these modeling efforts will demonstrate how multi-scale and multi-physics models can be used to guide the design, operation and material choice for electrochemical CO2 reduction. In fact, such models are essential for the understanding of electrochemical CO2 reduction since experimental investigations cannot provide the detail and locally resolved information required.
1.2-I1
Prof. Dr. Beatriz Roldán Cuenya is currently the director of the Interface Science Department as well as interims director of Inorganic Chemistry Department at the Fritz Haber Institute in Berlin (Germany). She began her academic career by completing her MSc in Physics in Spain in 1998 and a PhD in Physics in Germany in 2001. Her postdoctoral research took her to the Department of Chemical Engineering at the University of California Santa Barbara (USA). In 2004 she joined the Department of Physics at the University of Central Florida as Assistant Professor becoming a full professor in 2012. In 2013, she moved back to Germany and became a Chair professor of Solid State Physics at the Ruhr-University Bochum. She then joined the FHI in 2017.
Prof. Dr. Beatriz Roldan Cuenya is the author of 245 peer-reviewed publications, 6 book chapters and 6 patents. She has been supervising 74 postdoctoral fellows and 36 PhD students. She serves in the editorial board of the Journal of Catalysis and the Chemical Reviews journal. She is a member of the Academia Europaea as well as of the Germany National Academy of Sciences Leopoldina. Recently she received the Manchot Research Professorship from TU Munich (2023), the 2022 Paul H. Emmet Award of the North American Catalysis Society, the Röntgen Medal (2022), the Faraday Medal from The Electrochemistry Division of the UK Royal Society of Chemistry (2022), the AVS Fellow Award (2021) and the International Society of Electrochemistry-Elsevier Prize for Experimental Electrochemistry (2021).
The re-utilization of CO2 via its electrocatalytic reduction (CO2RR) into value-added chemicals and fuels is a promising avenue to minimize the impact of existing technologies on the climate change. This requires the development of low cost, efficient, selective and durable electrocatalysts. However, their rational design requires in depth understanding of the modifications that structurally and chemically well-defined pre-catalysts undergo during operation, especially when the reaction conditions themselves change dynamically.
Here, the transformations that Metal-N-C catalysts (M=Cu, Ni, Co, Fe, Sn, Zn) experience during static and pulsed CO2RR will be unveiled. This will be achieved by a synergistic combination of operando quick X-ray absorption spectroscopy (XAS), high energy resolution fluorescence detected X-ray absorption near edge structure (HERFD-XANES) and X-ray emission spectroscopy (XES), coupled with unsupervised and supervised machine learning methodologies and density functional theory.
In particular, I will illustrate the astonishing behavior displayed by Cu-N-C catalysts during CO2RR, featuring reversible transformations from single atom sites towards small clusters and Cu nanoparticles. The switchable nature of these species, that can be achieved by applying different potential pulses, holds the key for the on-demand control of the distribution of the CO2RR products and thus, a wide-spread adoption of this process. Moreover, I will elucidate the nature of the ligands formed under CO2RR at singly dispersed Ni sites or Co sites in Ni- or Co-N-C catalysts, which are currently drawing great attention for their excellent CO yields.
Overall, my lecture will feature the importance of operando characterization of electrocatalysts in order to elucidate structure/composition-reactivity correlations during CO2RR.
1.2-O1
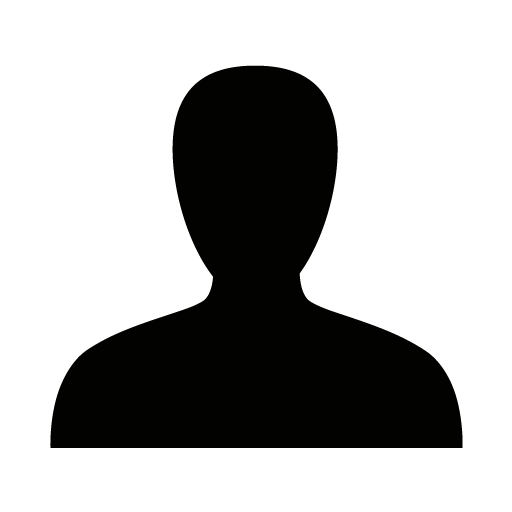
The current state-of-the-art copper electrode lifetime falls several orders of magnitude short from the 10.000 hours industrially relevant timespan.[1] Most copper catalyst cannot maintain their activity towards ethylene and other multicarbon products for more than 100 hours. Contributors to limited catalyst lifetime are copper restructuring[2] , salt formation[3] , flooding[4] and impurity deposition[5]. The restructuring of copper remains one of the least understood mechanisms. Scale up of CO2 electrolysers therefore requires a deeper understanding of the chemical processes leading up to copper instability as well as solutions on both an atomistic and system design level.
Several fundamental works have demonstrated that anodic/cathodic dissolution and redeposition of copper species is one of the main components of the restructuring process.[6] The degree of dissolution and redeposition of the positively charged copper-carbonyl complex was found to proportionate to the applied current density.[7] Based on these results, one would expect that low current CO2 electrolysis within H-cells results in very high electrode stability. Yet, it is the high current CO2 electrolysis in gas diffustion electrode (GDE) based cell configurations that demonstrate the most impressive numbers of operational hours.
In this work, restructuring on a poorly in-plane conducting PTFE based copper electrode is captured through ex situ SEM analysis. It is shown that, over time, copper migrates from the center of the electrode to the perimeter closest to the negatively charged current collector. Subsequently, a nonporous structure erupts, favouring the hydrogen evolution reaction (HER) over CO2 reduction reactions (CO2RR) after 45 minutes to 1 hour of operation at 200 mA cm-2. We then present confinement procedures that are commonly applied within (GDE) based systems to minimize the dissolution of copper species such as metaloxide nanoparticle coatings and ionomers. In addition, the redeposition location is brought closer to the dissolution location by distributing the potential more uniformly across the GDE. Using one or a combination of the outlined strategies allowed the catalyst lifetime to be lengthened to several hours.
1.2-O2
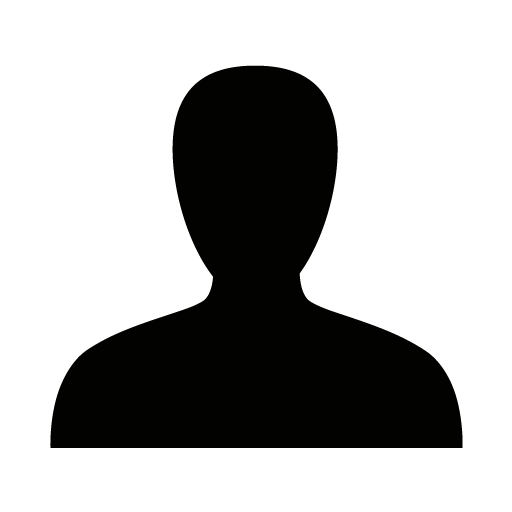
Copper is unique among metals for its ability to reduce CO₂ to high-value products. Despite its often-considered noble nature, copper readily degrades during electrochemical CO₂ reduction (ECR). Through extensive experimental results, theoretical modeling, and literature data, the mechanisms behind copper degradation have been elucidated.1 A high-surface-area Cu catalyst exhibited significant changes in morphology and product selectivity over several hours under constant cathodic potentials relevant to ECR (-0.8 to -1.1 V vs. reversible hydrogen electrode). The formation of copper complexes with CO₂ reduction intermediates was identified as the main driving force behind this instability.
Our findings additionally suggest that these dissolved Cu species preferentially redeposit on sites with lower intermediate coverage, such as adsorbed CO (*CO). A dynamic equilibrium between dissolution and selective redeposition of these copper complexes drives morphological restructuring, leading to catalyst deactivation. This results in a shift in selectivity away from ECR towards hydrogen production during prolonged operation. The interconnected changes in nanoparticle size, crystallographic facet orientation, *CO coverage, and the CObridge vs. COatop ratio were proposed as the key factors contributing to catalyst deactivation.
To confirm the universality of this effect across copper-based catalysts, experiments on electrodeposited copper nanoparticles and copper foil were conducted, yielding similar trends. Understanding these processes is essential for developing strategies to mitigate instability and improve catalyst stability, addressing one of the critical barriers to the industrialization of ECR.
1.2-O3
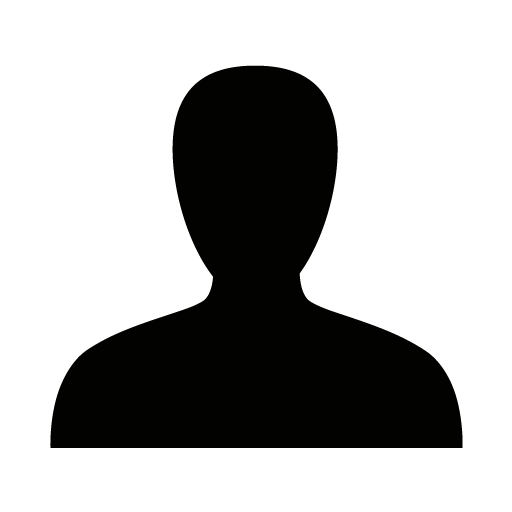
Metal- and nitrogen-doped carbons (M-N-Cs) represent a promising class of low cost electrocatalysts derived from nature-abundant elements for various electrochemical processes including CO2RR [1,2]. Traditionally, M-N-Cs containing active metals (M = Fe, Co, Ni) are synthesized by direct pyrolysis of inorganic and organic precursors, a process that often results in the undesired formation of inorganic side phases through carbothermal reduction, impeding the effective integration of active metals like Fe, Co and Ni. Furthermore, comparing the intrinsic activities of different M-N-Cs can be complicated due to variations in catalyst morphology and active site concentration that arise during the pyrolysis.
To address these challenges, we developed an active-site imprinting strategy in which active metals are introduced post-pyrolysis via ion-exchange [3-5]. In this work, we employed the Mg imprinting strategy to produce Co-N-Cs and Ni-N-Cs with comparable morphology and metal dopant concentration. Our approach allows for a more direct comparison of the intrinsic activities that arise from the metal dopant. The Ni-N-Cs derived this way are consistently higher in activity and selectivity than the corresponding Co-N-Cs, exhibiting a CO Faraday efficiency of up to 95% at potentials between -0.5 to -0.8 VRHE. The Ni-N-C catalyst maintains high stability at -0.65 VRHE, with 92.5% retention of current density and 97.6% retention of CO selectivity after 100 hours of continuous operation.
While Ni-N-Cs are known to be relatively good CO2RR catalysts, the origin of its activity remains rather elusive. Contrary to the widely accepted MN4 active sites in M-N-Cs, NiN4 sites are theoretically inert to both CO2RR and HER due to the instability of the *COOH and *H intermediates respectively, often leading to the proposal of alternative but unproven NiNx sites with various coordination structures to justify their performance. In this study, however, the absence of inorganic side phases allowed us to characterize the tetrapyrrolic NiN4 coordination structure via Extended X-ray Absorption Fine Structure (EXAFS), suggesting that NiN4 sites should in fact be CO2RR-active. Therefore, we performed mechanistic studies on the tetrapyrrolic NiN4 sites using density functional theory (DFT), drawing inspirations from recent studies which elucidated the critical role of cations in enhancing CO2RR activity on noble metal catalysts like Cu, Ag, and Au [6-7]. The presentation will further illustrate the influence of cations on the activity and selectivity of M-N-Cs towards CO2RR, as well as well as the advantage of the pyrrolic nitrogen coordination in promoting the adsorption of these cations.
Overall, this work not only highlights the potential of Mg-imprinted strategies in developing high-performance, morphological comparable electrocatalysts for sustainable energy applications and intrinsic activity studies, but also provides mechanistic insights on the involvement of cations in the valorization of the seemingly inert NiN4 site.
1.3-I1
The green transition requires discovery and development of new catalyst materials for sustainable production of chemicals and fuels. However, it is difficult to predict a material, which might have a high catalytic activity for a given reaction, therefore the development of catalysts up until now has been driven mainly by trial and error. It would increase the pace of development, if we could predict a range of promising materials or if we at least could understand the limitations of catalysis. In this context high entropy alloys offer a chemical space of possible materials where the composition can be smoothly varied and where the properties also might vary in a seamless manner. This is good news for catalysis as such a smooth space is easier to explore to determine the interesting regions in composition space. Furthermore, the highly heterogeneous nature of a high entropy alloy surface reveals fundamental effects which are important for chemistry on surfaces in general, but are overlooked in the classic mean field view on catalysis.
1.3-I2
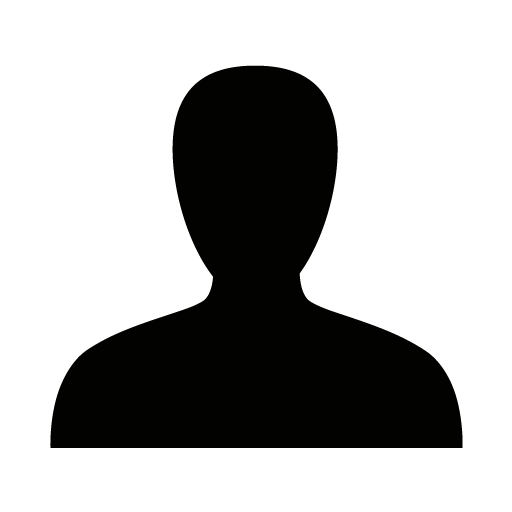
Finding affordable, durable, and efficient catalysts is crucial for advancing green hydrogen production and carbon dioxide conversion, both vital for mitigating climate change. Currently, the discovery of new catalysts is hindered by the gap between computational predictions and experimental outcomes. Traditional catalyst discovery is a slow, trial-and-error process relying on decades of expertise. Analyzing a single material takes time, so different parts of the material design space are often studied separately by various research groups. This results in reproducibility challenges, making it difficult to build on previous findings and draw comprehensive conclusions. Progress requires large, diverse experimental datasets that are reproducible and tested under industrially relevant conditions to bridge the gap in discovery. In my talk, I will highlight the development of the Open Catalyst Experiments OCx24 dataset, featuring more than 500 samples synthesized and characterized by XRF and XRD and tested on gas diffusion electrodes in zero-gap electrolysis for CO2 reduction and hydrogen evolution. To find correlations with experimental outcomes and to perform computational screens, we calculated DFT-verified adsorption energies for six adsorbates on approximately 20,000 inorganic materials, requiring 685 million AI-accelerated relaxations.
1.3-O1
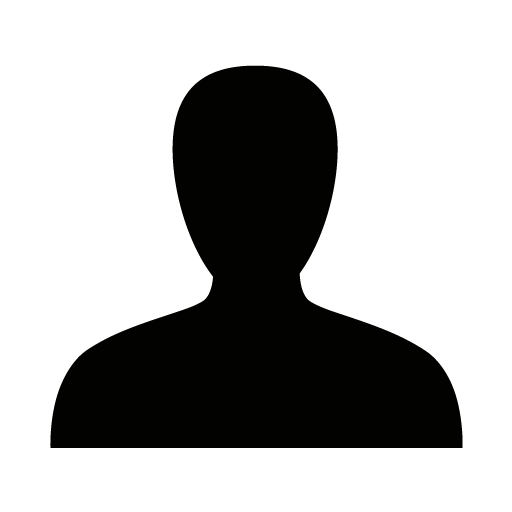
To alleviate CO2 emissions and their impact on climate change, converting carbon dioxide into valuable products such as multi-carbon organic chemicals is of great importance. CO2 can be converted via different pathways such as electrochemical, photo-electrochemical and biological etc. Each approach offers distinct merits but also certain challenges in terms of process efficiency, product selectivity and implementation at scale etc. Therefore, developing coupled CO2 conversion systems, for instance bioelectrochemical reactors, can potentially address some of those challenges.[1] In this work, the focus is on developing cost-efficient, biocompatible, and high activity porous M-N-C catalysts with M = Ni and Co that are atomically dispersed as NiN4 and CoN4 active sites in porous carbon matrix. Ni- and Co-N-Cs are prepared by active-site imprinting approach using Mg as an imprinter.[2][3] Pyrolysis of Mg-N-C is carried out in a salt-melt at high temperatures (≥ 800 oC) and followed by an exchange with Ni or Co at low temperatures. N2-sorption of the materials reveal a micro-mesoporous structure with high surface areas (> 1000 m2 g-1) and a mass-transport enabling pore system. Extended X-ray absorption fine structure (EXAFS) reveal the existence of atomically dispersed single atom active sites with defined active site structure. A variety of Ni-N-Cs and Co-N-Cs were tested for CO2R activity in a rotating disc electrode (RDE) setup, showing high activity and selectivity towards CO2R versus the competing HER. Subsequently, these catalysts were implemented in a home-made bio-electrocatalytical system (BES) consisting of a bioreactor coupled to a CO2 electrolysis cell. Here, CO2 is first electrochemically converted to CO in the electrolysis cell which is then directly fed to bacteria (Clostridium ragsdalei) in bioreactor who further metabolize it to valuable carbon compounds such as acetate. In the BES, partial pressures of CO reached a maximum of 5.7 mbar and that of hydrogen was 2.7 mbar after 30 h. A specific exponential bacterial growth rate of 0.16 h-1 was observed with acetate formation rate of 1.8 mg L-1 h-1 and an acetate concentration of 0.103 g L-1 corresponding to acetate formation rate of 0.73 mmol d-1. As will be discussed in greater details in this talk, we have successfully demonstrated the validity of a coupled bio-electrocatalytical system concept operating with Co- and Ni-N-C catalysts for CO2 conversion.
1.3-O2
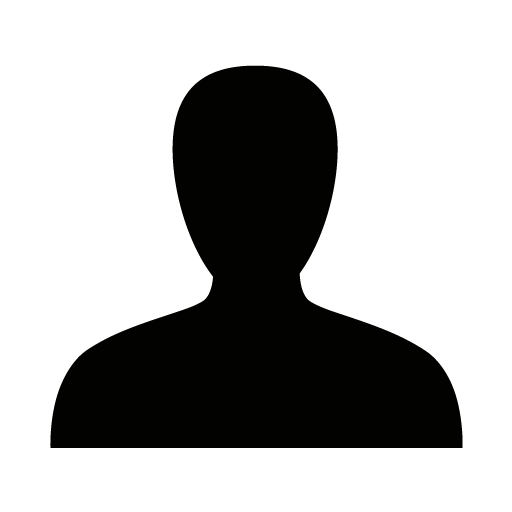
The electrochemical conversion of CO2 has in recent years emerged as a promising technology towards allowing the conversion of CO2 emissions to valuable chemicals and synthons. In recent years, molecular electrocatalysts have risen to the foreground as a tunable, more mass active and selective alternative to transition metal electrocatalysts. Despite the impressive results reached in recent years for a multitude of systems, transferring molecular systems to the large-scale is not yet a smooth endeavor.
Herein, we present our recent efforts towards a holistic transfer of molecular based assemblies, based on the Ag-BIAN (BIAN: N,N′-bis(arylimino)acenaphthene) structure in industrially relevant CO2 electrolyzers operating at elevated current densities ≥ 300 mA cm-2 at 60°C. Notably, we show how the performance and long-term stability of gas diffusion electrodes coated with Ag-BIANs is not only affected by the nature of the ligand system but also the operation conditions. Together with the performance of ex-situ and operando characterization, we show how ink compositions can play a crucial role in unlocking the activity and stability of previously though inactive complexes under electrolytic conditions at 600 mA cm-2.
Furthermore, the currently running and future scale-up projects of Fraunhofer UMSICHT will be presented opening the discussion on the possible up-scaling of molecular systems.
1.3-O3
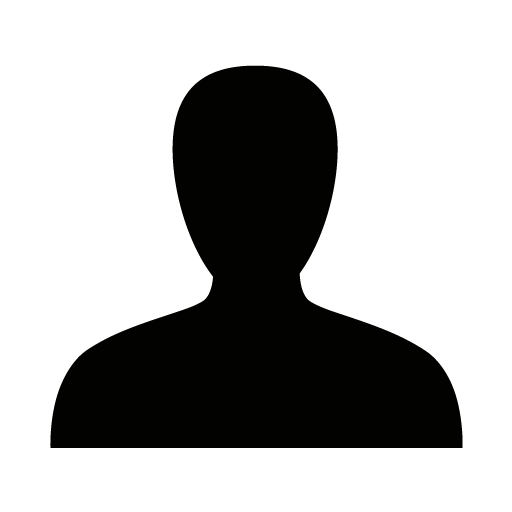
The electrochemical reduction of CO2 offers a promising route for converting renewable energy into carbon-based fuels through CO2 hydrogenation, creating a net-zero carbon emissions energy cycle. Formate is one of the important value-added CO2 reduction products. It is highly demanded in textile and food preserving industries. So, we targeted formate as selective product in CO2 reduction in aqueous electrolyte.[1] Among the catalysts explored, Bismuth (Bi) stands out for being earth-abundant, environmentally friendly, cost-effective, and highly stable, with excellent selectivity for formate production. We developed a controlled synthesis process to produce uniform height 2D Bi flakes with (012) facets, using the chemical reduction of a sacrificial 2D BiOCl template under ambient conditions.[2] These Bi flakes demonstrate an impressive formate partial current density of 18.7 mA/cm² at -1.14 VRHE during chronoamperometry, along with a peak formate Faradaic Efficiency (FE) of around 90% at -0.84 VRHE. However, due to weak solubility of CO2 in water, at higher potential, CO2 saturation at the electrode surface drops which cause severe formate selectivity drop, giving rise to competitive Hydrogen Evolution Reaction (HER). To address this, we strategically modified the 2D Bi flakes with an ultrathin coating of Polyaniline (PANI), a redox-active conducting polymer. The basic amine groups in the polymer chain of PANI attract weakly acidic CO2 molecules to the electrode surface. We found, PANI is not at all active for CO2RR but when it is coated on 2D Bi flakes, it significantly improved performance, increasing the formate partial current density to 35.5 mA/cm² at -1.14 VRHE. Moreover, this modification also reduced the formate selectivity drop at higher potentials in comparison to bare 2D bismuth flakes, demonstrating the synergy between PANI and 2D Bi flakes in enhancing CO2 reduction. We also have postulated a different mechanistic path that shows the role of PANI which is working behind this enhanced activity.
2.1-O1
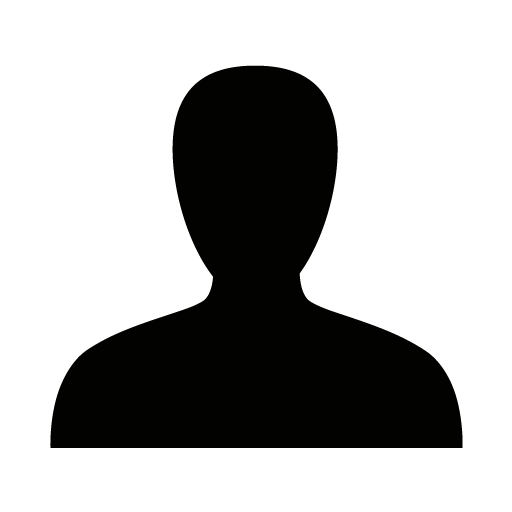
It is widely recognized that CO2 is one of the driving forces behind the climate change. However, CO2 is also a valuable resource for the formation of industrially relevant chemicals, and thus carbon capture and utilization, especially its electrical conversion, is becoming increasingly important. A major challenge for electrochemical fixation of CO2 is the low energy efficiency of electrocatalysts due to high overpotentials. A cost-effective and sustainable material in the field of CCU is nitrogen-rich polymers such as polyethyleneimine (PEI) [1] or polyimidazolium (PI). These polymers are particularly well suited for CO2 adsorption at low partial pressures, which would allow for the direct utilization of industrial flue gas. However, they lack the necessary electrical conductivity. Therefore, the design of hybrid materials combining porous carbons with high electrical conductivity and the CO2-absorbing polymer is key. In this work, these nitrogen-rich polymers were incorporated into carbon substrates via a sonication-assisted impregnation process to improve transport properties and conductivity, both of which are important for subsequent electrochemical CO2 conversion.
The pristine mesoporous carbon substrate was synthesized via zinc oxide-templated carbonization of sucrose at 950 °C and exhibits a specific BET surface area of 1750 m²/g, a high pore volume of 3 cm³/g as well as a hierarchical pore system [2]. These polymer-carbon composites have been characterized by physisorption measurements (N2/CO2), thermal response measurements (Infrasorp) and DRIFTS. All these measurements indicate a strong interaction of these polymer-carbon composites with CO2 even at low pressures. By using a highly porous carbon support matrix, the initial CO2 uptake is increased at least 10-fold compared to the bulk polymer. In addition, the irreversibility of CO2 adsorption is proposed to follow a chemisorption mechanism and thus activate the CO2 molecule.
First electrochemical measurements were performed on a rotating ring-disk electrode (RRDE). The results exemplify how the material can be used as an electrocatalyst for the reduction of CO2. These hybrids were found to exhibit significant selectivity between the hydrogen evolution reaction (HER) and the CO2 reduction reaction (CO2RR), as no hydrogen was detected in CO2-saturated KHCO3 solutions. It is anticipated that, due to their enhanced affinity for CO2, a selective conversion process can be achieved without the need for metallic catalyst centers. Current work aims at transitioning from these fundamental RRDE investigations to more application-oriented measurements in a GDE/zero gap setup [3]. The goal is to enhance the faradaic efficiency further towards CO formation under flue gas conditions by using diluted CO2 as a feed gas.
2.1-O2
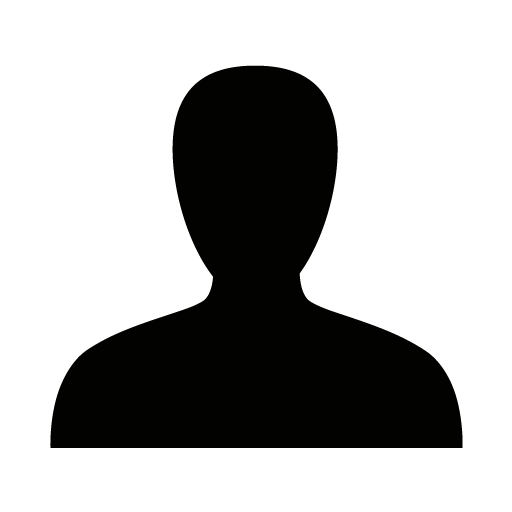
The rising levels of CO₂ in the atmosphere require the development of novel approaches to carbon management. Moreover, the installed capacity of photovoltaic (PV) systems is expanding at a considerable rate, resulting in a significant discrepancy between the generation of electricity from PV sources and the actual demand for electricity. The long-term storage of energy in molecules such as fuels or other industrially useful chemicals is of particular significance in counterbalancing the seasonal variations in photovoltaic power generation. The direct-coupled photovoltaic-electrochemical (PV-EC) system addresses these issues by converting excess PV energy into chemicals prior to feeding it to the grid. This enhances the utilization of photovoltaic power and improves grid stability. In this study, we have designed and tested a direct-coupled photovoltaic (PV) electrocatalytic (EC) device for the conversion of carbon dioxide (CO₂) into carbon monoxide (CO) and hydrogen (H₂) (see Figure 1). The device employs emulated PV that reproduces the IV characteristics of real PV modules in the field at the most relevant irradiance and temperature combinations (Figure 1(a)). The characteristic operating points of the PV-EC were obtained using the NREL public database for silicon heterojunction (SHJ) modules installed in specific regions of the USA. The newly developed PV emulator routine enables the precise and accurate reproduction of any IV characteristic of a PV module, at a level comparable to that of a Class A+ solar simulator. In our study, a SHJ module with an emulated area of 44.5cm² drives a flow-type stack EC cell (area 9.5cm²) with a silver/gas diffusion layer (GDL) cathode and an iridium oxide anode (see Figure 1(b)). The operation of the PV-EC system was evaluated under dynamic conditions, represented by three sunny days in a cycling procedure. This procedure involved eleven steps of irradiance-temperature pairs, ranging from 0.2 Sun to 1.1 Sun and 20°C to 54°C, followed by idle 'night' periods. The operating voltages achieved were in the range of 2.4 to 3.2V, while the operating currents were between 70 and 335mA corresponding to current densities of 7.4 to 35.2mA/cm2. The solar-to-chemical efficiency was observed to range between 8.7 and 10.8% at a high degree of coupling (0.85 to 1) in the absence of power electronics. Finally, a consistent and stable dynamic operation towards CO as the primary product with 75% faradaic efficiency and H₂ (25%) as a by-product over one to three-day cycles was demonstrated. This coupling, in conjunction with the high selectivity towards CO, renders the approach an attractive one for the decentralized storage of excess PV energy, offering a material-saving route.
2.1-I1
(Photo)electrochemical conversion of solar energy represents a sustainable approach for generating chemical fuels, such as hydrogen (H₂) and hydrocarbons. Recent advances have highlighted the potential of PEC systems to drive convert carbon dioxide (CO₂) into various hydrocarbons. Despite these advancements, several critical challenges persist, particularly regarding the selectivity of the systems and the suboptimal efficiency and limited long-term stability of current PEC systems. Overcoming these limitations is crucial for realizing the full potential of PEC technology in renewable fuel production and for its broader adoption within the energy sector.
Here we will share the most recent results in our group on two main aspects regarding (photo)electrochemical CO2 reduction. On the one hand, we will focus on the importance of the microenvironment in determining and tuning selectivity by changing the local environment on metal electrodes or the chemical environment of metal centers otherwise selective for H2 production. On the other hand, we will show examples of stabilized and stable system for CO2 (photo)electroreduction.
2.1-I2
The electrochemical carbon dioxide (CO2) conversion to fuels and chemicals, powered by renewable electricity, presents a compelling avenue for reducing CO2 emissions while facilitating large-scale and long-term renewable energy storage. Over the past decade, there have been promising steps in the production of fuels and chemicals (e.g., methane, ethylene, and ethanol) through electrochemical CO2 conversion. Specifically, advancements in catalyst and system designs have enabled high selectivity for methane and ethylene (>70%) at high current densities (100 – 1000 mA/cm2).
While high selectivity at high current densities has been achieved, the stability of electrochemical hydrocarbon production remains insufficient for practical applications. Copper (Cu)-based materials are the most efficient catalysts for hydrocarbon production, but they experience morphological, structural, and chemical transformations under CO2 reduction conditions, leading to changes in product selectivity.
In this study, we introduce the concept of reversible catalysts for CO2 conversion to methane. This approach leverages the dynamic behavior of copper catalysts during CO2 reduction. Using this method, we achieved CO2-to-methane conversion at a current density of 200 mA/cm², with a methane Faradaic efficiency exceeding 50% sustained over 1,000 hours of operation.
2.2-I1
Electrolysis technologies such as water splitting, CO2 electroreduction, and other emerging reactions, present sustainable alternatives to power large industries such as transport (fuels, e.g., green hydrogen), manufacturing (chemical feedstock) and agriculture. These reactions rely on breaking molecules and reassembling them into desired products with sufficient activity and selectivity. Their viability relies on achieving sufficient performance in metrics in scalable processes.
Conventionally, this has been pursued by innovation at the catalyst level, for example, designing materials with target physicochemical properties. This is challenging, since catalysts change substantially during reaction, which precludes a predictive catalyst design. On the other hand, while water is arguably the main ingredient in water electrolysis, its role at the catalyst environment and in the electrolyte remains underexplored.
I will first present a new strategy to program catalyst reconstruction. Starting from the same precatalyst, this can either lead to unpredictable composition and structure or enable predictable catalysts with improved reliability. I will then focus on the liquid-side of the reaction. I will show examples on how control over water at catalyst interfaces can enable achieving distinct physicochemical properties leading to improved stability during water electrolysis and activity and selectivity during CO2 electroreduction.
To conclude, I will briefly overview sustainability issues in the scale up and path to market of these technologies.
2.2-O1
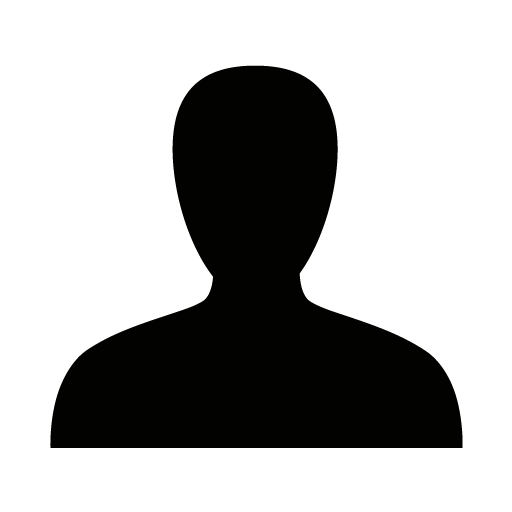
The electrochemical CO2 reduction reaction (CO2RR) has garnered significant attention over the past decade due to its distinct advantages, including operation under ambient conditions, compatibility with renewable electricity, and the ability to produce a diverse array of value-added products. Most CO2RR research to date has utilized pure CO2 as the feedstock. However, real-world CO2 waste streams, such as those in flue gas or biogas, typically contain no more than 40% CO2. This discrepancy poses challenges for the economic feasibility and sustainability of CO2RR, as CO2 purification steps prior to electrolysis—such as CO2/N2 separation—can cost $70–100 per ton of CO2 and significantly increase the carbon footprint [1].
In recent years, integrating CO2 capture with electroreduction into a single unit has emerged as a promising strategy to address these limitations. Many studies have focused on using CO2 capture solutions as electrolytes, achieving encouraging results in improving the overall cost-efficiency of CO2RR. Beyond solution- or electrolyte-based capture methods (e.g., amine solutions and ionic liquids), integrating alternative approaches such as solid adsorption and membrane-based processes into CO2RR systems offers considerable potential. For instance, combining these methods with gas-diffusion electrode designs could enhance the efficiency and practicality of CO2 capture-electroreduction systems [2,3].
Herein, we showcase the scenarios to integrate adsorption and membrane separation within gas-diffusion electrodes (GDEs) and present our recent research data of CO2 conversion by an ionic liquid-mediated CO2-selective GDE. GDEs have been tested with gaseous feed with CO2 concentration as low as 15% containing O2, resembling flue gas, and showed high-rate syngas production with a polymer-ionic liquid selective layer.
2.2-O2
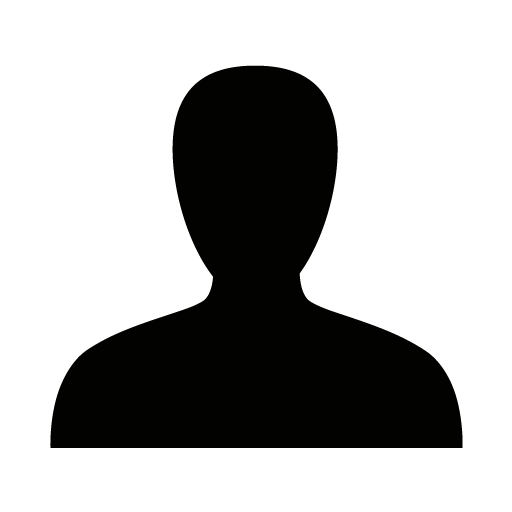
Bipolar membranes under reverse-bias (r-BPM) offer a possibility of using PGM-free anodes in CO2 electrolysers. Under ideal circumstances, the OH- generated from the BPM can fully replenish the OH- consumed by the OER, maintaining a stable anolyte pH over time. However, the OH- regeneration rate, and hence the stability of such systems, is dependent on the Water Dissociation Efficiency (WDE). In non-ideal BPM, the transport of co-ions through the membrane lowers the WDE below 100%. In this case, a pH decrease in the anolyte over time is expected, compromising the long-term stability of PGM-free anodes in CO2 electrolyser.
In our study we aim to explore the feasibility of replacing PGM anodes under industrially relevant conditions. To simulate the long-term stability of a PGM-free CO2 electrolyser, we have developed a methodology that combines both an experimental and modelling approach. Using a MEA cell architecture, we have determined the WDE of commercial BPMs under various process conditions, including current density, anolyte concentration, or cation identity. The experimental results have been used as the model input to extrapolate the long-term performance of a r-BPM CO2 electrolyser. Our results suggest that current commercial BPM WDEs are not high enough to allow for the replacement of PGM anodes in CO2 electrolysers. In addition, we highlight the importance of assuming realistic industrially relevant anolyte volumes when assessing the stability of PGM-free anodes.
1.1-I1
Meeting the growing demand for lithium-ion batteries in electric vehicles and portable devices requires efficient lithium extraction methods. Electrochemical approaches, particularly those that leverage energy-efficient direct lithium extraction, are emerging as promising solutions. This talk explores both continuous and intermittent concepts for lithium-ion recovery, emphasizing advancements in material selection, membrane integration, and electrode stability.
Intermittent lithium-ion extraction is explored using lithium iron phosphate (LFP), a widely available battery material with a high theoretical capacity and favorable lithium insertion potential. Despite these benefits, LFP faces performance stability challenges. Our work investigates the role of additional cations and dissolved oxygen on LFP stability, finding that calcium cations and dissolved oxygen contribute to capacity fading. In contrast, sodium and magnesium cations have minimal impact. Performance is enhanced through continuous nitrogen flushing of the electrolyte and carbon coating of the LFP electrode, resulting in a lithium extraction capacity of 21 mg per gram of electrode material. This approach achieves an energy consumption of 3.03 ± 0.5 Wh per mole of lithium, with a capacity retention of 82% over 10 cycles. This system is of particular use for the processing of hydrometallurgical media within the context of lithium-ion battery recycling.
To continuously extract lithium from seawater, mine water, or other aqueous media, one can use continuous operation of electrochemical systems that integrate lithium-ion-selective ceramic membranes (LISICON). We show the facile operation of a simple redox-flow electrolyte, enabling continuous lithium recovery at a high purity of 93.5% and a Li/Mg selectivity factor of approximately 500,000:1. This concept is not limited to redox-flow battery technology; instead, our work shows that a fuel cell (fueled with oxygen and hydrogen) can also co-produce electricity and separate lithium-ion during continuous operation.
1.1-I2
Dr. Trócoli got his Ph. D based on developing materials for Li-ion batteries at the University of Cordoba in 2012. He started his postdoctoral career by joining the Bochum Universität (Prof. La Mantia). Firstly, working in aqueous batteries, developing the first Zn-ion battery based on CuHCF; lately, he got involved in other fields, including his first works in Li selective and exclusion electrodes, as well as in an industrial project with Bayer MaterialsScience – reactor design. Dr. Trócoli started a collaboration with Prof. Alfred Ludwig to develop thin-film cathodes by RF-Magnetron sputtering, his first incursion in all-solid-state batteries. In January 2016, he joined the Nanoionics and fuel cell group (IREC, Spain, Prof. Tarancón - H2020 “Sinergy” project), working in thin film deposition methods. He developed a new multi-target technique and fabricated, among others, the first double-ion micro battery based on a Li intercalation cathode and a Zn metal anode. In September 2017, Dr. Trócoli started as a Marie Curie fellow in the group of Prof. Palacín (ICMAB-CSIC). Lately, he joined the FET-H2020 project E-Magic, working on novel cathodes for Mg batteries and nitride materials. In 2020, Dr. Trócoli obtained an EMERGIA project (Junta de Andalucía) and a JIN project (Retos-2020) for the development of new materials for Li extraction starting in November 2021 as a senior researcher at the University of Cordoba. Currently, Dr. Trócoli works as a Ramón y Cajal researcher at the University of Córdoba. He led several national and regional projects on battery recycling and post-lithium ion batteries.
A robust supply chain of main Li-ion battery (LIBs) components has become critical since the rapid growth in energy demand storage, the so-called Green transition promoting the market of electric vehicles, and the ubiquitous presence of batteries in portable applications has spread out LIBs manufacturing and components needs. The dependence of these materials on third countries, with China as the main battery grade supplier, has prompted the European Committee to develop a new EU regulatory framework for batteries, which encourages battery recycling as an alternative supply chain, targeting, for example the recovery by 2027 of at least 50% of the lithium contained in spent batteries and its reutilization for the manufacturing of new cathode materials by 2035 at 10%, value increased to 12% and 20%for Ni and Co respectively. [1] Current LIBS recycling is carried out at an industrial scale using pyrometallurgical and hydrometallurgical processes. However, these technologies have several inherent limitations; in most cases, these methods face difficulties in recovering lithium and focus on extracting nickel and cobalt; in addition, they suffer from an intensive consumption of energy or chemical reagents, lengthy operational procedures with low recovery rates, and the generation of hazardous wastewater or polluting gas emissions, thus involving severe environmental impacts [2, 3].
In this communication, we develop an electrochemical method - ion pumping technology- to selectively extract lithium from battery spent; based on the use of lithium-selective-electrodes, such as olivine LiFePO4 (Figure 1), as well as, we have proven the viability of the same technology to recover Ni and Co from NMC spent, in this case based on the use of Prussian Blue Analogues, KxNi[Fe(CN)6]y - z H2O. The materials selectivity and the influence of critical extraction parameters (current density, time…) were analyzed by constant current measurements and Inductively coupled plasma mass spectrometry. The results demonstrate this technology's potential for electrochemical recovery of lithium and multivalent cations in short operational times.
1.1-O1
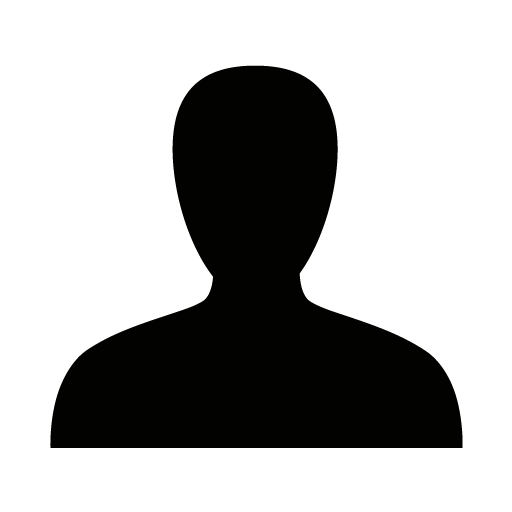
Pyrometallurgy is one of the most relevant processes to recycle Lithium-ion Batteries (LIB). It involves the melting of the waste batteries in a shaft furnace at around 1500ºC. Two main products are obtained: an alloy, rich in Ni, Co, Cu, Fe; and a slag, which mainly contains Li and Al [1]. Typically, this slag was not valorised, and its main application was as a filling material in the construction sector. In 2021, one of the main European companies in the field of battery recycling published a patent where they claim a hydrometallurgical process to recover lithium from this slag, by leaching with H2SO4 and then precipitating Al to leave Li in solution [2]. The present study arose as an attempt to investigate the possibility of lithium extraction from leaching solutions containing aluminium.
Our approach focuses on exploring electrochemical capture technologies, such as faradaic deionization, to minimize the chemical usage and provide a more cost-effective, energy-efficient and environmentally sustainable solution. For that purpose, various lithium metal oxides (LMO, LFP, LMFP, NMC) were tested as active materials for electrochemical lithium recovery. Lithium manganese oxide (LMO) emerges as a promising candidate leading to the synthesis of Truncated-Octahedral LMO (Tr-Oh-LMO) [3], theoretically more stable than commercial LMO, to enhance the robustness of the process. Electrodes were prepared in two configurations, carbon paper (CP) and buckypaper (BP), and tested across different electrolyte compositions and pH values.To assess the performance, a novel Electrode Endurance Diagram was proposed to visualize the influence of pH on electrode stability during cycling. This tool enables a quick and comparative evaluation of the performance of different materials across a range of pH levels. Additionally, various characterization techniques, such as XRD and SEM-EDS, are employed to investigate electrode degradation mechanisms as a function of pH and aluminum presence.
Overall, this work provides valuable insights into the influence of pH and aluminum on the stability of lithium oxide electrodes, emphasizing the need to optimize process conditions and explore alternative active materials to enhance the feasibility of electrochemical lithium extraction from aluminum-rich solutions.
1.1-O2
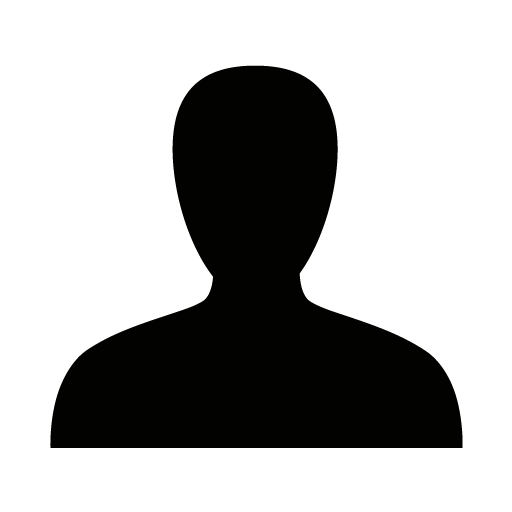
Li-ion battery recycling is currently a topic of significant scientific interest, with numerous studies and research projects exploring new techniques and optimizing existing ones. This is further encouraged to cope with the need for an independent supply of the main battery materials (e.g. Li, Co, Ni), most of them classified as Critical Raw Materials (CRM) by international organisations such as the EU [1]. Battery recycling therefore aims at facing the rapid growth in energy demand and the so-called Green transition promoting the market of electric vehicles. Not to mention that battery recycling tackles environmental problems related to their disposal. Current recycling methods employed are primarily based on hydrometallurgical and pyrometallurgical processes. However, these technologies have several inherent limitations, including an intensive consumption of energy or chemical reagents, lengthy operational procedures with low recovery rates, and the generation of hazardous wastewater or polluting gas emissions, thus involving severe environmental impacts [2]. Consequently, the development of novel alternative and sustainable recycling techniques is imperative, with electrochemical recycling representing a promising avenue for advancement [3].
In this work, an electrochemical technique has been the subject of study using materials like Prussian Blue Analogues (KxNi[Fe(CN)6]y - z H2O), capable of reversible intercalation of divalent cations (Co and Ni). Following the acquisition of encouraging results from 965 ppm of recovered CRM under static conditions in a microcell, the method was fully automated simulating a scenario closer to the final application utilising a semicontinuous flow-through reactor and resulting in the recovery of 1600 ppm CRM and 121 Wh/g of energy consumption. This is accomplished through the integration of electrochemical techniques to study the electrochemical properties of the PBA and to develop the operational modes of the setup. Furthermore, an examination of the material's structural, morphological, compositional, and characteristics is conducted.
Accordingly, this study represents a novel automated electrochemical method for the recovery of nickel and cobalt from battery recycling wastewater
1.2-O1
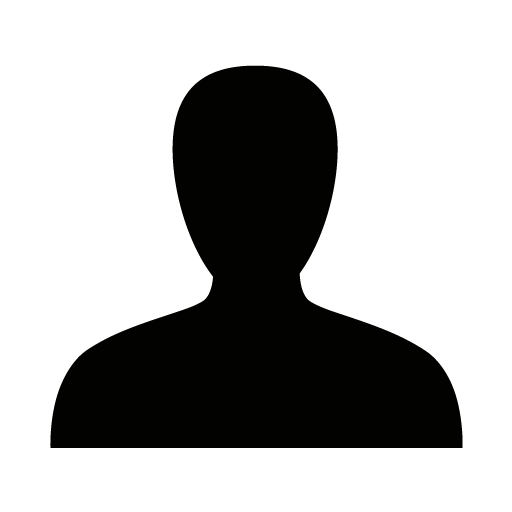
In recent years, there has been a notable increase in lithium production, mainly due to the development and widespread adoption of Li-ion batteries (LiBs) in electronic devices; additionally, further increments are expected since vehicle electrification is based on LiBs because of its high energy density, safety and relatively low cost [1]. Lithium could be produced from hard-rock ores and continental brines. The dependence of this metal on third countries has prompted the European Committee to develop a new EU regulatory framework for batteries, which requires ensuring the recovery by 2027of at least 50% of the lithium contained in spent batteries and its reutilization for the manufacturing of new cathode material by 2030 [2]. Current LIBs recycling is carried out at an industrial scale using pyrometallurgical and hydrometallurgical processes. However, in most cases, these methods face difficulties in recovering lithium and focus on extracting nickel and cobalt [3].
In this communication, we develop an electrochemical method - ion pumping technology- to selectively extract lithium from battery spent, based on the use of lithium-selective-electrodes, such as olivine LiFePO4 (Figure 1A), which can intercalate lithium while neglecting the insertion of other co-cations present in the solution, e.g., case of Ni, Co, and Mn for NMC based spent. The value of material selectivity towards lithium and the influence of critical extraction parameters (current density, time…) were analyzed by constant current measurements (Figure 1B) and inductively coupled plasma mass spectrometry. The results demonstrate this technology's potential for electrochemical recovery of lithium, reaching purities higher than 98% in short times - 120 minutes.
1.2-I1
Professor Louis de Smet holds a personal chair on Advanced Materials & Interfaces at Wageningen University, The Netherlands.
Materials science has proven to be valuable in tuning the selectivity in the electrochemical separation of ions. In this contribution, we specifically focus on the exploration of polymers to address the selectivity of membranes and electrodes that are employed in electrochemical deionization (EDC) and electrodialysis (ED).
Several approaches with different types of polymers will be discussed. First, the build-up of a multilayer of charged polymers (poly(allylamine hydrochloride), PAH and poly(styrene sulfonate), PSS) onto commercial available cation-exchange membranes proved to be a facile and versatile approach in switching from Mg2+-selectivity to Na+-selectivity when applied in ECD [1]. Next, in the presence of a series of mineral acids (HCl, HNO3, H2SO4, and H3PO4) a conductive polymer (polyaniline, PAni) was electrodeposited onto carbon electrodes. When employed under ECD conditions, the PAni/H2SO4 system exhibits promising behavior for tuning ion selectivity [2]. Among the tested ECD electrodes, this system achieved a notable 20% reduction in chloride adsorption while maintaining consistent sulfate adsorption. Lastly, hydrophobic polymers (poly(vinylidene fluoride), PVDF, poly(vinyl chloride), PVC, polyacrylonitrile, PAN) were blended with an ionomer to obtain anion-exchange membranes.[3,4] In electrodialysis, the nitrate over chloride selectivity trend was found to be PVC > PVDF > PAN.
While evaluation of the complete set of charged, conductive, and hydrophobic polymers shows that a ‘one‐size‐fits‐all’ approach is not readily accessible when pursuing ion selectivity, the extraction of several general principles contribute to guiding the development of advanced materials set to further tune electrochemical separation.
1.2-I2
Sylwin Pawlowski holds a PhD degree in Chemical Engineering from Universidade NOVA de Lisboa (2015). Since February 2011, he has been developing his research at the Laboratory of Membrane Processes, first as a PhD student, followed by a Postdoc position at iBET, a Postdoctoral Research Associate position at the University of Edinburgh (Scotland/UK), and currently as an Assistant Researcher at NOVA, a position earned in a very prestigious CEEC IND call.
His main area of interest is experimental and modelling work on membranes and electromembrane processes, one of the most sustainable chemical processes for power generation, water desalination and resource recovery. His research activities so far comprise recovery/recycling of lithium from brines/end-of-life Li-ion batteries, electrospinning, 3D printing, sustainable power generation by reverse electrodialysis, membrane profiling, membrane fouling, flow capacitive deionisation, brackish water desalination, digital twins, machine learning and computational fluid dynamics (CFD).
Lithium has emerged as a critical raw material because of its indispensable role in the energy transition, especially in manufacturing lithium-ion batteries for electric vehicles and portable devices. However, 95% of such batteries are discarded without recycling once they reach the end of their life. When recycled, the batteries are shredded to form a black mass, which is leached by sulfuric acid. The resulting leachate contains transition cations such as nickel, cobalt, and manganese, besides Li. Thus, it is necessary to separate these elements, which is typically achieved through successive precipitation by increasing the pH of the leachate. However, this process results in a 40-60% loss of lithium which is the last element to be recovered.
Flow Electrode Capacitive Deionization (FCDI) is a very recent electromembrane desalination technology which employs flow electrodes (carbon slurries) to remove ions from saline water. We hypothesised that replacing standard cation exchange membranes with lithium-selective ones could allow for lithium recovery from brines and spent Li-ion batteries. In this talk, the journey behind the creation of Lithium Membrane Flow Capacitive Deionization (Li-MFCDI) [1] will be disclosed.
Several key challenges were addressed to optimise the FCDI/Li-MFCDI performance regarding energy efficiency and the possibility of scale-up. Polymeric lithium-selective membranes were developed [2] to overcome the limitations of ceramic membranes, which are brittle and expensive, limiting their scalability. Another challenge in FCDI and Li-MFCDI is to maintain the uninterrupted flow of carbon slurry electrodes while preventing channel blockage. In this context, the design of flow electrode channels was investigated experimentally and by computational fluid dynamics (CFD), considering the shear-thinning behaviour of flow electrodes [3]. Furthermore, innovations, including the utilisation of 3D-printed flow electrode gaskets as a substitute for the state-of-the-art computer numerical control (CNC) milled graphite current collectors, were explored to improve system scalability and efficiency. The research also assessed various operational modes under the same operating conditions to identify the most efficient operational mode for continuous and scalable desalination or lithium recovery. Finally, several different activated carbons were tested in the FCDI system to hunt out the best material for flow electrodes to enhance performance, scalability, and overall system efficiency.
1.2-O2
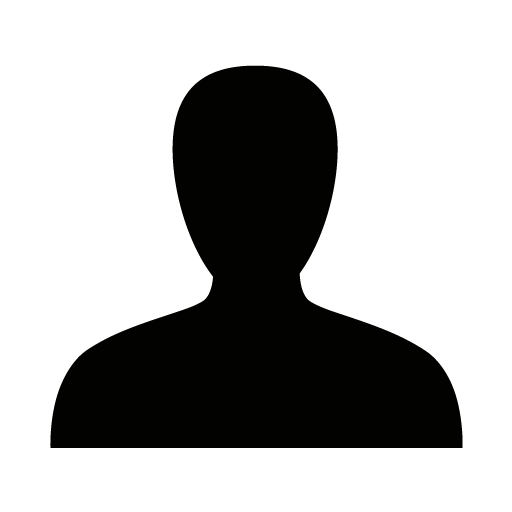
Faradaic electrochemical deionization (FDI) is emerging as a transformative technology for water treatment, addressing critical challenges in conventional capacitive deionization (CDI) such as low desalination capacity, carbon anode oxidation, and co-ion expulsion effects [1]. Utilizing faradaic electrode materials—engineered by incorporating the electrochemical principles of battery electrodes—FDI employs redox and intercalation mechanisms to selectively remove ions. This innovative approach not only achieves higher desalination capacity but also offers superior energy efficiency, particularly at lower salinity levels.
In this work, we compare two advanced battery-type electrode materials for FDI: inorganic sodium-manganese oxides (NMOs) and organic polymers. NMOs, known for their open crystal structures, allow efficient ion insertion and extraction, making them ideal for Na-ion storage in aqueous systems [2]. Meanwhile, redox-active polymers like poly[N,N′-(ethane-1,2-diyl)-1,4,5,8-naphthalenetetracarboximide] (PNDIE) offer advantages in terms of low weight, stability, safety, and sustainability [3].
Our study explores two innovative FDI approaches to enhance salt removal capacity (SRC) and cycling stability:
i) All-polymer symmetric FDI cells: Utilizing buckypaper electrodes made from PNDIE, these cells achieved a superior salt removal capacity of 155.4 mg g⁻¹. The all-polymer design enhances production, reduces energy costs and promotes sustainability.
ii) Optimization of Sodium-Manganese Oxides: This study highlights the critical role of morphology and crystal structure in the desalination performance of NMOs. The mixed-phase NMO (mp-NMO) demonstrates outstanding stability, effectively mitigating the Jahn-Teller effect—a common issue in manganese oxides that can lead to stability challenges depending on the crystalline phase.
Both approaches employ rocking-chair flow cell configurations for continuous desalination, showcasing the potential of these novel electrode materials and designs. The findings underscore their promise for efficient, sustainable brackish water desalination, offering significant contributions to global water scarcity solutions.
1.2-O3
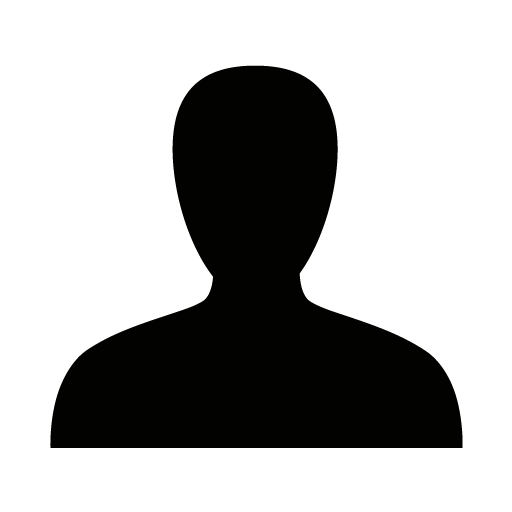
In proton exchange membrane water electrolyzers (PEMWE), the oxygen evolution reaction (OER) is considered the limiting process in water splitting. To date, iridium has been recognized as having the best OER performance in acidic conditions when regarding both, activity and stability. This instigated study of various Ir-based materials using different techniques, but some questions about the mechanisms are still debated [1]. Interesting insights into the redox Ir(III)/Ir(IV) reaction and the OER process can also be gained via Raman spectroscopy [2,3], and we apply this technique in our work using an ex situ [4] and in situ approach.
Since Ir is a scarce metal, its amount in electrocatalysis is reduced by the preparation of dispersed Ir NPs on different supports. When Raman spectroscopy of iridium has been reported in the literature, it has been recorded on metal foil [2], electrochemically deposited IrOx film on GCE [3] or drop-casted samples on GCE [4], i.e. more bulk samples. The question arises whether it is possible to perform Raman measurements directly on supported Ir NPs. We made these measurements ex situ and showed that the formation of iridium oxide can be detected for degraded states. However, the relatively low loading of Ir NPs causes the low intensity of the iridium oxide bands (Eg, B2g and A1g vibrations). The two supports that are used in this investigation were carbon and TiO2 (i.e. P25).
Consequently, we continued to investigate the performance of two commercial Ir-based compounds, Ir nanoparticles (Ir NPs) and rutile IrO2, and the measurements were made on unsupported samples. Both compounds were drop-casted from suspensions on glassy carbon electrode (GCE) and activated in a 0.1 M HClO4 electrolyte. The Raman spectrum of GCE/IrO2 consists of three active modes at 557 cm-1 (Eg), 728 cm-1 (B2g) and 746 cm-1 (A1g), while the fourth low-intensity B1g mode at 145 cm-1 [2-4] could not be identified. In the GCE/Ir NPs sample, the Eg mode appears at 552 cm-1, while the B2g and A1g modes appear as an overlapping band at 724 cm-1. Such spectrum indicates that the Ir NPs oxidize in air and the amorphous Ir-oxide forms on the surface. During the in situ Raman measurements of the GCE/Ir NPs a composed, broad band feature evolves. The previous works were carried out in different electrolytes, potential ranges and conditions [2,3] which makes it difficult to compare the results. We consequently decided to make a systematic study in three different potential ranges: 0.05 to 1.45 VRHE , 0.05 to 1.6 VRHE, and 1.1 to 1.6 VRHE and in the 0.1 M HClO4 electrolyte, which has not been used for in situ Raman spectroelectrochemical measurements before. The evolved broad bands are explored after initial, soaked and activated states. In addition, the possible perchlorate adsorption [4] at the electrode is considered.
1.3-I1
Dr. William Tarpeh is an assistant professor of chemical engineering at Stanford University. The Tarpeh Lab uses catalysis and separations to advance wastewater refining, which generates tunable portfolios of products from water pollutants. In addition to improving mechanistic understanding of novel materials and processes, the group also advances wastewater treatment in resource-constrained communities to improve access to water, fertilizers, and chemical commodities. Will completed his B.S. in chemical engineering at Stanford, his M.S. and Ph.D. in environmental engineering at UC Berkeley, and postdoctoral training at the University of Michigan. His recent awards include the NSF CAREER Award, Dreyfus Teacher-Scholar Award, AIChE 35 Under 35 and the Environmental Division Early Career Award, and the Electrochemical Society Young Investigator Fellowship.
Over the past century, humans have altered the global nitrogen cycle so drastically that managing nitrogen has emerged as a grand engineering challenge and urgent need. The emissions-intensive Haber-Bosch process for industrial fertilizer production, which converts nitrogen gas into ammonia, outpaces wastewater nitrogen removal due to fertilizer runoff and 80% of wastewater being discharged without treatment. Refining nitrate and ammonia into valuable products through reactive separations, which integrate catalysis and separations, is a useful approach for addressing both water pollution and chemical manufacturing. For example, selective membranes and adsorbents can be leveraged to control catalytic performance by tuning microenvironments near catalyst active sites. This seminar will focus on recent work designing metal electrocatalysts for selective reduction of nitrate to ammonia, along with separation of high-purity ammonia from real wastewaters. Specifically, we focus on understanding the reaction microevenionment of titanium and cobalt in multiple catalyst architectures while leveraging a systematic study of electrolyte composition on catalyst activity, selectivity, and stability. We complement these efforts with reactive separation devices that leverage electrochemical potential to drive nitrate and ammonia transport, which advances the vision of wastewater refining: producing a tunable portfolio of products from real wastewaters.
1.3-I2
The alkaline oxygen evolution reaction (OER) is crucial for green hydrogen production via water electrolysis. However, its industrial implementation at high current densities remains limited due to the scalability, overpotential, and stability challenges of current commercial electrocatalysts. Layered hydroxides (LH), particularly those based on abundant transition metals, are emerging as promising alternatives owing to their remarkable electrochemical properties.
In this talk, we will present the latest advances from the 2D-Chem research group (www.icmol.es/2dchem) in the synthesis and characterization of novel two-dimensional (2D) LH materials. Specifically, we have developed an industrially scalable, room-temperature, atmospheric-pressure homogeneous alkalinization synthetic pathway to produce optimized NiFe layered double hydroxide (NiFe-LDH). By leveraging the nucleophilic attack of chloride on an epoxide ring, we have achieved a low-dimensional, highly defective NiFe-LDH exhibiting pronounced cation clustering and excellent electrochemical performance. Spectroscopic studies, including in-operando XANES, EXAFS, SAXS or Raman combined with ab-initio calculations reveal the critical role of Fe clustering in lowering the energy pathway for improved catalytic activity.
Furthermore, we have extended this synthetic route to other compositions that will demonstrate the versatility of these materials beyond green hydrogen production. Indeed, in-situ XAS and PXRD studies provide further insights into the behavior of these layered materials during operation, allowing their use as precursors for metallic nanocomposites with applications in energy storage. Finally, optimized LDH and hybrid LDH-nanocarbon electrocatalysts with tailor made compositions can also play a pivotal role in alkaline electrochemical water treatment for the remediation of contaminated water systems, offering a sustainable approach for pollutant degradation and removal.
1.3-O1
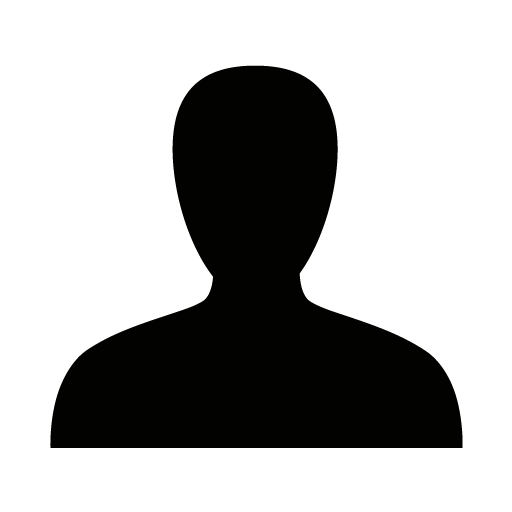
In recent years, the utilization of renewable energy, particularly solar power, has been accelerating globally from the perspectives of both the global energy problem and the decarbonization of society. To expand the use of renewable energy, it is necessary to store energy, and hydrogen is particularly suitable for large-scale, medium- to long-term storage. In a world aiming for carbon neutrality, hydrogen is a clean energy that can be produced by electrochemically splitting water, and water electrolysis cells that enable this are being researched and developed for practical use, with simulator development being part of this effort.
However, current simulator development involves detailed simulation environments at the particle level that represent reaction mechanisms and electrochemical phenomena. Using these as a system places a heavy burden on the calculations, making them difficult to use. When considering device requirements, there are currently no simulators available that can represent current-voltage characteristics in a simple but reasonably detailed manner.
Therefore, we constructed a physical model of a PEM water electrolysis cell using MATLAB/Simulink, which takes into account the frequency characteristics and capacitance characteristics, and can also represent the current-voltage characteristics.
In this study, with the cooperation of RIKEN, we measured actual data from water electrolysis cells used in distributed hydrogen systems. To express both static and dynamic characteristics, we conducted I-V measurements and FRA measurements, respectively, and performed parameter fitting using the measurement results.
As a result, the current-voltage characteristics within the compatible range could be expressed with an accuracy of over 95%, and the electrical transient characteristics of the water electrolysis cell, such as inrush current, could be expressed qualitatively.
Furthermore, by accurately expressing the behavior of water electrolysis cells while ensuring sufficient simulation speed as a simulation environment for the system, it became possible to verify the necessary device requirements. We expect this to be utilized in system studies using water electrolysis cells and in device prototyping using electrochemical expressions.
1.3-O2
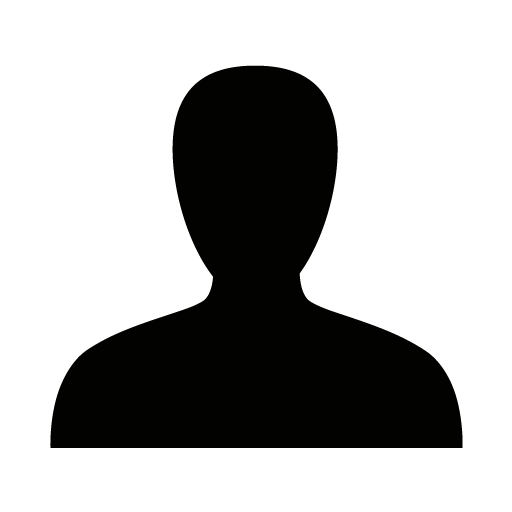
The activation of O2 through electrochemical reduction (ORR, oxygen reduction reaction) has shown promising results as alternative energy conversion technologies that can produce added-value chemicals from simple and abundant feedstocks. However, despite extensive efforts to develop catalytic materials with high reactivity and high selectivity, one can currently observe the lack of demonstrative performance for viable industrial applications. The deliberate surface modification of catalyst has been recently recognized as a powerful approach to design efficient and durable electrocatalysts [1]. It then becomes essential to obtain a good control over the spatial distribution of the chemical functions over the nanoobject surfaces. Recently, excellent catalytic properties towards ORR have been obtained in alkaline media of gold [2], silver [3], and platinum [4] nanoparticles when modified through the reductive grafting of rigid macrocycle calix[4]arene-tetradiazonium salts [5]. However, many fundamental questions, with important operational implications, remain open about these calixarene-modified surfaces. In particular, the conformation of the calixarene on the surface, and the structural, thermodynamic and electronic description of the interface. In addition, the C-Au bond has been poorly investigated in comparison to the S-Au bond.
Here, using spectroscopic studies coupled with computational modeling performed with density functional based tight-binding (DFTB) approaches, we investigate the interaction between calix[4]arene macrocycles and gold and platinum nanoparticles. After exploring the nature of the bond between the macrocycle and the gold surface thanks to a good agreement between measured and calculated Raman spectra, we describe the effect of calix[4]arenes on nanoparticles electrocatalytic properties [6].
2.1-I1
Hydrogen is a clean energy source and an important candidate to replace fossil fuels in the near future (energy transition). However, traditional hydrogen production is achieved through processes that are expensive and non-sustainable require high amounts of energy, such as carbon gasification or steam methane reforming. Waste waters are considered a cheap and abundant energy source, as they contain high amounts of organic compounds. Therefore, the hydrogen production from waste water can be a useful solution in order to reduce the energy costs associated with traditional processes.
REGENERA project (CDTI- Misiones 2019) investigates the delocalized storage of energy from renewable energies in the form of green fuels, hydrogen and methane. This communication presents the BES-BioH2 laboratory concept, a “Power-to-Gas” technology that aims to generate hydrogen (H2) by using a microbial electrochemical cell. The objective is to achieve an integrated system at laboratory scale which allows the simultaneous waste water treatment, hydrogen production and biogas upgrading (achieving a purity >95% of CH4).
Bioelectrochemical systems (BES) combine electrochemistry with the metabolism of electroactive microorganisms for energy production. In Microbial Electrolysis Cells (MECs), electroactive bacteria grow building a biofilm on the surface of a conductive anode, which acts as electron acceptor. The electroactive microorganisms oxidize organic matter to CO2 under anaerobic conditions and the electrons obtained in the process are transferred from the anode to the cathode through an electrical circuit [1]. The cathodic reaction is the H2 formation through H2O reduction under alkaline conditions. The reduction of water to hydrogen is a non-spontaneous process, so the application of an external potential is required.
This study presents the experimental results using different real waste waters (urban and industrial) and discusses on the potential of various organic substrates for hydrogen production. Thus, the energy cost for hydrogen production (kWh/kg H2), the chemical organic demand removal rate (g/m3 day) and the hydrogen production rate (m3 hydrogen/m3 reactor) are presented in order to compare the present system with conventional system for urban waste water treatment. Finally, the study suggests main limitations and opportunities for the implementation at real scale, as the final objective of REGENERA project is the development of a prototype of 500 L capacity by the end of year 2024, with capacity to produce 1-10 Nm3H2/day.
2.1-I2
Conductive carbon materials gather a matchless combination of exceptional properties, highlighting their availability, relatively low-cost, lightness, sufficient stability and enormous versatility to be prepared in different sizes, shapes, conformations, porous textures and surface compositions, making them excellent candidates to be used as electrodes in various electrochemical technologies. Among them, there stand out the technologies applied in the field of water treatment, like those based on pollutants electrosorption, electrooxidation and biodegradation (in the so-called microbial electrochemical technologies (METs)). In addition, these technologies show great interest in addressing the challenges of water-energy nexus. In this context, the design of carbon properties is of paramount importance for the feasibility and optimization of these technologies. On the other hand, the availability, cost and environmental impact of these materials are key factors for their development and full-scale application.
This contribution revises the carbon properties that determine their performance in electrochemical water treatment applications. Particularly, the influence of microstructure, porosity and surface chemistry on the electrochemical properties of carbons (conductivity, stability, electrochemical double layer, electron transfer, etc.) is analyzed. Furthermore, advances in strategies and tools to control and optimize these properties are discussed. Finally, recent findings on carbon properties stimulating microbial extracellular electron transfer for METs are summarized. In this respect, recent studies demonstrate that certain oxygen surface groups can promote anchorage and/or electron transfer with electroactive bacteria; whereas nanoscale (bacteria-inaccessible) porosity remarkably enhances the microbially derived electrical current. Among different carbon materials, electroactive biochar is proposed as a good candidate for large-scale environmental applications of METs.
2.1-O1
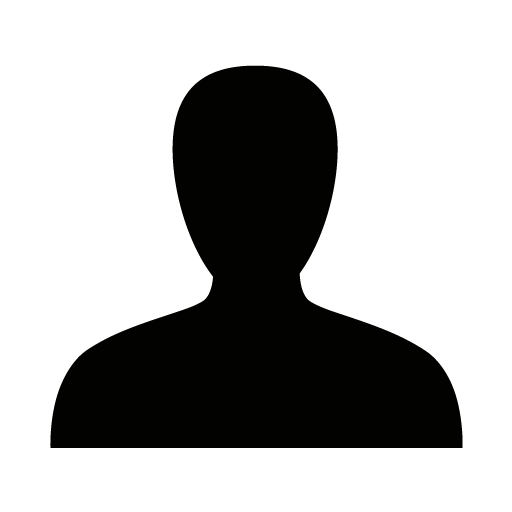
Among the electrochemical advanced oxidation processes (EAOPs) for wastewater treatment, which exhibit high effectiveness combined with green deployment, some of them primarily rely on the efficiency of anode materials in generating potent oxidants like hydroxyl radicals (•OH). Hence, the development of novel, cost-effective nickel-manganese-based anodes has been investigated in this work, aiming to revolutionize the electro-oxidation of organic pollutants. Key to this endeavor is the use of 3D porous conductive substrates, akin to those employed in redox-flow batteries and water electrolyzers. These substrates are crucial for maximizing the electrode-electrolyte interactions and facilitating efficient flow-through designs, thereby significantly boosting the EAOPs performance. The synthesis, characterization, and optimization of these Ni-Mn-based electrodes, including their physicochemical structure and electrochemical properties, have been studied. It has been found that by employing Ni-Mn-based materials as the active material and utilizing the high surface area of 3D substrates such as nickel foam and graphite felt, our electrodes achieved a remarkable 100% removal of phenol, coupled with an 80% reduction in chemical oxygen demand (COD), thus marking a significant advancement over traditional anodes. Comparative analyses with boron-doped diamond (BDD) and dimensionally stable anode (DSA) highlight the superior activity and efficiency of our anodes. Further, a detailed mechanistic study was undertaken to elucidate the electrochemical pathways and interactions. This investigation reveals an enhanced generation of hydroxyl radicals and other oxidizing species on the anode surface, justifying the observed degradation efficiency. The implications of these findings are substantial in the field of wastewater treatment. Developing our Ni-Mn oxides not only sets a new benchmark in pollutant degradation efficiency but also offers valuable insights into the electrochemical mechanisms underpinning EAOPs.
2.1-O2
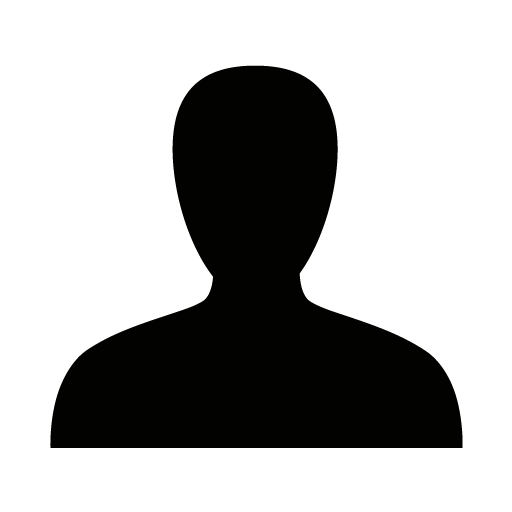
The water crisis is a major concern at global scale, which underscores the need for alternative freshwater resources. In this context, urban wastewater is one of the main targets to feed regenerated water for many applications, but it faces challenges from persistent pharmaceuticals resistant to conventional treatments. Advanced methods like electrochemical advanced oxidation processes (EAOPs), particularly the electro-Fenton (EF) process, offer a highly promising performance [1]. However, limitations such as narrow pH range and low H2O2 yields must be addressed by developing new electrocatalysts and heterogeneous catalysts.
This work addresses these issues through two innovations: (1) Electrocatalysts for highly selective two-electron oxygen reduction reaction (2e– ORR) to generate H2O2 in situ, and (2) advanced heterogeneous catalysts with enhanced H2O2 activation efficiency.
In EF, 3.0 to 15.0 mM H2O2 is sufficient to purify wastewater [2], making the electrochemical 2e– ORR with a gas-diffusion electrode (GDE) an attractive alternative to the industrialized anthraquinone process to synthesize H2O2. Tin (Sn) exhibits strong O2 adsorption under alkaline conditions [3]; accordingly, Sn-doped carbon materials are demonstrated to have outstanding H2O2 selectivity (98%) and high H2O2 production efficiency at near-neutral pH, with an electron transfer number of 2.04. Nitrogen-doped carbons, optimized for pyrrolic nitrogen content, delivered superior H2O2 yields (reaching 18 mg h–1 cm–2) compared to commercial GDEs.
For H2O2 activation, Cu/NC and FeCu/NC catalysts derived from MOFs exhibited great performance. Cu/NC enabled the effective pollutant mineralization at pH 6–8, being superior to the EF process with soluble Fe2+ at pH 3. The core-shell structure of FeCu/NC minimized metal leaching and extended catalyst lifespan. This brought about an accelerated Fe(II) regeneration, achieving 100% removal of lisinopril within 75 min.
This research shows significant progress in the development of sustainable EF systems, integrating the efficient electrogeneration and activation of H2O2, which allows addressing the problem of pharmaceutical pollutants in wastewater.
2.2-I1
Emmanuel Mousset has completed his Erasmus PhD in Environmental Science and Technology in 2013 delivered by University of Paris-Est (France), University of Cassino (Italy) and UNESCO-IHE (The Netherlands), followed by post-doc positions in National University of Singapore (NUS) and Helmholtz Center for Environmental Research (Leipzig, Germany). He is currently Senior Researcher at the French National Scientific Research Center (CNRS) since 2016. His main scientific and technological contributions are in electrochemical engineering (e.g., reactor design and engineering, sizing), (electro)-chemical kinetics and reactivity, as well as electrode/electrolyte interfaces studies. He has been awarded with the young investigator price in 2022 by the French Electrochemical Group from the French Chemical Society, the Carl Wagner Medal of Excellence in Electrochemical Engineering in 2020 from the Electrochemical engineering group of the European Federation of Chemical Engineering (EFCE), as well as the Green Electrochemistry Prize in 2018 from the International Society of Electrochemistry (ISE) and Elsevier.
Water issues represents one of the biggest challenges of the 21st century. The United Nations has addressed Sustainable Development Goal (SDG) to answers the water crisis. The increase of water demand in the different sectors (agriculture, industry and household), and the water stress that is globally rising in the meantime, are responsible for this critical issue. To face it, the water reuse approach is more and more considered. However, before reusing wastewater there is the need to completely remove emerging pollutants (e.g., pharmaceuticals, pesticides, personal care products) since they are not eliminated by the bioprocesses typically applied in plants. Still, wastewater contains valuable chemicals (e.g., phosphate, magnesium) that could be recovered in the meantime. Electrochemical systems can tune the removal and recovery steps by playing on the applied potential/current, in contrast with chemical processes. They also offer the advantages of operating several unit operations (separation and conversion technologies) without the need for chemicals addition and within the same reactor design. Moreover, vector of energy could be electrogenerated (e.g., hydrogen (H2)). This presentation will focus on case studies implementing electro-precipitation, electro-sorption and electro-reduction/-oxidation for resource recovery in wastewater under microfluidic conditions.
2.2-I2
Dr. Sonia Lanzalaco is a Chemical Engineer and Ph.D. by the Università degli Studi di Palermo (UniPA, Italy). Her research interests are focused on materials science and polymer technology, having wide expertise in materials synthesis and characterization, as well as in supercritical and electrochemical methods. In 2016, she obtained her Ph.D. Degree in Chemical and Materials Engineering (co-funded by LIMA Corporate) at UniPA. During her Ph.D., she spent a 4-month period as Visiting Researcher at Carnegie Mellon University in Pittsburgh (USA) under the supervision of world-renowned Prof. Dr. Krzysztof Matyjaszewski (ATRP polymerization). She has collaborated with Prof. Giuseppe Storti (ETH-Zurich), Prof. Armando Gennaro (Università di Padova, Italy) and Prof. Ignasi Sirés (Universitat de Barcelona, UB, Spain). In 2018, she obtained a prestigious individual fellowship under the Marie Sklodowska-Curie Actions (MSCA) call funded by the H2020 program. As MSCA postdoctoral researcher at the Innovation in Materials and Molecular Engineering (IMEM) group of the Universitat Politècnica de Catalunya (UPC), she led the project entitled 4D-POLYpropylene meshes as SENsitive motion SEnsors (4D-POLYSENSE). She worked in close collaboration with the global company BBraun Surgical S.A. (6-month secondment), developing innovative and smart polymer-based materials for hernia repair. Currently, she is a Lecturer and Senior Researcher at IMEM group (Prof. C.Alemán) and actively participates in several research projects and student supervision and training. Her current research agenda includes the preparation of: (i) smart platforms for electrochemical sensor devices, (ii) new in vitro detection and sterilization methods for bioengineering applications, and (iii) biomass-based polymers for health and environmental applications.
Feeding long-term sustainability to our planet is becoming a major must, given the urgent need to preserve our natural resources, reduce pollution and protect the natural ecosystems. Sustainable development holds on three pillars, namely environmental sustainability and protection, economic viability, and social equity. Among them, the former receives great attention and it is build up on reducing carbon emissions and footprint, packaging waste, water usage, and other negative environmental impacts. New paradigm towards green chemistry, sustainability, and circular economy in the chemical sciences must be developed, in order to better employ, reuse, and recycle the materials employed in every aspect of modern life. Following this approach, electrochemical reactions have found technological applications in various fields, including electrochemical synthesis, energy storage, and environmental remediation [1]. Sustainability and electrochemistry are therefore closely related. In this talk, the utilization of electrochemical tools together with bio-based or sustainable materials is presented.
First, the exploration of new synthetic routes to reduce the toxicity of residual monomer and other chemicals employed (initiators, surfactants) during the fabrication of polymer hydrogels, one of the most promising groups of biomaterials, is required. With a similar approach to the previously developed "green" and clean production of polymer nanogels, largely used in biomedicine, based on the recourse to high energy irradiation, electrochemical advanced oxidation technologies were used to crosslink hydrogels of poly(vinylpyrrolidone)(PVP) by means of electrogenerated hydroxyl radicals. This facile electrosynthesis route showed that the kind of radicals strongly drives the transformation of the architecture of linear, inert polymer chains into a functionalized nanogel (with -COOH and succinimide groups), more suitable for further conjugation [2-4].
Second, the conversion of bio-based polymers such as chitosan and agarose, into eco-friendly carbonaceous electrodes is described, as a suitable choice for promoting sustainability due to their low cost and high activity/selectivity [5-6]. The use of mesoporous carbon supports both reduce the amount of noble metals employed as electrocatalysts and enhance the accessibility of reactants to the active sites. On the one hand, excellent electrocatalytic performance of N-doped chitosan-derived carbons and large surface area agarose-derived carbons are responsible of the high efficiencies achieved (above 95%), allowing the fast destruction of pharmaceutical residues in electro-Fenton treatment. On the other hand, chitosan-derived mesoporous carbons served as optimal supports for PtCu electrocatalysts, evidencing an increased activity of both the four-electron ORR and the methanol oxidation reaction as compared to commercial supported Pt and PtCu catalysts, which is attributed to the good balance achieved between micro/mesoporosity.
Finally, one of the most appealing and recent trends in the application of biopolymers in electrochemical water treatment is reported. The co-generation of freshwater and sustainable energy in a closed loop where the solar energy is used not only for water purification treatment with porous materials like hydrogels, but also for thermoelectric power generation, by means of material transpiration and diffusion processes. The photothermal electricity production is promoted by hydrogels based on biopolymers such as alginate (ALG) and thermosensistive materials like poly(N-isopropylacrylamide) (PNIPAAm). ALG-PNIPAAm bio-hydrogel, modified with conducting polymer (CP), as thermal absorber component, was used to obtain freshwater from seawater desalination under sunlight. Higher evaporation rates (> 4 kg/h*m2) have been observed in the presence of lineal CP, if compared with nanoparticles of CP [7-8]. Impedance measurements elucidate the ion diffusion dynamics within the hydrogel, directly correlating this behavior to enhanced power generation; these results revealed that the presence of hydrophilic groups (─OH, ─SO3H), present in the CP backbone, promotes the capillary flow of the electrolyte during the sunlight irradiation. The doped CP molecules facilitate a fast ion transport thanks to a good balance between the material hydrophilicity and the interconnected pores.
2.2-I3
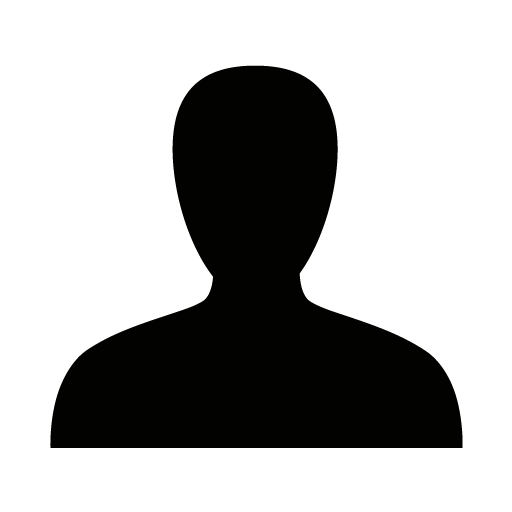
Electrocatalysis is increasingly important in water treatment for its efficiency and eco-friendliness, and materials play important role in performance. In this work, we synthesized an efficient single-atom Co-N/C catalyst for electrocatalytic dehalogenation, which provided more active sites and a faster charge transfer rate. Co-N/C effectively removed florfenicol (FLO) over a broad pH range, with rate constants that were 3.5 and 2.1 times higher than those of N/C and commercial Pd/C, respectively. The defluorination and dechlorination efficiencies were 67.6 and 95.6%, respectively, with extremely low Co leaching (6 μg L-1) and low energy consumption (22.7 kWh kg-1). H* and direct electron transfer were the primary causes of dehalogenation. The Co-N/C was minimally affected by pH, co-existing ions, and water quality, maintaining a high removal rate (>90%) after ten cycles [1]. To enhance H* production, the phosphorus-doped cobalt nitrogen carbon catalyst (Co-NP/C) was prepared for electrocatalytic dechlorination, which had high catalytic activity in a wide pH range (3-11). The introduction of phosphorus was found enhanced the electron density of cobalt and regulated the electron transfer.
We further developed the heterogeneous electro-Fenton process based on dual-functional cathodes. The catalyst composed of nitrogen-doped carbon nanotubes encapsulating zero-valent iron (Fe@N-C) was synthesized, which demonstrated superior degradation of sulfamethazine (SMT) under mildly alkaline conditions. The primary reactive species generated by Fe@N-C were H* and singlet oxygen (1O2), with hydroxyl radicals (∙OH) playing a supportive role [2]. Additionally, the catalyst with boron and nitrogen co-doped carbon nanotubes encapsulating zero-valent iron (Fe@BN-C) was fabricated, which significantly increased the selectivity for H2O2 to 94%, and H2O2 was directionally converted to 1O2 via surface ∙OH. Theoretical calculations confirmed the confinement effect of Fe0 overcame the rate-limiting step for H2O2 formation, achieving high efficiency and selectivity for 1O2 transformation[3].
Traditional free radicals-dominated electrochemical advanced oxidation processes (EAOPs) and sulfate radical-based advanced oxidation processes (SR-AOPs) are limited by pH dependence and weak reusability, respectively. To address these shortcomings, electro-enhanced activation of peroxymonosulfate (PMS) was proposed. Firstly, a novel perovskite-Ti4O7 composite anode activating PMS (E-PTi-PMS) system achieved an ultra-efficient removal rate (k = 0.467 min-1) of carbamazepine (CBZ). The electric field expedited the decomposition and utilization of PMS, promoting the generation of radicals and expanding the formation pathway of 1O2. This system presented superiorities over wide pH (3-10) and less dosage of PMS (1 mM), expanding the pH adaptability and reducing the cost of EAOPs [4].
1.1-I1
Since 2019, Yana Vaynzof holds the Chair for Emerging Electronic Technologies at the Technical University of Dresden. Prior to that (2014-2019), she was a juniorprofessor in the Department of Physics and Astronomy, Heidelberg University (Germany). She received a B.Sc degree (summa cum laude) in electrical engineering from the Technion - Israel Institute of Technology (Israel) in 2006, and a M.Sc. degree in electrical engineering from Princeton University, (USA) in 2008. She pursued a Ph.D. degree in physics under the supervision of Prof. Sir. Richard Friend at the Optoelectronics Group, Cavendish Laboratory, University of Cambridge (UK), and investigated the development of hybrid polymer solar cells and the improvement of their efficiency and stability. Upon completing her PhD in 2011, she joined the Microelectronics group at the University of Cambridge as a Postdoctoral Research Associate focusing on the research of surfaces and interfaces in organic and hybrid optoelectronics. Yana Vaynzof was the recipient of a number of fellowships and awards, including the ERC Starting Grant, Gordon Y. Wu Fellowship, Henry Kressel Fellowship, Fulbright-Cottrell Award and the Walter Kalkhof-Rose Memorial Prize.
Introducing chiral organic spacers in low-dimensional metal-halide perovskites triggers chiroptical activity, making these materials of great interest for spintronic applications. To enable such applications, it is necessary to develop a deep understanding of the structure formation of chiral two-dimensional (2D) perovskites and its impact on their optical properties. While much attention has been dedicated to developing processing routes to control the properties of achiral 2D perovskites, the use of chiral cations introduces higher steric hindrance, thus significantly impacting structure formation. I will discuss how changing the processing conditions impacts the phase purity, microstructure, and chiroptical properties of chiral 2D perovskites. For example, in solution-processed chiral 2D perovskites, the choice of solvent or the use of additives enables control over the structure and microstructure of the deposited thin films. Alternatively, I will show that chiral 2D perovskites can be processed by thermal evaporation, opening new pathways to large-scale deposition and microstructuring.
1.1-I2
Dr. Beatriz Martín-García received her Ph.D. in Chemical Physics (Cum Laude) from University of Salamanca (Spain) in 2013. Then, she joined Istituto Italiano di Tecnologia (Italy) under the Graphene Flagship project working during almost 6 years on the modulation of optoelectronic properties of different materials (nanocrystals, 2D materials and hybrid metal-halide perovskites) by chemical-design and surface-functionalization strategies for their integration in solar cells, photodetectors and memories. She is currently an Ikerbasque researcher and Ramón y Cajal fellow at CIC nanoGUNE BRTA, leading a research line developing tailor-made low-dimensional materials and studying them by Raman and photoluminescence spectroscopy techniques to drive the selection of desired properties for their integration in optoelectronic and spintronic devices.
The chemical and structural flexibility of layered (2D) hybrid organic–inorganic metal-halide perovskites (HOIPs) has been proposed as an ideal platform for the synthesis of novel circularly polarized light emitters through the incorporation of chiral organic molecules, showing potential application in optoelectronics and spintronics.[1,2] Furthermore, the weak forces between the organic layers in the case of Ruddlesden-Popper 2D HOIPs, allow mechanical exfoliation of bulk crystals to obtain flakes[3] making possible their integration in nanodevices. Most studies to date have focused on bulk compounds, specifically on the unstable and toxic Pb-based HOIPs,[1,2] although Mn-based HOIPs apart from lower toxicity can show not only chiroptical but also magnetic properties[4]. In this work, we report the chiroptical properties of R- and S-β-methylphenethylammonium Mn chloride HOIPs, which exhibit antiferromagnetic order,[5] in both bulk and mechanically exfoliated flakes. In these compounds, we observe the red photoluminescence (PL) emission originating from the octahedrally coordinated Mn2+, with a PL redshift as they transition from bulk to flake form. Circular dichroism (CD) and circularly polarized luminescence (CPL) mirrored signals confirm the chirality transfer from the organic cations to the inorganic lattice in bulk materials, presenting glum values (0.01) among the highest reported for chiral hybrid Mn halides. This chirality is preserved in the exfoliated flakes, reaching degrees of circularly polarized PL (P) of up to 17% at 80K, which systematically decrease with increasing temperature as previously observed in 2D Pb-based HOIPs[6]. Additionally, angle-resolved PL measurements show that the PL emission and P are isotropic. Therefore, our results demonstrate that these 2D Mn-based HOIPs are highly valuable, as they can compete with their Pb analogs and offer additional functionalities for spin-optoelectronic applications, thanks to the magnetic behavior associated with Mn2+.[7]
1.1-I3
Hybrid organic–inorganic perovskites have emerged as exceptional materials for optoelectronic and energy conversion devices[1]. Recently, chiral hybrid perovskites, which incorporate chiral organic ligands into the inorganic framework, have attracted increasing attention as promising chiroptoelectronic systems with potential applications in optoelectronics, spintronics, and beyond [2]. The chirality and associated chiroptical responses in these materials are attributed to a chiral bias originating from the chiral organic ligands, which propagates through the inorganic framework, influencing the geometry of the entire hybrid perovskite structure [3].
Modern multiscale modeling and simulation techniques have now reached unprecedented levels of accuracy, enabling the efficient design of chiral materials and the precise optimization of their chiroptical properties. In this discussion, I will present simulation workflows developed over the years to predict the circular dichroism (CD) and circularly polarized luminescence (CPL) of soft [5]and hybrid materials [6].
Enhanced sampling simulations, particularly through parallel bias metadynamics, in conjunction with ab-initio molecular dynamics (AIMD) based on density functional theory (DFT) methods and their time-dependent extensions, were employed to investigate the structure, dynamics, and chiroptical spectra, with a focus on CD and CPL.
This simulation strategy enables the prediction of how non-covalent interactions in excited states drive the generation of CPL spectra and the associated dissymmetry factors.
References
[1] Grancini, Nazeeruddin. Nat. Rev. Mater. 4, 4-22.
[2] Pietropaolo, Mattoni, Pica, Fortino, Schifino, Grancini. Chem 8: 2022, 1231.
[3] Long, Sabatini, Saidaminov, Lakhwani, Rasmita, Liu, Sargent, Gao. Nat. Rev. Mater. 2020 5 423.
[4] Albano, Pescitelli, Di Bari. Chem. Rev. 120: 2020, 10145.
[5] Wu, Pietropaolo, Fortino, Shimoda, Maeda, Nishimura, Bando, Naga, Nakano. Angew. Chem. Int. Ed. 61: 2022, e202210556.
[6] Fortino, Mattoni, Pietropaolo. J. Mater. Chem. C 11: 2023, 9135.
1.2-O1
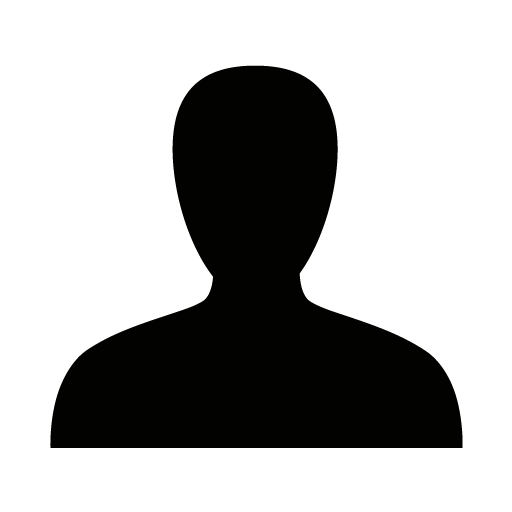
Over the last ten years, the role of hybrid metal halide perovskites has received significant attention as suitable materials for various electronic applications. Indeed, their outstanding optoelectronic properties such as high-power conversion efficiency, tunable bandgap, and high absorption coefficient, make them suited for several applications in different devices as photovoltaic cells, photodetectors, light emitting diodes, and sensors[2]. Starting from the hybrid organic-inorganic perovskites (HOIPs), the introduction of a chiral molecule as organic cation leads to the breaking of the spatial inversion symmetry, allowing new possible designs based on the combination of polarity and chirality[1,3]. In the scientific scene, this opened plenty of novel applications provided by outstanding chiroptical properties, such as circular dichroism, circular polarized emission, chiral induced spin selectivity and so on. To extend the actual knowledge of these chiral systems, it is important to investigate those parameters which have a major impact on the chirality transfer mechanism, with the final aim to unveil it. From a material chemistry point of view this involve an important work on materials’ structure, involving several modulations/substitutions on the latter. More specifically, in this contribution we will present the results of the role of the organic cation, showing the modulation of the optoelectronic properties engineering unconventionally chiral cation. Firstly, we decide to move away from the commercial chiral cation and to synthesize homologous series that can help unveil the role of the chemical nature of the cation on the chiroptical properties. We obtained a new phase (R-/S-AMOL)PbI3 and the correspondence with the Sn[4]. The work provides a comparison not only in terms of structural features and chiroptical properties but also in terms of computational modelling, which helps us to deeply understating the role of organic cation and the difference in terms of efficiency moving from a Pb-based perovskites to a Pb-free one. Final aim of this work is to unveil the impact of chemical degrees of freedom on the chirality transfer between the organic cation and the inorganic framework to provide tuning strategies for materials engineering.
1.2-O2
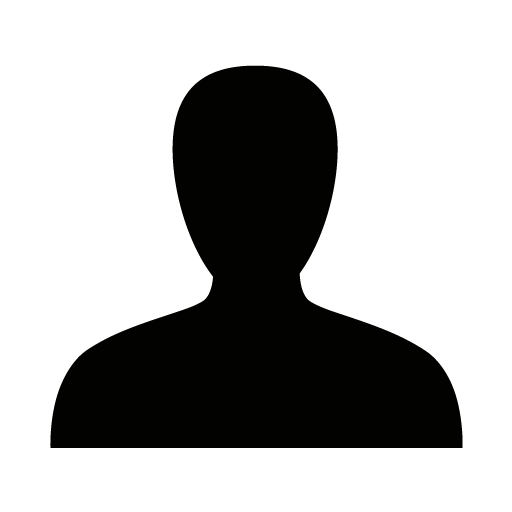
Metal Halide Perovskites (MHPs) and their derivatives are the subject of intense research due to their remarkable optoelectronic properties and tunable bandgap [1]. Major applications include photovoltaic cells for three-dimensional perovskites, as well as photodetectors, photodiodes, and lasers for other perovskite-based materials. A significant modification that can be made to organic-inorganic metal halides is the introduction of chiral organic cations, leading to the emergence of chiroptical properties and the Rashba-Edelstein effect [2]. The latter is particularly interesting for spin-related phenomena such as chirality-induced spin selectivity (CISS).To date, most chiral metal halides contain lead, a toxic element subject to strict regulations. Therefore, the exploration of lead-free materials is crucial. In this study, we report the synthesis of bismuth (III)- and antimony (III)-containing chiral iodides, including R/S-1-(4-Chloro)-Phenylethylammonium (abbreviated as Cl-PEA) as the organic chiral cation. Single crystals with the stoichiometry Cl-PEA₄M₂I₁₀ (where M represents Bi or Sb) have been prepared for both enantiomers and the racemic compound, and their structures have been resolved via single-crystal XRD. Additionally, mixed Sb/Bi systems have been synthesized to investigate potential bandgap bowing, which has already been observed in analogous vacancy-ordered achiral perovskites [3], and already analysed via powder diffraction.The optical properties of the prepared samples have been analyzed using UV-Vis and CD spectroscopy. Finally, starting from the experimental data, computational modeling has been employed to optimize the crystal structures, determine the electronic band structure, and evaluate the presence and extent of Rashba splitting. The modeling has also been used to calculate the projected density of states (PDOS) and the total density of states (DOS) of our compounds. These calculations provide insights into the orbital contributions to the observed bandgap bowing and identify the contributing species.
1.2-I1
During the recent years, chiral hybrid organic-inorganic perovskites where the organic cations are the “source” of chirality, have received great attention from the physics and chemistry research community. Their functional properties enable the control of light, charge, and electron spins in the same materials. Here, we will discuss the intriguing “chirality transfer mechanism” in some newly synthesized ferromagnetic chiral hybrid inorganic perovskite along with their interplay with magnetism. Although the organic cations are chiral and polar molecules, their arrangement in the crystal structure results in a chiral non-polar space-group P212121. Moreover, we discuss a new chirality order parameter such as the electronic chirality measure (ECM) aiming at quantify the molecular cation chirality taking into account ionic and electronic degrees of freedom simultaneously. Also, the relation of ECM to physical properties of chiral hybrid perovskites will be discussed, so shedding light on the fundamental principles governing their behavior and paving the way for the development of innovative optoelectronic and spintronic devices.
1.2-I2
Shuxia Tao is a compuational materials scientist and she studies how photons, electrons and ions interact with each other and how such interactions determine the formation, function and degradation of materials. Currently, she leads the Computational Materials Physics group at the department of Applied Physics, Eindhoven University of Technology, the Netherlands.
Tao's group focuses on multiscale modelling of energy and optoelectronic materials, studying the growth of nanomaterials and developing theory of light-matter interactions. The ultimate goal is perfecting the quality of these materials and maximizing their efficiency for converting and storing energy and information. Her recent contribution to PV materials focuses on halide perovskites, where she made important contribution in the understanding of the electronic structure, the defect chemistry/physics and the nucleation and growth of halide perovskites. Recently, she also expanded the research to the interactions of perovskites with other contact materials in devices and novel optoelectronic properties, such as optical chirality and chiral induced spin selevetivity.
Chirality, the property of objects being mirror images but non-superimposable, is a fundamental characteristic found in diverse systems, from DNA helices to subatomic particles. When chiral systems interact with electron spin, they exhibit Chiral-Induced Spin Selectivity (CISS), enabling chiral molecules to act as spin filters. This remarkable phenomenon has transformative implications for spintronics, drug design, and understanding the origin of biological chirality. By employing advanced semiconductors like perovskites, we explore CISS under controlled conditions. These chiral perovskites not only enhance the CISS effect but also display unique optical properties, such as the ability to absorb and emit circularly polarized light, unlocking exciting opportunities for technologies like spin LEDs and chiral detectors.
Despite progress, the underlying mechanisms of CISS remain poorly understood, as static helical models fail to align with experimental data. To address this, my team is developing a unified theoretical framework that captures the intricate quantum interactions in real materials. By integrating Density Functional Theory (DFT), Tight Binding (TB), and Machine Learning, we aim to model electronic, vibrational, and optical properties within a cohesive transport model. Early findings reveal that spin-phonon coupling plays a vital role in spin selectivity, highlighting the importance of dynamic quantum effects.
With support from a recently awarded ERC Consolidator Grant, we are expanding this framework to include new quantum interactions and transport calculations from both semiclassical and fully quantum perspectives. Once complete, this framework will bridge the gap between theory and experiment, offering powerful tools to advance our understanding of chirality and propel next-generation chiral technologies.
1.3-I1
Coupling chiral organic cations with the inorganic skeleton of perovskites is paving the way for new materials combining the second-order non-linear optical responses granted by the chiral molecules [1] and the modulable absorption and luminescence features settled by the perovskite or perovskite-derivative structures [2,3]. The heightened flexibility of design permitted by the vastity of available chemical constituents allows creating materials where the structural and functional parameters can be engineered, resulting in elevated circular dichroism, tunable photoluminescence, elevated spin selectivity, and more. Within this growing field, a deep understanding of how chemical composition and structural parameters impact on photophysical properties and chirality transfer mechanism is crucial, and parameters such as framework dimensionality, octahedral distortion and hydrogen bond network must be taken into account.
In this perspective, the current presentation will focus on novel hybrid organic inorganic perovskites (HOIPs) and their derivatives, attained through a rational tuning of the components to evaluate its impact on crystal structure and chiroptical properties. The effect of materials dimensionality on the chiral response will be discussed, including examples of 3D HOIPs derivatives which are not common due to the steric constrains of chiral cations. The metal centres impact on octahedra distortion, bandgap and chiroptical features will be examined, reporting results from our group with cations such as SnII and GeII in addition to the well-known PbII. The chiral cations modulation will also be presented, exploring molecules with mixed functionalities, i.e. simultaneously containing amino and hydroxyl groups, synthesized ad hoc to move away from the few commercially available ones. Finally, high-pressure studies unveiling the structural and optical response of chiral HOIPs upon external stimuli will be outlined.
1.3-I2
Juan José Palacios Burgos (Full Professor since 2019, UAM) graduated in Physics at the Universidad Autónoma de Madrid (UAM) in 1989 where he also received his PhD in 1993 for his theoretical work on electronic structure and transport properties of semiconductor quantum dots and on various topics related to the integer and fractional quantum Hall effect (QHE). He continued his professional career as a postdoctoral researcher at the National Research Council (Canada), the Indiana University (USA) as a NATO fellow, and the University of Kentucky (USA), where he explored more in depth the many-body physics of the fractional QHE in collaboration with Allan MacDonald, also doing fundamental work on vortex matter and mesoscopic superconductivity in collaboration with Nobel-prize awardee Andre Geim. At the Universidad of Alicante (Spain), where he worked for almost 10 years, he started a new research group on Nanophysics, a new master program in Nanoscience, and pioneered one of the first projects worldwide to compute quantum transport from first principles (Alicante NanoTransport, ANT). Since 2009 he works at the department of Condensed Matter Physics (UAM) where he is exploring the physics of two-dimensional and topological materials with focus on spintronics and optoelectronics applications. He has taught courses at all levels, including master studies. He has supervised 8 master students, 12 PhD students, and is currently supervising 6 PhD students more. In the meantime, in 2014, he co-founded SIMUNE Atomistics, the first company in Spain that offers computational services for material science related industrial needs, and was a Fulbright scholar in 2018 at University of Austin, in Texas. He is currently a member of Spanish as well as several international project evaluation committees.
It is generally accepted that spin-dependent electron transmission may appear in chiral systems, even without magnetic components, as long as significant spin–orbit coupling (SOC) is present in some of its elements [1]. However, this chirality-induced spin selectivity (CISS) can only manifest in experiments when system is taken out of equilibrium. Aided by group theoretical considerations and nonequilibrium DFT-based quantum transport calculations, here we show that, when spatial symmetries that forbid a finite spin polarization in equilibrium are broken, a net spin accumulation appears at finite bias in an arbitrary chiral two-terminal nanojunction. Furthermore, when a suitably magnetized detector is introduced into the system, the net spin accumulation, in turn, translates into a finite magneto-conductance [2]. These calculations have been possible thanks to new SOC implementation in our code ANT.Gaussian (https://github.com/juanjosepalacios/ANT.Gaussian). We also extend this analysis to chiral crystals where a similar phenomenology should be present in bulk. We do so thanks to a new code based on the computation of k-dependent transmission and polarization on top of a Hamiltonian obtained with CRYSTAL23.
1.2-I1
Three-dimensional tin halide perovskites are promising materials for photovoltaic applications due to their ability to achieve narrower bandgaps compared to their lead-based counterparts. However, a major challenge in advancing their performance is their intrinsic self-p-doping, which occurs even in the absence of external oxidizing conditions. This native p-type behavior is driven by the unique defect chemistry of the material, leading to reduced minority carrier lifetimes and ultimately limiting charge transfer, extraction, and power conversion efficiency. In this talk, we gain deeper insights into the defect chemistry of tin halide perovskites by combining experimental and computational approaches. Spectroscopic techniques, such as photoluminescence quantum yield and carrier lifetime measurements, are used to evaluate key optoelectronic figures of merit, while density functional theory calculations provided insights into defect formation energies. We first highlight pristine stoichiometric films and explored the role of SnF2, a commonly used additive in this class of materials, to understand its impact on defect passivation and optoelectronic properties. Then, we extend our studies to compositional engineering, systematically modifying: A-site cations, B-site cations through Sn-Pb mixing with varying ratios, and X-site halides by introducing iodide-bromide mixed systems. Our findings highlight strategies to modulate defect chemistry and charge carrier dynamics, offering pathways to optimize 3D Sn perovskites for efficient photovoltaic applications.
1.2-I2
Tin halide perovskites are highly interesting alternatives to lead-based materials for a range of optoelectronic applications. However, their defect content and characteristics, heavily determined by the crystallization process of these materials, has slowed down their development. In this talk, we will cover the main differences in solution properties between lead and tin defining the current limitations of the latter. We will also discuss the actual potential of traditional and novel strategies to deal with this challenge, particularly regarding the solvent nature. Finally, a wide range of solution and thin-film characterization will give us a comprehensive understanding of the key parameters and how to use them to effectively control tin-based perovskite crystallization. This talk aims to provide both an overview and a set of directions to overcome current limitations in tin halide perovskite applications through completely new processing systems. Furthermore, beyond tin-containing perovskites, the fundamental character of this work maked it of high interest also for other similar novel materials under development.
1.2-O1
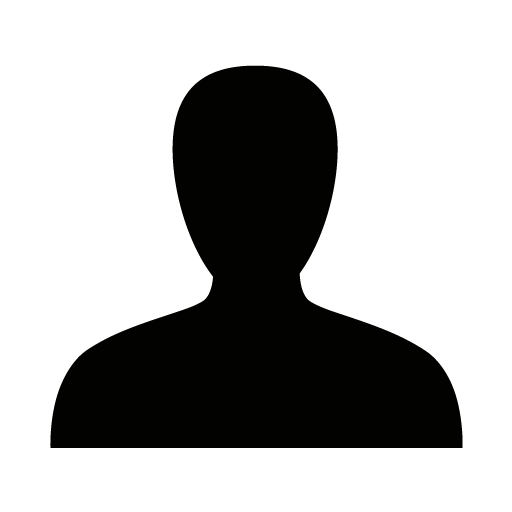
The development of new transparent conducting polymers has gained significant interest due to their potential in various optoelectronic applications. We report new generation of highly transparent bisEDOT-based conducting polymers as an alternative to PEDOT/PSS. The synthetic approach is based on the in situ polymerization of bis-EDOT, a dimer of 3,4-ethylenedioxythiophene, inside polymethylmetacrylate (PMMA) via an oxidative polymerization reaction by Cu(ClO4)2. This approach yields a homogeneous transparent conducting polymer with enhanced electrical conductivity, mechanical stability, and excellent film-forming properties, offering significant advantages for high-performance applications in electronic and optoelectronic devices. The bis-EDOT-based conducting polymer can be processed in the form of inks for the formation of layers with thickness control, the possibility of tuning electrical conductivity, high transparency in the visible and near-infrared spectrum, and the possibility of adapting its formulation with various solvents, and additives to be fully compatible with perovskite in manufactured devices. The resulting conducting polymer films exhibit superior charge transport properties and long-term stability, making them ideal candidates for hole transport materials (HTMs) in perovskite-based photovoltaics. Moreover, the simplicity, scalability, and cost-effectiveness of this method make it highly suitable for large-scale production, with applications extending to flexible electronics, organic light-emitting diodes (OLEDs), sensors, and transparent conductive coatings. Overall, this in situ polymerization strategy provides a promising route for fabricating high-performance materials for advanced electronic and energy conversion technologies.
1.3-I1
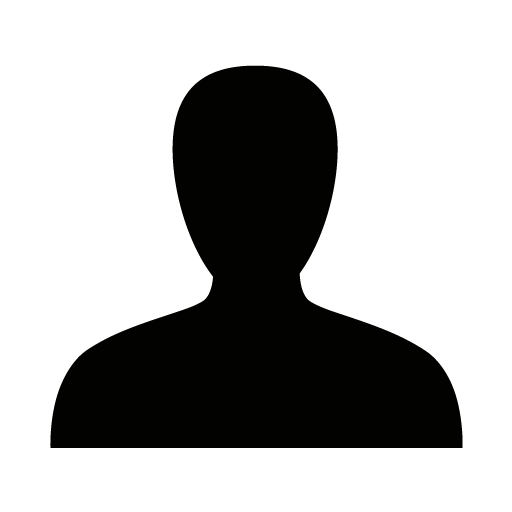
Layered metal-halide perovskites, or two-dimensional perovskites, can be synthesized in solution, and their optical and electronic properties can be tuned by changing their composition. In this talk, I will present a molecular templating method that restricted crystal growth along all crystallographic directions except for [110] and promoted one-dimensional growth out of the inherently layered 2D structures. This templating effect is achieved via introducing directional intermolecular interlayer hydrogen bonding interactions. This approach is widely applicable to synthesize a range of high-quality layered perovskite nanowires with large aspect ratios and tunable organic-inorganic chemical compositions (including Sn and Pb based perovskites, different quantum well thickness, and different halides). These nanowires form exceptionally well-defined and flexible cavities that exhibited a wide range of unusual optical properties beyond those of conventional perovskite nanowires. We observed anisotropic emission polarization, low-loss waveguiding (below 3 decibels per millimeter), and efficient low-threshold light amplification (below 20 microjoules per square centimeter). The 1D-2D mixed dimensional nanostructures provide unprecedented opportunities for next-generation optoelectronics and photonics.
1.3-I2
Juan P. Martínez-Pastor, Full Prof. at the University of Valencia. PhD in Physics, 1990. Three years of postdoctoral experience at the European Laboratory of Non-Linear Spectroscopy (Florence, Italy) and at the École Normale Supérieure (Paris, France). Prof. Martínez-Pastor is expert in Semiconductor Physics, particularly optical properties and exciton recombination dynamics in quantum wells, wires and dots based on III-V semiconductors and other compounds since 1990. This research line continues nowadays focused on quantum light produced by quantum dot semiconductors and its management for quantum communications. After 2006 he has leaded/co-leaded several research lines in nanoscience and nanotechnology regarding the development of several types of nanomaterials (metal and quantum dots, multi-functional nanocomposites) and applications to photonics and plasmonics. In the last three years, he focuses his research in optical properties, exciton recombination dynamics and applications in photonics of two-dimensional semiconductors and metal halide perovskites. He has supervised 16 PhD theses and is author/co-author of 220 peer-reviewed publications, other than seven patents and promotor of a spin-off company.
The continuous development in smart devices and microsystems for the control of industrial processes, biomedical sensors and instruments, visible and NIR light communications, as many other applications, is triggering new demands for photonic chips. Metal halide perovskites (MHPs) can be a good solution, because of their good optoelectronic properties and tolerance against crystalline defects, other than low-cost processing and low CO2 footprint. In the present talk the optical properties of several 2D MHPs of formula ABX3 (A = organic cation, B = Pb, Sn, X = I) have been investigated and will be presented in this talk.
First of all, basic optical properties of Pb-perovskites, as PEA2PbI4 (and higher order Ruddlesden-Popper phases), will be presented, both in the case of polycrystalline thin films and nanoflakes with lateral size greater than 10 µm. Moreover, these nanoflakes can be the base of micrometric photodevices by using Pt-prepatterned Si/SiO2 substrates with channel lengths in the range 2-10 µm. Measured photocurrent is highly dependent on the thickness flake due to the great absorption coefficient and negligible carrier transport in the vertical direction. Interestingly, in the case of few layer nanoflakes, photocurrents from 10 pA to 100 nA can be measured in the range 10 pW to more than 500 nW.
Sn-perovskites are also very interesting 2D semiconductors, because they are non-toxic alternatives of Pb-perovskites for applications as photodevices. However, the use of Sn-perovskites still suffers from very low stability and most of the synthesis, fabrication and/or characterization work must be done under inert atmosphere or vacuum, even if antioxidative synthetic routes can be followed for reducing the negative effect of ambient conditions. 2D tin-perovskites, TEA2SnI4, are gaining more stability and resistance to ambient condition, whose deposition is possible by scalable solution processing techniques as Inkjet-printing. Room- and low-temperature excitonic PL and charge carrier recombination dynamics in (TEA)2SnI4 thin films were studied and demonstrated two excitonic optical transitions. The analysis of micro-PL measurements suggests that the low-energy emission line is associated to the volume of perovskite grains (platelets), while the high-energy excitonic transition seems to be originated at the platelet edges (as identified in the biggest ones). Photoconductive detectors based on TEA2SnI4 inkjet-printed films were also studied after encapsulation. High electrical (dark currents as low as » 10 - 20 nA at 10 V of bias voltage) and electro-optical parameters (responsivities in the range 1-20 A/W) were obtained for these photodevices under ambient conditions over several weeks.
1.3-I3
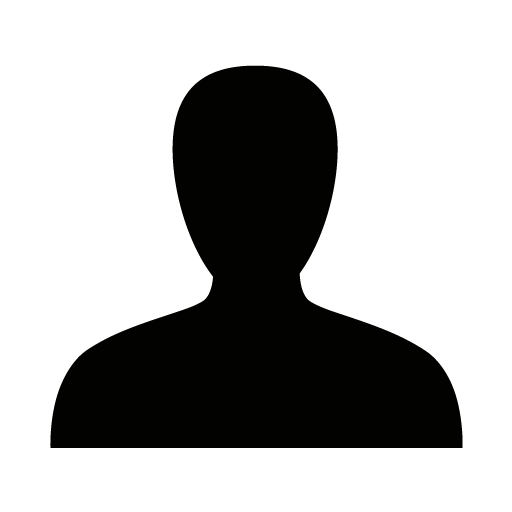
PhD in Chemistry in 2006 (University of Erlangen-Nuremberg (Germany)) for his thesis on the synthesis of electrically conducting polymers for electron beam lithography, which was carried out at Infineon Technologies AG (former company of Siemens AG).
In 2006 he joined the UMDO group of Prof. Juan Martinez-Pastor at the Materials Science Institute of University of Valencia as a postdoc where he launched a new research line in the field of synthesis of new nanomaterials for optoelectronics, photovoltaics and sensing.
At the end of 2009 he co-founded Intenanomat S.L., a spin-off company of the University of Valencia where he was the R&D manager for 7 years. Intenanomat is aimed at R&D of nanomaterials for applications in biotechnology, sensors, optoelectronics, catalysis and renewable energy.
In 2017 he joined again the University of Valencia with a Ramón y Cajal fellowship and launched a new research line in the field of nanomaterials for optoelectronics, photovoltaics, photocatalysis and sensing. In particular, he has a great interest in the synthesis and printing of nanocomposites of 2D materials (Lead Halide Perovskites and TMDs), inorganic nanoparticles (metal nanoparticles and metal chalcogenide quantum dots) embedded in polymers and metal oxides.
Metal halide perovskite (MHP) semiconductors have garnered significant research attention due to their exceptional physical properties, including long charge carrier diffusion lengths and high absorption coefficients. Furthermore, their synthesis is relatively straightforward, requiring mild conditions and scalable methods that utilize non-critical metals. These characteristics position MHPs as promising, cost-efficient materials for a broad range of applications in photovoltaics, photocatalysis, optoelectronics, and beyond.
Our research group is focused on synthesizing metal halide perovskites from macroscopic structures to nanocrystals (NCs), employing a variety of chemical routes, including traditional wet chemistry and in-situ synthesis. This talk presents a novel strategy for the in-situ synthesis of perovskite NCs embedded in a nanocomposite, achieving outstanding optical properties and enhanced stability.
Hybrid materials of this nature have attracted increasing interest over the past decade as they mitigate NC aggregation and improve long-term stability. By embedding NCs into suitable matrices, it is possible to engineer multifunctional materials that combine optical, mechanical, electrical, thermal, electrochemical, or photocatalytic properties within a single system.
Over the past two years, we have developed an innovative in-situ synthesis method for various perovskite NCs with different compositions and dimensionalities. This approach involves the incorporation of MHPs into a metal- organic matrix (e.g., nickel acetate, magnesium acetate) to produce nanocomposite thin films via solution processing under ambient conditions, eliminating the need for an inert atmosphere (N₂ glovebox) [1,2,3,4].
We report the successful synthesis of 3D bulk lead-based perovskite NCs with different halides (Cl⁻, Br⁻, I⁻), incorporating both organic (MA⁺, FA⁺) and inorganic monovalent cations (Cs⁺). For specific compositions, we achieve near-unity photoluminescence quantum yield (PLQY). Furthermore, including bulky organic cations such as PEA⁺ and TEA⁺ enables the formation of low-dimensional tin-based perovskites with remarkable stability. In particular, the TEA-Sn molar ratio plays a crucial role in driving the formation and stabilization of 0D Sn-based MHPs, exhibiting exceptional photoluminescence and environmental resilience [5]. Additionally, we can also synthesize Ag- and Bi-based halide double perovskite NCs (i.e. Cs₂AgBiBr₆) with promising potential for catalytic applications.
This method stands out for its exceptional reproducibility and process reliability under low-demanding fabrication conditions, making it an ideal platform for testing the synthesis of novel perovskites proposed through machine- learning approaches. Its key advantage lies in its high versatility and seamless compatibility with high-throughput roll-to-roll (R2R) printing techniques, enabling the scalable and cost-effective fabrication of large-area, high- performance devices. This approach has already shown a broad range of applications in photocatalysis [4], photovoltaics and light-emitting technologies such as lasing [6], down-conversion, and gas sensing.
2.1-I1
Yong-Young Noh is Chair Professor in the Department of Chemical Engineering, Pohang University of Science and Technology (POSTECH), Pohang, Republic of Korea. He received his PhD in 2005 from GIST, Republic of Korea, and then worked at the Cavendish Laboratory in Cambridge, UK, as a postdoctoral associate. Afterwards, he worked at ETRI as a senior researcher, at Hanbat National University as an assistant professor, Dongguk University-Seoul as an associate professor. He has won Merck Young Scientist Award (2013), Korea President Award (2014), IEEE George E. Smith Award (2014), as selected this month as a Scientist from Korea Government (September. 2016), and the Order of Science and Technology Merit, Science Medal from Korea Government (April 2024). He is a fellow of Fellow of Korean Academy of Science and Technology and a Fellow of The National Academy of Engineering of Korea. He has published over 360 papers in international journals in the field of materials for electronics and optoelectric devices, in particular, OFETs, OLEDs, Metal Halide, perovskites, carbon nanotube 2D layered materials, and oxide TFTs. Selected Recent Papers: Selenium alloyed tellurium oxide for amorphous p-channel transistors, Ao Liu, Yong-Sung Kim, Min Gyu Kim, Youjin Reo, Taoyu Zou, Taesu Choi, Sai Bai, Huihui Zhu, Yong-Young Noh, Nature, 629, pages798–802 (2024), Tin perovskite transistors and complementary circuits based on A-site cation engineering, Huihui Zhu, Wonryeol Yang, Youjin Reo, Guanhaojie Zheng, Sai Bai, Ao Liu, Yong‐Young Noh, Nature Electronics, 6(9), 650-657 (2023), High-performance metal halide perovskite transistors, Ao Liu, Huihui Zhu, Sai Bai, Youjin Reo, Mario Caironi, Annamaria Petrozza, Letian Dou, Yong-Young Noh, Nature Electronics, 6(8), 559-571 (2023), High-Performance Inorganic Metal Halide Perovskite Transistors, Ao Liu, Huihui Zhu, Sai Bai, Youjin Reo, Taoyu Zou, Myung-Gil Kim, Yong-Young Noh*, Nature Electronics 5(2), 78-83 (2022).
Enable GingerCannot connect to Ginger Check your internet connection
or reload the browserDisable GingerRephraseRephrase with Ginger (Ctrl+Alt+E)0Edit in GingerGinger is checking your text for mistakes...Disable Ginger in this text fieldDisable Ginger on this website×
Developing high-mobility p-type semiconductors that can be grown using silicon-compatible processes at low temperatures, has remained challenging in the electronics community to integrate complementary electronics with the well-developed n-type counterparts.
This presentation will discuss our recent progress in developing high-performance p-type semiconductors as channel materials for thin film transistors. For the first part of my talk, I will present high-performance tin (Sn2+) halide perovskite transistors using high-crystallinity and uniform cesium-tin-triiodide-based semiconducting layers [1.2]. The optimized devices exhibit high field-effect hole mobilities of over 50 cm2 V−1 s−1, large current modulation greater than 108, and high operational stability and reproducibility [3]. In addition, we explore triple A-cations of caesium-formamidinium-phenethylammonium to create high-quality cascaded Sn perovskite channel films. As such, the optimized TFTs show record hole mobilities of over 70 cm2 V−1 s−1 and on/off current ratios of over 108, comparable to the commercial low-temperature polysilicon technique level.
Next, I present an amorphous p-type oxide semiconductor composed of selenium-alloyed tellurium in a tellurium sub-oxide matrix, demonstrating its utility in high-performance, stable p-channel TFTs, and complementary circuits [4]. Theoretical analysis unveils a delocalized valence band from tellurium 5p bands with shallow acceptor states, enabling excess hole doping and transport. Selenium alloying suppresses hole concentrations and facilitates the p orbital connectivity, realizing high-performance p-channel TFTs with an average field-effect hole mobility of ~15 cm2 V-1 s-1 and on/off current ratios of 106~107, along with wafer-scale uniformity and long-term stabilities under bias stress and ambient aging.
References
[1] A. Liu, Y.-Y. Noh et al, Nature Electronics 5, 78-83 (2022)
[2] H. Zhu, Y.-Y. Noh et al, Nature Electronics 6, 650-657 (2023)
[3] A. Liu, Y.-Y. Noh et al, Nature Electronics 6, 559-571 (2023)
[4] A. Liu, Y.-Y. Noh et al, Nature, 629, 798–802 (2024)
2.1-I2
Low-dimensional (LD) organic metal halide hybrids (OMHHs), comprising diverse organic cations and metal halide anions, represent an emerging class of perovskite-related hybrid materials with exceptional structural and property tunability. By carefully selecting organic and metal halide components, their crystallographic structures can be precisely tailored at the molecular level, with metal halide units forming two-dimensional (2D), one-dimensional (1D), or zero-dimensional (0D) structures. The site isolation and confinement of metal halides by organic cations endow LD OMHHs with unique properties distinct from conventional three-dimensional (3D) metal halide perovskites. For example, 2D and 1D OMHHs have demonstrated broadband white emissions, while 0D OMHHs have achieved near-unity photoluminescence quantum efficiency (PLQE) with tunable emissions spanning blue, green, yellow, orange, and red wavelengths. The true significance of LD OMHHs lies not only in these specific accomplishments but also in their role as a new paradigm in materials design. In this talk, I will present our recent progress in the development and study of LD OMHHs, from synthetic control to device integration. Applications of LD OMHHs in various areas, including optically pumped white LEDs, electroluminescent devices, X-ray scintillators, and direct X-ray detectors will be discussed.
2.1-O1
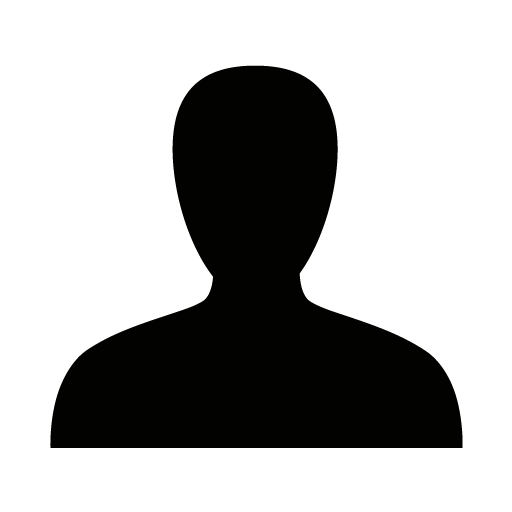
Cs2TiBr6, a promising lead-free and earth-abundant double perovskite, exhibits excellent photovoltaic and optoelectronic potential due to its 1.8 eV bandgap and theoretical stability under various conditions. Despite its advantages, challenges in conventional synthesis—such as high temperature, pressure, and solubility issues—have limited its practical application. Additionally, its susceptibility to air-induced degradation raises further concerns about its stability.
This work introduces a novel, microwave-assisted synthesis method that significantly reduces time, temperature, pressure, and cost while maintaining structural stability under diverse atmospheric conditions, including air, oxygen, white light, and temperatures exceeding 130°C. A gradual cation exchange process was implemented to enhance stability by substituting Ti4+ with Sn4+ in the efficient microwave-assisted synthesis method, developing a double perovskite Cs2SnxTi1-xBr6 type. A systematic study of Sn-doping revealed improved air stability for over a week, uniform polygonal crystal morphology, and a slight bandgap broadening.
This efficient and sustainable synthesis approach offers a pathway to more durable and environmentally friendly perovskites, unlocking new opportunities for advanced optical and electronic devices.
2.1-O2
Gustavo de Miguel graduated in Chemistry in 2002 by the University of Cordoba, Spain. He completed his PhD Thesis in the Physical Chemistry Department of the same University in 2007 studying the molecular organization of thin films prepared at the air-water interface. After several post-doc positions in the Friedrich-Alexander University of Erlangen-Nuremberg, University of Castilla-La Mancha and the Italian Institute of Technology, he moved back to the University of Cordoba with a Ramón y Cajal five-year tenure track position, becoming Associate Professor in 2020.
Dr. de Miguel is a physical chemist with an expertise in absorption and photoluminescence spectroscopy (steady-state and time-resolved) applied to elucidate the photophysics and photochemistry of organic compounds with application in photovoltaics. In the last years, he has added a good knowledge of structural characterization of hybrid materials (perovskites) through different X-ray diffraction techniques.
He participates in National and European projects focusing on how to enhance the stability of metal halide perovskite materials for photovoltaics (SUNREY, Ref:101084422). He has contributed with about 100 publications in international peer-reviewed journals.
Quasi-2D metal halide perovskites (MHPs) employed in photovoltaics involve the use of monoammonium or diammonium spacer cations to form the Ruddlesden-Popper or the Dion-Jacobson phases, respectively.1 Moreover, the chemical nature of the bulky cations plays a significant role in the optoelectronic properties of the quasi-2D MHPs, controlling the orientation of the inorganic octahedral layers, the interlayer distance and thickness of the inorganic layers, phase distribution and the dielectric constant and dipole moment of the organic barriers which all determines the charge transport in the quasi-2D MHPs.2 Moreover, additive engineering and processing strategies can also modulate the charge carrier transport.
We have used a p-conjugated bulky cation, 4,4′-diaminostilbene dihydrochloride (Sb), to synthesize a Dion-Jacobson 2D MHPs that is employed to fabricate photovoltaic devices. The relative ratio of the employed precursors is selected to define the n = 5 phase, (Sb)FA2.8MA1.2Pb5I15. However, a distribution of low dimensional phases is found (n = 1, 2 and 3) although other phases with longer n values are also present. The quasi-2D DJ MHP in the film is highly oriented and the deactivation of the photoexcited charge carriers (mostly excitons) is through a radiative recombination pathway assisted by the quantum confinement in the low dimensional phases. This fast deactivation of the excited state prevents a rapid extraction of the charges from the material and therefore, the short circuit current (Jsc) in the solar devices prepared with this perovskite is low, Jsc = 9.03 mA·cm-2.
On the contrary, the addition of the MASCN additive to the initial precursor solution modifies the distribution of the phases in the film. Thus, the low dimensional phases are scarce now but phases with higher dimensionality are more present in these films. This is clearly demonstrated by the absence of steady-state and transient absorption features of the low dimensional phases. Moreover, the PL decays display a much longer time constant, which is a hint for a less intense quantum confinement in these samples. The reduction of the defect concentration calculated with the space-charge limited current measurements suggests that the excess of the stilbene derivative is localized in between the perovskite grain passivating the superficial defects and, therefore, reducing the non-radiative recombination pathway.
2.2-I1
Scintillating materials aim to detect ionizing radiations and are currently widely used in many detection systems addressing different fields, such as medical imaging, homeland security, high energy physics (HEP) calorimetry, industrial control, and oil drilling exploration. Quality criteria for these materials span over several parameters, three of which are of primary importance: the scintillation yield, the density, and the timing response. In the case of the interaction with a high-energy photon such as X-ray or gamma ray, the time response shows a very complex structure in the multi time-scale regime, making it critical for several applications. Solution-processable perovskite scintillators have been shown to be the solution for the replacements for the current expensive lanthanide scintillators as they share the same or even better properties for state-of-the-art imaging and detection applications. As examples, time-of-flight (TOF) functionalities require time resolution below 100 ps, coincidence techniques often need sub-tens of ns time response [1], counting regime detection prefer sub-μs time response, and afterglow over ms is detrimental for X-ray imaging [2]. Among all perovskite materials, two-dimensional lead halide perovskites have shown remarkable environmental and thermal stability, a large Stokes’ shift, usually coupled with very broad emission compared to their three-dimensional and quantum dot counterparts [3]. Here, we will show the progress for the research towards those applications since the beginning of our activities with perovskite scintillators. Moreover, we will discuss our approaches to tackle problems in some perovskite materials through energy sharing concept and nanophotonic structures. The latter will bring faster and brighter scintillators through Purcell enhancements while we will demonstrate how we can reach this goal through photonic crystals and plasmonic structures [4.5]. Such visions will pave the way towards new research directions and applications on the high-energy physics and nanophotonics interactions.
2.2-I2
The author was graduated from Osaka University in 1978 and received Ph.D from Osaka University in 1983. He joined R&D Center in Toshiba from 1978 to 2000, during which the author was engaged in development of ULSI lithography, solar cells direct methanol fuel cells, and polysilane. He joined polysilane research in Robert West group of Wisconsin University (US) from 1988 to 1990. He was a professor of Kyushu Institute of Technology (National Institute) since 2001. From 2019, the author is a professor in The University of Electro-Communications in Japan. His research interest is printable solar cells.
The efficiencies of tin-lead alloyed perovskite solar cell and lead-free tin perovskite solar cells are now 24-25% and 15-16%, respectively, which are still lower than that of lead perovskite solar cells. Besides the efficiency enhancement of these tin-based solar cells, stability improvement is another important research theme. In this presentation we focuse on the stability improvement of these tin-based perovskite solar cells. We learnt from the stability study of lead perovskite solar cells that ion-migration has to be suppressed for the improvement of stability. To do this for the tin-lead alloyed perovskite solar cells, Ge ions which finally cover the grain boundary as GeOx, were added to the perovskite layer. In addition, ALD SnOx layer was inserted between the electron-transporting layer (Fullerenes). The stability of the tin-lead alloyed perovskite solar cells was improved drastically. The conventional composition consisting of FTO/PEDOT-PSS/SnPb-PVK/PCBM/C60/BCP/Ag degraded to 40% of the initial efficiency after the solar cell was put in the 85 ℃ under N2 atmosphere for 200 h. The efficiency decrease of the improved solar cell was suppressed to around 5% of the initial efficiency after the sample was kept for 1000h in the same condition. It is well-known that PEDOT-PSS frequently employed as the hole-transporting layers damages the tin-based perovskite layer by the proton migration. The proton migration is somehow retarded by the GeOx. The ALD SnOx layer suppressed the iodine migration to the electron-transporting layer and the Ag electrode. In the same way, the thermal stability of the lead-free tin perovskite solar cell was improved. The efficiency of the conventional solar cells consisting of FTO/PEDOT-PSS/Sn-perovskite/C60/BCP/Ag decreased to 20% of the initial efficiency after the sample was put in the 85 ℃ under N2 atmosphere for 100 h. The decrease of the improved sample was suppressed to 20% after the sample was kept for 400 h in the same condition. It was proved that the thermal stability of the tin-based perovskite solar cells is improved by suppressing the ion migration, which is similar to that of lead perovskite solar cells.
2.2-I3
Tin-based halide perovskites (THPs) have emerged as promising candidates for both photovoltaics and near - IR light emitting applications thanks to their high carrier mobilities, low band gap, ideal for pairing with silicon in tandem solar cells[1,2]. THPs present highly stable acceptor defects such as Sn vacancies and I interstitials, which result in a permanent population of holes: the material than behaves as a p-doped semiconductor, with self-doping densities as high as 1022 cm-3 that can negatively impact device performances. As such, it is of critical importance to devise strategies to both quantify and control the dopant hole densities in THPs by compensating the oxidation state of Sn during material fabrication using additives such as SnF2.
In this context, terahertz (THz) spectroscopy represents a powerful tool to characterize the carrier populations and dynamics in THPs. THz radiation is sensitive to mobile charged carriers[3], as well as their coupling with lattice phonons[4]: it can then be used to shed light on charge transport properties in THPs, as low frequency phonons in the THz range have been shown to limit their thermal and electrical conductivity. Moreover, as the doping density affects both transparency to THz radiation and carrier phonon coupling, THz absorption can be used as a sensitive, contactless probe to characterize the self – doping density even in samples where it has been brought down to levels comparable to background carrier densities suitable for device applications (e.g. 1015 cm-3), and that could be challenging to characterize by traditional Hall effect measurements. Furthermore, time resolved THz spectroscopy after optical excitation of the material allows to follow the dynamics of photogenerated carriers with ps temporal resolution.
Here we develop a robust technique to study the doping hole density in FACsSnI3 thin films by analyzing the static THz conductivity response, and characterize ultrafast carrier dynamics using time resolved THz spectroscopy. By supporting our results with DFT calculations, we also investigate the effect of doping concentration and defect states on optical phonon frequencies and their coupling to charge carriers. Finally, the possibility of studying the material polaronic response by combining THz and XUV absorption spectroscopy will be discussed.
1.3-I1
The quest for low cost, non-toxic and Earth abundant materials solutions for thin film PV, has sparked significant interest in new inorganic semiconductors with complex compositions. One of the challenges with material discovery and new material compositions, is their fabrication in thin film form. Challenges such as volatility or solvent incompatibility, hinders progress in either high quality material demonstration or functionality via device integration.
To overcome these barriers, we employ mechanochemical synthesis to identify promising halide and chalco-halide powders as candidate materials. These powders serve as targets for thin-film deposition via pulsed laser deposition (PLD), a technique that uniquely enables near-stoichiometric transfer of complex compositions, regardless of elemental volatility. This capability facilitates the fabrication of high-quality thin films for proof-of-concept solar cells, with critical PLD parameters optimized to tailor the properties of complex halide, chalco-halide, and chalcogenide semiconductors.
The versatility of PLD is further leveraged for material discovery through compositional gradient screening on substrates, enabling rapid evaluation via photoluminescence and compositional mapping. The resulting materials, selected based on their band gaps, are explored as photo-absorbers or contact layers, advancing their integration into thin-film solar cell devices. This presentation will highlight strategies for experimental material discovery, thin-film synthesis, and device integration, contributing to the development of the next generation of optoelectronic materials and devices.
References:
https://pubs.acs.org/doi/10.1021/acsenergylett.4c01466
https://doi.org/10.1002/adfm.202316144
https://doi.org/10.1021/acs.chemmater.1c02054
https://doi.org/10.1021/acs.chemmater.3c01349
https://doi.org/10.1016/j.matt.2023.10.003
1.3-I2
Bismuth-based semiconductors, including the double perovskite Cs₂AgBiBr₆ but also perovskite-inspired materials such as bismuth oxyhalides, show great promise for sustainable light-energy conversion due to their low toxicity, abundance, and tunable electronic properties. This presentation will explore strategies to enhance the efficiency, stability, and scalability of these materials in photoelectrocatalytic and photovoltaic applications. Methods like automated film production, surface modifications, and heterojunction formation have been employed to improve the performance of BiOI and BiOBr in water splitting and hydrogen evolution reactions. A continuous automated film production method for BiOI photoelectrodes was introduced, significantly improving the reproducibility and efficiency of large-scale production. Surface modifications and heterojunction formation have been explored to optimize PEC performance, with enhanced water oxidation and hydrogen evolution reactions observed. Additionally, the lead-free double perovskite Cs₂AgBiBr₆ was optimized for use in solar cells with improved efficiency through interface engineering and low-cost carbon-based electrodes. These advancements position bismuth-based semiconductors as viable, eco-friendly alternatives for energy conversion technologies.
1.3-I3
Chalcogenide perovskites have much to recommend them for photovoltaics (PV). They absorb light strongly, with direct band gap tunable (at least) over the range 1.4 – 1.9 eV. They have limited polymorphism and are stable in air, water, and at high temperature. They are made of Earth-abundant, and (mostly) non-toxic elements, and have isotropic properties. However, there are substantial challenges facing the development of chalcogenide perovskite PV. Synthesis requires aggressive conditions - oxygen-free sulfurization or selenization at high temperature – that severely constrain thin-film growth. The few published reports of transport properties describe n-type material with high electron concentration, undesirable for thin-film PV. Photoluminescence (PL) has been reported, but the quantum yield is often low, and sample-to-sample variability is high. The defects that limit performance are not yet understood, and even less is known about interface and heterojunction design. Clearly, it will be a long road to chalcogenide perovskite PV technology.
I will motivate why, despite these challenges, research on chalcogenide perovskites for PV is worthwhile and exciting. I will then describe our own efforts, which center on the processing and properties of thin films. We have achieved a number of synthesis milestones, including growing thin films of BaZrS3 and BaZr(S,Se)3 alloys with tunable band gap, in epitaxial and polycrystalline forms. Selenium alloying can produce films with band gap suitable for single- and dual-junction PV, but the vast majority of synthesis procedures reported to-date focus on pure sulfides. I will discuss our finding of rapid alloying by post-growth selenization of sulfide thin films. This recalls the sulfurization-after-selenization process in CIGS manufacturing, and may make alloy studies more widely accessible. I will also present findings on how variations in cation composition affect crystallization kinetics. These results bolster evidence for BaS3-liquid-assisted crystal growth, and may be useful for lowering the temperature of thin film synthesis.
I will then discuss our ongoing studies of photoluminescence (PL) and electronic transport. We have previously reported long excited-state PL lifetimes for BaZrS3 and Ba3Zr2S7, and others have reported band-edge PL even from powder samples. However, PL emission is highly variable, sample-to-sample, and many samples have no measurable band-edge emission. To understand this variability, we carry out a quantitative comparison of temperature-dependent PL of BaZrS3 and a prototypical halide perovskite, CsPbBr3. The halide has PL yield between 100 and 10,000 times larger than the chalcogenide. By comparing the vibrational properties of the chalcogenide and the halide, we suggest why defect-assisted recombination may be faster in the chalcogenide. On the other hand, the variability between chalcogenide samples suggests a substantial upside, if the recombination-active defect(s) can be identified and diminished. Our temperature-dependent Hall transport studies find that mobility at room temperature is limited by electron-phonon scattering, even in highly-doped samples; this may be related to our previous finding that chalcogenide perovskites have exceptional dielectric polarizability. At cryogenic temperature, the role of ionized defect scattering varies sample-to-sample. We also find that photoconductive responsivity varies tremendously from sample-to-sample, apparently due to variations in processing that affect the concentration of extended defects. All films are n-type as grown, but with tremendous variability in electron concentration. Studies of post-growth annealing support the hypothesis that the predominant intrinsic shallow donors are sulfur vacancies; we use this understanding to vary electron concentration by over a million-fold.
I will end by highlighting exciting next-steps including alternative methods of thin film deposition to make thicker films at lower temperature, studies of device semi-fabricates including detailed investigation of Mo/BaZrS3 interfaces, and controlling carrier concentration.
1.3-O1
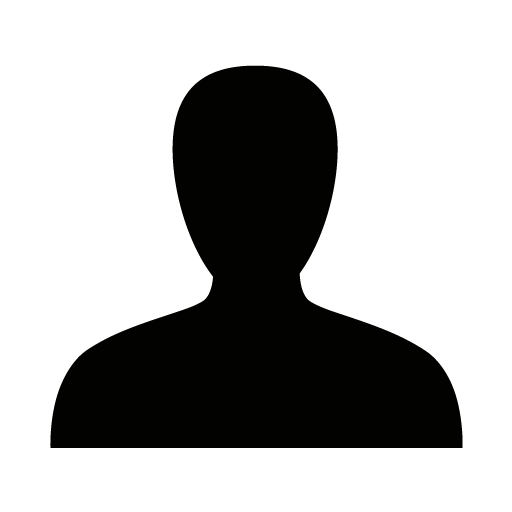
Chalcogenide perovskites are a new emerging group of Pb-free perovskites featuring high environmental stability, direct band gap with extraordinary absorption coefficient, and good carrier transport properties, that can be suitably tailored for photovoltaic applications. Their constituent elements can vary, with the A and B cations having oxidation +2 and +4, respectively, while the anion is a chalcogen, such as S, Se, and Te, with an oxidation state -2. Currently, the most researched chalcogenide perovskite is BaZrS3, due to its natural abundance of elements and its bandgap, which is favorable for tandem solar cells.
A prerequisite to adapt BaZrS3 in device architectures is to understand how the complex surface chemistry affects optoelectronic properties of BaZrS3 thin films. Our research focuses on the study of the BaZrS3 surface/interfaces, developing methodologies for investigating the interfaces and the band alignment for devices. In our work, the measurements of the surfaces for varied films are achieved by photoelectron spectroscopy (XPS) performed both at in-house laboratories and at synchrotrons, exploiting the variable X-ray energy and the high flux.
In this presentation, I will report the advances of the BaZrS3 as studied in our research on BaZrS3 thin films. First, the bulk quality of our samples will be highlighted correlating the bulk properties to the functionality in terms of XRD, XAS and PL [1] . The surface chemistry and electronic structure, specifically orbitally-resolved valence band characteristics in relation to charge-carrier type, will be described as revealed by XPS measurements, differentiating the perovskite peaks from the secondary phases and performing a depth profile analysis of the top few nanometers from the film surface [2]. Our recent highlight includes in situ high-temperature post-annealing in ultra-high vacuum, allowing us to access the perovskite peaks by soft X-ray XPS from the purest film surface achieved so far. Our work is fundamental for unraveling the interfacial properties and the band alignment that directly impact charge transport. The findings will help identifying optimal design parameters for utilization of chalcogenide perovskites in optoelectronic devices, such as photodiodes and solar cells.
2.1-I1
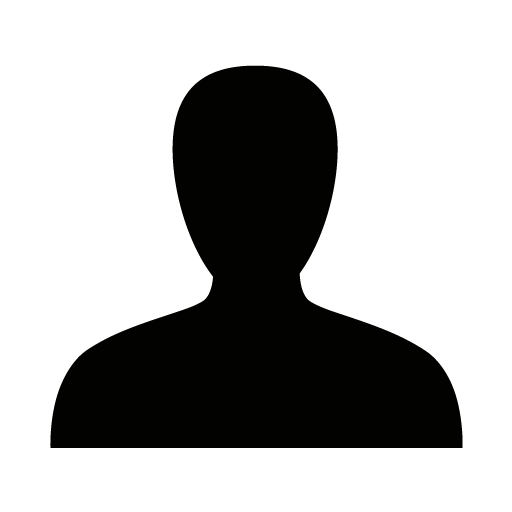
Ternary nitrides represent an emerging class of materials with immense potential in solar energy conversion, thermoelectrics, power electronics, coatings, and superconductivity, combining distinctive bonding properties, defect tolerance, and tunable functionalities. [1], [2] However, challenges in synthesis and metastability have limited their exploration compared to oxides. Recent synthetic and computational advances are now opening pathways for their development as next-generation solar materials.
This talk showcases two visible-light-absorbing nitrides that have recently emerged and offer especially interesting optoelectronic properties for solar energy conversion, copper tantalum nitride (CuTaN₂) [3] and zirconium tantalum nitride (ZrTaN₃) [4], with an emphasis on complex physical interactions that define their electronic structures. CuTaN₂ exhibits highly anharmonic structural dynamics, as displayed by phonon calculations and finite-temperature Raman experiments. Ab initio molecular dynamics is used to reveal the microscopic mechanisms of atomic motion, which are linked to macroscopic properties including its negative thermal expansion and temperature-dependent increase in the bandgap, thus emphasizing the critical role of structural dynamics in defining optoelectronic properties. In a second example, ZrTaN₃ thin films synthesized via reactive magnetron co-sputtering are shown to exhibit strong visible light absorption and significant photoelectrochemical activity. Complementary density functional theory calculations reveal that cation disorder, particularly Wyckoff-site occupancy, significantly modulates the bandgap and orbital hybridization in this ternary compound, underscoring the impact of cation arrangement on optoelectronic properties.
These findings highlight the versatility of ternary nitrides as advanced photoactive materials and offer insights into tailoring their properties through atomic-scale engineering.
2.1-O1
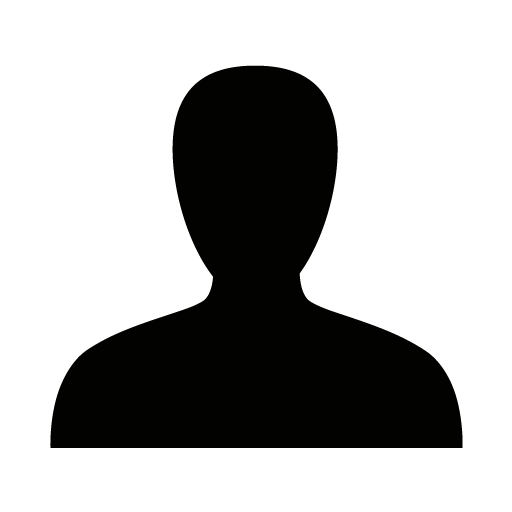
Toxicity and instability of lead-based metal halide perovskites (MHP) have fueled explorations of new lead-free all-inorganic materials, especially in the CuI-AgI-BiI3 phase space. In particular, Cu2AgBiI6 shows promising optoelectronic properties such as a high absorption coefficient of ~ 105 cm-1, a direct band gap of ~ 2 eV, a low exciton binding energy comparable to the thermal energy at room temperature, a modest mobility of 1.7 cm2V-1s-1 and a nanosecond charge-carrier lifetime.[1] However, the champion power conversion efficiency (PCE) of the Cu2AgBiI6 solar cells was only 2.39 %,[2] significantly lagging behind the PCE of the MHP counterparts. It has been shown that Cu2AgBiI6 exhibits ultrafast charge-carrier localization, which imposes the fundamental limits on its PCE.[3] However, such low PCEs cannot be solely attributed to the ultrafast charge-carrier localization, given that Cs2AgBiBr6, which also shows ultrafast charge-carrier localization and has an indirect bandgap, has achieved the champion PCE of 6.37 %.[4]
Herein, we aim to understand additional factors limiting the PCE of Cu2AgBiI6 beyond intrinsic ultrafast localization by investigating optoelectronic properties of charge transport layer (CTL)/Cu2AgBiI6 half stacks using CuI and PTAA as hole transport layers and SnO2 and PCBM as electron transport layers. By analysing absorption spectra, X-ray diffraction (XRD) patterns and optical-pump terahertz-probe (OPTP) transients, we observe that the formation of quaternary phase Cu2AgBiI6 is influenced by the deposition of charge transport layers, especially by CuI and SnO2, which modify its optoelectronic properties. Materials within the CuI-AgI-BiI3 phase space share similar band gaps and lattice parameters, making it challenging to confidently distinguish Cu2AgBiI6 from other impurity phases by using absorption spectra and XRD patterns alone. By extracting THz mobilities from OPTP, we confidently factor out Cu2AgBiI6 phase and identify impurity phases induced by the transport layer deposition. We find that deposition of CuI on Cu2AgBiI6 induces the formation of copper-rich quaternary CuxAgBiI4+x phases near the interface and deposition of Cu2AgBiI6 on SnO2 hinders the formation of the quaternary phase and instead forms ternary and binary phases, leading to the decrease in the mobility. Overall, we highlight that the deposition of CTLs can significantly affect the formation and optical properties of Cu2AgBiI6. Characterization of CTL/Cu2AgBiI6 half stacks is therefore critical to improve the device performance. Moreover, further developments are needed to suppress the formation of unwanted impurity phases upon deposition of transport layers.
2.2-I1
Lead-free halide double perovskites (HDPs, A2BB’X6) with attractive optical and electronic features are regarded as one of the most promising alternatives to overcome the toxicity and stability issues of lead halide perovskites. They provide a wide range of possible combinations and rich substitutional chemistry with interesting properties for various optoelectronic devices.[1] However, the performance of state-of-the-art lead-free HDPs is not yet comparable to that of lead halide perovskites, especially in the photovoltaic field.[2] One of the main reasons for this is that HDPs usually have large and/or indirect bandgaps, which limit their optical and optoelectronic properties in the visible and infrared regions. In this presentation, I will talk about our work on double perovskites, including the fabrication of the first planar double perovskite solar cell devices, and the modification of the bandgap and optical properties of HDPs using metal doping/alloying and crystallization control,[3],[4] as well as provide detailed understanding of the alloying at the atomic level.
2.2-I2
Thomas Bein received his PhD in Chemistry from the University of Hamburg (Germany) and the Catholic University Leuven (Belgium) in 1984. He continued his studies as Visiting Scientist at the DuPont Central Research and Development Department in Wilmington, DE (USA). From 1986 to 1991 he was Assistant Professor of Chemistry at the University of New Mexico in Albuquerque (USA). In 1991 he joined Purdue University (Indiana) as Associate Professor, and was promoted to Full Professor of Chemistry in 1995. In 1999 he was appointed Chair of Physical Chemistry at the University of Munich (LMU), where he also served as Director of the Department of Chemistry.
He has recently won an ERC Advanced Grant entitled “Electroactive Donor-Acceptor Covalent Organic Frameworks”. Presently he is LMU-Coordinator of the newly funded Excellence Cluster “e-conversion”. Bibliographic data: Over 500 publications, over 38.000 citations, h=115. Since 2018, Thomas Bein is listed as a Highly Cited Researcher (Clarivate).
His current research interests cover the synthesis and physical properties of functional nanostructures, with an emphasis on porous materials for targeted drug delivery and nanostructured materials for solar energy conversion.
URL: http://bein.cup.uni-muenchen.de/
In the search for lead-free alternatives for halide perovskites, a number of different research routes are being pursued. Here we will discuss three different pathways presently under investigation. For example, silver pnictohalides have emerged as perovskite-inspired materials for photovoltaics due to their high stability, low toxicity, and promising early efficiencies, particularly for indoor applications. While most research has focused on silver bismuth iodides (Ag–Bi–I rudorffites), antimony analogs remain underexplored due to difficulties in synthesizing Sb-based thin films. Here, a novel synthesis route using thiourea as a Lewis-base additive enabled the preparation of Ag–Sb–I films, which were further optimized by Cu alloying to improve thin-film morphology and increase power conversion efficiency to 0.7% [1]. Theoretical and optical studies confirmed Cu incorporation into a Cu₁₋ₓAgₓSbI₄ phase without altering bandgap properties. Our studies also identified Ag point defects as traps reducing open-circuit voltage, with minor Bi additions enhancing efficiency and stability.
Heteroatom alloying presents an additional strategy for tuning the optoelectronic properties of lead-free perovskite derivatives. Tin-alloyed layered MA₃Sb₂I₉ thin films were synthesized using a solution-based approach with precursor engineering, blending acetate and halide salts [2]. Increasing tin halide concentrations expanded visible-spectrum absorption and improved stability. Tin incorporation into the MA₃Sb₂I₉ lattice introduced new electronic states in the bandgap, confirmed by theoretical calculations. These features enhanced absorption through intervalence and interband transitions while stabilizing charge transport. This system’s robustness toward mixed oxidation states improved ambient stability, demonstrating its potential for various optoelectronic applications.
Finally, the integration of organic semiconducting materials with inorganic halide perovskites offers promising opportunities for tuning optoelectronic properties. Using stable, nontoxic double perovskites as hosts for electroactive organic cations enables the creation of two-dimensional (2D) hybrid materials that are both functional and lead-free for optoelectronic applications. By incorporating naphthalene and pyrene moieties into Ag–Bi–I and Cu–Bi–I double perovskite lattices, we address intrinsic electronic limitations of double perovskites, and modulate the anisotropic electronic properties of 2D perovskites [3]. Among eight newly developed 2D double perovskites, (POE)₄AgBiI₈ containing pyrene moieties was identified as having a favorable electronic band structure, exhibiting a type IIb multiple quantum well system conducive to out-of-plane conductivity and achieving a photocurrent response ratio of nearly three orders of magnitude under AM1.5G illumination. This material was used to create the first pure n = 1 Ruddlesden–Popper 2D double perovskite solar cell. Concluding, these and additional examples discussed in the presentation illustrate the enormous design space available for the generation and tuning of novel lead-free perovskite-derived absorber materials.
[1] Hooijer, R.; Weis, A.; Kaiser, W.; Biewald, A. ; Dörflinger, P. ; Maheu, C.; Arsatiants, O.; Helminger, D.; Dyakonov, V.; Hartschuh, A.; Mosconi, E.; De Angelis, F.; Bein, T. Cu/Ag–Sb–I Rudorffite Thin Films for Photovoltaic Applications. Chem. Mater. 2023, 35, 23, 9988–10000.
[2] Weis, A.; Ganswindt, P.; Kaiser, W.; Illner, H.; Maheu, C.; Glück, N.; Dörflinger, P.; Armer, M.; Dyakonov, V.; Hofmann, J.P.; Mosconi, E.; De Angelis, F.; Bein, T. Heterovalent Tin Alloying in Layered MA3Sb2I9 Thin Films: Assessing the Origin of Enhanced Absorption and Self-Stabilizing Charge States. J. Phys. Chem. C 2022, 126, 49, 21040–21049.
[3] Hooijer, R.; Wang, S.; Biewald, A.; Eckel, C.; Righetto, M.; Chen, M.; Xu, Z.; Blätte, D.; Han, D.; Ebert, H.; Herz, L.M.; Weitz, R.T.; Hartschuh, A.; Bein, T. Overcoming Intrinsic Quantum Confinement and Ultrafast Self-Trapping in Ag–Bi–I- and Cu–Bi–I-Based 2D Double Perovskites through Electroactive Cations. J. Am. Chem. Soc. 2024, 146, 39, 26694–26706.
2.2-I3
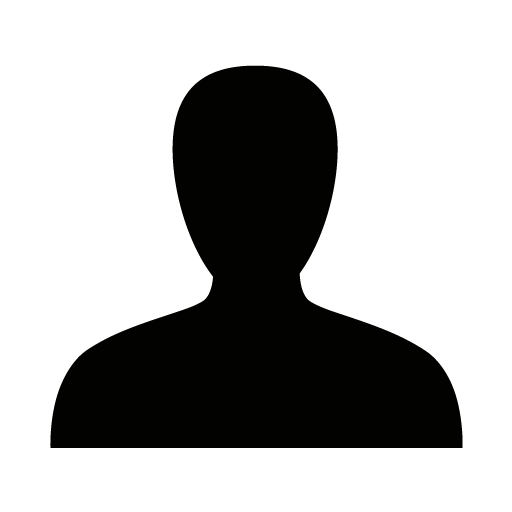
Solar cells based on nanocrystals have seen increasing interest in recent years due to the continuous rise in their power conversion efficiency. The most efficient cells are often based on nanocrystals that contain lead, such as lead sulfide (PbS) or metal halide perovskites (e.g., FAPbI3 or CsPbI3). While these nanocrystals lead to high efficiencies, they raise concerns regarding their large-scale applicability due to the environmental hazards they pose. In this talk, I will discuss the synthesis and application in solar cells of lead-free nanocrystals, such as those based on bismuth and antimony. I will present a method to synthesize an array of compositions by cation exchange, a simple, low-temperature process. Finally, I will introduce a facile templated growth of small antimony sulfide (Sb2S3) nanorods that are of great interest for application in photovoltaics.
2.2-O1
Researcher (Staff scientist), Lab Engineer, and Teacher at the Department of Chemistry at the Ben-Gurion University of the Negev, Israel.
Inorganic semiconductor photoabsorbers such as colloidal nanocrystals are frequently employed for a wide variety of energy-related applications by themselves or as part of a hybrid nanostructure,[1] including photocatalysis and photovoltaic devices. However, using them as the photoactive layer in photoelectrochemical (PEC) is less common due to the difficulty in binder-free attachment to transparent conductive oxide (TCO) substrates. On the other hand, polymeric carbon nitrides(CNs) are a family of cheap and highly stable semiconductor materials that can be in situ grown on TCOs. Unfortunately, their successful utilization for PEC has yet to reach the performance of state-of-the-art metal-oxide-based systems, among others, due to insufficient light harvesting and inferior conductivity.[2,3]
In this talk, I present a simple method based on electrophoretic deposition of ZnSe nanocrystals into a porous modified CN layer as the scaffold to form an efficient hybrid photoactive layer over TCO.4 The merits of this simple yet scalable solution processing method will be discussed, including the crucial step of stripping long-chain alkyl surfactant from the nanocrystals. The resulting hybrid structure achieves an impressive Faradaic efficiency towards the oxygen evolution reaction at ca. 87% and doubles the measured photocurrent and IPCE values relative to samples without ZnSe. Our results show the benefit of such a combination in terms of charge separation, stability, and successful water-splitting without using additional co-catalysts—evolving oxygen while minimizing parasitic self-oxidation.[4] This method paves the way for the incorporation of other nanocrystals into porous organic hosts.
1.1-O2
Juan Bisquert (pHD Universitat de València, 1991) is a Professor of applied physics at Universitat Jaume I de Castelló, Spain. He is the director of the Institute of Advanced Materials at UJI. He authored 360 peer reviewed papers, and a series of books including . Physics of Solar Cells: Perovskites, Organics, and Photovoltaics Fundamentals (CRC Press). His h-index 95, and is currently a Senior Editor of the Journal of Physical Chemistry Letters. He conducts experimental and theoretical research on materials and devices for production and storage of clean energies. His main topics of interest are materials and processes in perovskite solar cells and solar fuel production. He has developed the application of measurement techniques and physical modeling of nanostructured energy devices, that relate the device operation with the elementary steps that take place at the nanoscale dimension: charge transfer, carrier transport, chemical reaction, etc., especially in the field of impedance spectroscopy, as well as general device models. He has been distinguished in the 2014-2019 list of ISI Highly Cited Researchers.
The study of perovskite solar cells degradation is a complex issue due to the multitude of phenomena that can contribute to it. Normally the degradation can produce two main impacts, decrease of charge collection (lowering photocurrent) or increase of recombination (lowering photovoltage). We need to find dynamical signatures of the phenomena causing these effects to discover the physical reasons for the devaluated performance. Recently, we have obtained new insights using a model that combines several electronic and ionic processes, that can produce capacitive and inductive response in different circumstances. These models are very successful to describe huge memory effects and hysteresis in perovskite memristors, by the combination of different techniques: current-voltage scan, time transients, and impedance spectroscopy. Here we show the changes of impedance spectroscopy and time transients as a diagnosis of evolution of degradation in the perovskite solar cells. This analysis expands the tools available for understanding the transformation of perovskite solar cells under working conditions.
1.1-I2
Electrochemical impedance spectroscopy measurements of perovskite solar cells (PSCs) show characteristic features at low frequencies, such as a large illumination-dependent capacitance [1,2]. While this effect is well known, the debate on its origin persists [3,4]. An illumination-dependent increase in the conductivity of ionic charge carriers was suggested early on [2]. However, experiments to elucidate the presence of such a photo-conductive effect require special devices or measurement techniques and neglect possible influences of the enhanced electronic charge concentrations. Thus, only a few studies investigated this effect in detail.
Using drift-diffusion simulations and two novel techniques to analyze the simulation results, we show how the illumination-dependent part of the capacitance originates from electronic currents that are amplified due to the screening of the alternating electric field by the ions [5]. This is inherently caused by the mixed electronic-ionic interaction. Counter-intuitively, an illumination-dependent ion conductivity even reduces the magnitude of the capacitance increase.
As the presence and magnitude of a low-frequency capacitance increase by itself are unsuited to assess the presence of illumination-dependent ion conductivity, we propose a novel characterization technique based solely on capacitance measurements at short-circuit on fully integrated devices. The frequency shift of the onset in capacitance is extracted at varying illumination intensity. This quantity shows a distinct qualitative difference depending on whether the ion conductivity depends on illumination or is constant. As these measurements can be performed on unaltered, fully integrated devices and with standard equipment, the method is well suited for widespread investigation of a photo-conductive effect in different materials and devices or in response to degradation.
The method is applied to a range of perovskite solar cells with different active layer materials. Remarkably, all measured samples show a clear signature of photoenhanced ion conductivity, posing fundamental questions on the underlying nature of the photosensitive mechanism.
1.1-O1
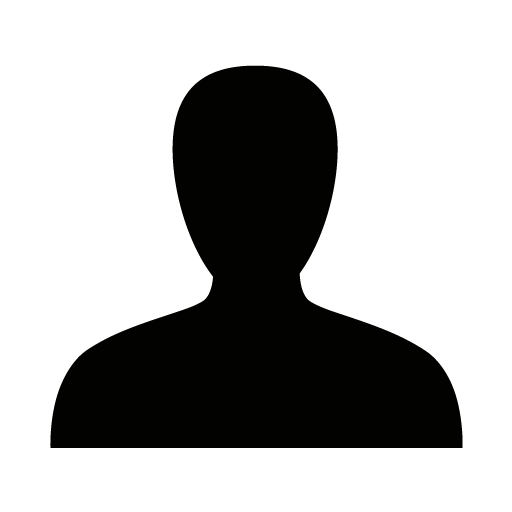
Semitransparent perovskite solar cells are emerging as a promising technology for applications such as energy-generating windows and integrated photovoltaics, where a balance between efficiency, transparency, and aesthetics is essential. [1-5] Designing such devices demands a deep understanding of light absorption, reflection, and transmission within the cell structure. In this work, we outline a simulation-based methodology for optimizing these devices, beginning with the calculation of optical constants for each layer in the cell, which is key not only for understanding the limitations associated to different devices, but also for accurately predicting the best design for each application. [6-7] We show the importance of validating the accuracy of the optical model by comparing simulated results with experimental data of full devices, performing global fits of different devices which ensures the model reliability. Finally, we explore various optimization strategies aimed at achieving specific performance targets, such as maximizing power conversion efficiency while maintaining desired levels of transparency and color rendering properties. By integrating accurate material characterization, rigorous simulation validation, and strategic optimization, this approach accelerates the development of high-performance, semitransparent perovskite solar cells tailored for diverse applications. This framework offers a practical pathway for bridging the gap between fundamental research and scalable device design.
1.1-I1
In this talk we set out to explain the physics that gives rise to two surprising phenomena that are frequently observed in perovskite solar cells, namely inverted hysteresis (IH) and the appearance of a third (intermediate frequency) feature in PSC impedance plots. Both these phenomena are caused by the leakage of charge carriers from one of the transport layers into the perovskite in sufficient numbers that they are able to partially screen electric fields in the interior of the perovskite layer. In order to better understand these phenomena, we analyze the standard drift-diffusion model of a planar three-layer PSC, using asymptotic techniques, to derive a reduced order model capable of describing the screening effects in the perovskite layers arising both from the presence of ions (which redistribute slowly) and from that of charge carriers (which adjust almost instantaneously). This approximate reduced order model shows excellent agreement with numerical simulations to the full drift-diffusion model and provides fundamental insights into the causes of inverted hysteresis reconciling the alternative explanations of this phenomenon found in the literature. Furthermore it can be used to explain the appearance of the third (intermediate frequency) feature in PSC impedance plots. Understanding why these atypical phenoneman occur is important for the device physicist because their appearance can be used to diagnose certain properties of the cell.
1.2-I1
In the last 10+ years of development, perovskite solar cells have achieved excellent efficiencies and a more gradual improvement in stability. Perovskite materials for photovoltaics are mixed electronic-ionic conductors [1]. It is therefore essential to consider the density and mobility of ionic defects in continuum-level models of perovskite-based devices, including tandem cells. Ionic defects impact both the steady-state and dynamic behaviour of perovskite cells [2] by modulating the electric field and charge carrier recombination rates.
An initial density of ionic defects is formed during cell fabrication; however studies suggest that additional defects can form during operation, under bias and illumination. Accumulation of mobile ion defects at the perovskite/transport layer interfaces results in undesirable degradation and performance loss over a timescale of hundreds of hours [3].
Improved modelling and simulation is required to understand the process of defect generation and quantify its impact on device characteristics over relevant timescales. We extend the charge-transport model that underpins our open-source IonMonger tool [4] and perform simulations to investigate the impact of defect generation and migration on perovskite solar cell performance.
1.2-O1
Zinc Phosphide (Zn3P2) is a promising earth abundant absorber for photovoltaics, offering direct bandgap of (1.5 eV), high optical absorption coefficient in the visible range (104 - 105 cm-1) and long carrier diffusion length, making it ideal for thin-film solar cells.
Monocrystalline Zn3P2, grown via Selective Area Epitaxy (SAE) naturally forms textured films with periodic pyramid-shaped nanostructures [1], [2], [3]. The growth mechanism necessitates a SiO2 patterned substrate, where Zn3P2 can grow on selectively exposed area of the substrate.
These naturally occurring nanostructures can be controlled in height and periodicity, depending on the opening dimension, and offer potential light management benefits to minimize in-coupling and out-coupling losses. The patterned substrate is a consequence of the growth mechanism; however, it provides the advantages of shaping and reducing the p-n junction contact area with valuable prospects for enhanced carrier management.
In this study, we present comprehensive device modelling of textured Zn3P2-based solar cells using coupled 3D optical and electrical simulations, employing FDTD and a Schrödinger-Poisson drift-diffusion solver to optimize the solar cell design.
Optical simulations are used to study the optical phenomena occurring within this complex structure including Mie modes, Rayleigh anomalies, and Fabry-Perot resonances. These effects are analyzed as a function of the pyramid height, periodicity, and thickness of the thin film beneath the pyramid. By tuning these modes and their interactions through geometric adjustments, the photocurrent is optimized to approach up to 89% of the Lambertian limit.
Electrical simulations are employed to study the effect of the reduced p-n junction contact area between Zn3P2 and the substrate. The junction area fraction was varied from 100% (continuous interface) down to 1.4%. Analysis of the simulated JV curves under both illumination and dark conditions highlighted the benefits of reducing the junction area fraction, for enhancing the open-circuit voltage (Voc) up to 0.12V.
This optical-electrical model exploits the potential for Zn3P2-based solar cells grown by SAE combining advanced light management and optimized junction design to enhance performance.
1.2-I2
We present a tour through the many and varied impedance spectra observed in perovskite solar cells, including loops, mid-frequency features, and the so-called ‘giant’ and ‘negative’ capacitances. Beginning with single-arc spectra, progressing through double- and triple-arcs, and finishing with discussion of the effects of degradation, we classify observed spectra into generic types, named for animals resembling their Nyquist plot. Remarkably, all of these spectral ‘animals’ can be faithfully replicated using the well-established ionic-electronic drift-diffusion model with a single mobile ion species, eliminating the need for speculative physics. Perovskite solar cell spectra often defy traditional interpretations, prompting increasingly intricate equivalent circuit models comprising elements without a sensible physical meaning. However, our animal-inspired framework offers a simpler, more intuitive approach to spectral analysis. This ‘spotter’s guide’ allows researchers to identify spectral features and their underlying physical origins based only shape recognition from a safe distance, unlocking insights without the need to venture into the wilds of computational modelling.
1.2-I3
Ion migration lies at the heart of perovskite solar cell (PSC) performance and stability. Different experimental techniques have been used to better understand the puzzling performance of ion migration in PSCs. In this talk, I will present our last results on the characterization of ion migration by two characterization measurements, X-Ray Photoemission Spectroscopy (XPS) [1] and impedance spectroscopy (IS) measurements [2] interpreted by drift-diffusion (DD) simulations.
For the characterization of the electronic and chemical properties of halide perovskites surface and interfaces to adjacent layers, XPS is a versatile technique. We use a lateral microstructure in which two different charge transport layers in co-planar contact configuration are separated by a perovskite channel constituting a lateral solar cell [1]. We apply reverse and forward bias with typical PSC operational conditions to the lateral microstructure to analyze the performance of the PSCs focusing on the evolution of band bending.
On the other hand, Impedance Spectroscopy is a consolidated tool to analyze the opto-electronic response of PSCs. Lately, it has been proved the proof of concept of the coupling of DD simulations with IS for a better understanding of device degradation [2]. I will present our last results on numerical DD simulations, and in particular, the study case of NiOx-based PSCs with various interface passivation treatments [2]. Our simulations approach several experimental measurements of IS under short-circuit conditions at different illumination intensities, along with bias-stress accelerated operational stability tests under constant illumination. Drift-diffusion simulations suggest that interface modification with the hole transport material may modify ion mobility within the perovskite layer. Our findings provide a systematic approach for characterizing instability mechanisms in PSCs using IS under short-circuit conditions.
1.3-I1
Dye-sensitized solar cells (DSSCs) are promising for glazing applications due to their potential for semi-transparency. However, their photovoltaic performance and light transmittance are largely determined by the dye and electrolyte used, both of which are fixed during the manufacturing process. Electrolytes are critical to energy technologies, yet their optimization is challenging due to the complexity of their formulations and the multitude of interacting chemical components. Typically, optimization requires numerous experiments, as the effects of these components are often correlated and difficult to analyze independently.
In this study, we employed a design of experiments (DoE) methodology combined with machine learning (ML) to design electrolytes that effectively balance two typically conflicting properties: visible transparency and power conversion efficiency (PCE). The model required only a limited number of experiments for training and exhibited excellent predictive agreement with experimental results.
First, we optimized iodine-based electrolytes to fabricate solar cells with a visible transparency range of 34% and a maximum PCE of 2.94%. We then extended this approach to electrolytes based on alternative redox systems. Using our data-driven modeling approach, we optimized a TEMPO-based electrolyte, achieving photochromic semi-transparent cells with a 42% transmittance variation and a PCE of 2.16%. For opaque cells, this novel electrolyte delivered a PCE of 3.46% with a photochromic dye and an impressive 7.64% PCE when paired with a non-photochromic dye.
1.3-O1
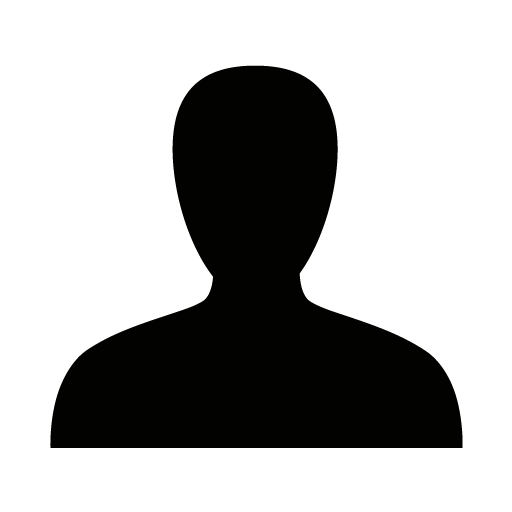
An efficient way to substantially increase the surface area coverage of photovoltaic (PV) modules, while maintaining the existent electricity infrastructure, is to integrate such modules into the roofs and facades of buildings. Aside from the practical technical requirements, such as high power conversion efficiency (PCE), low cost, and long lifetime, photovoltaic modules for building applications also necessitate an aesthetically attractive design, which can be achieved by finetuning their color according to the specific architectural needs of the building [1,2].
For some PV technologies, intrinsic coloration with a limited selection of colors can be attained by selecting absorbing materials with specific spectral absorption behavior [3,4]. To achieve a broader range of colors typically requires the addition of colored encapsulants, printed glass covers or interlayers in front of the PV module. Most commonly, pigments or chemical colorants are employed to accomplish such coloration, but they tend to absorb a significant portion of the solar spectrum, drastically reducing the PCE of the resulting PV module. A promising alternative to overcome such issues is to take advantage of the interference effects between non-absorbing dielectric materials with contrasting refractive indexes to design PV modules with vivid structural colors and low optical losses [5,6].
Periodic distributed Bragg reflectors (DBRs) have been considered in some previous works to realize structural coloration in PV modules [7,8], but they typically fail to reproduce some colors, especially reds, owing to the appearance of higher-order interference peaks in the reflectance spectra. To reach a broader color gamut thus requires breaking up the periodicity inherent to DBRs in a controlled way, such that the optical response of the PV module is tuned to achieve the desired coloration. In the present work, a numerical approach combining the electromagnetic description of light propagation together with the use of optimization algorithms is considered to optimize the configuration of a dielectric multilayer structure deposited directly on top of a polymer foil interlayer that is placed in between the substrate and the PV cell, targeting different structural colors for the PV module. As it will be demonstrated, the non-trivial aperiodic structures obtained from this method cover a broader color gamut than the simpler DBRs, and are key to achieve different red hues, including the ones of commonly used raw construction materials, such as clay or brick. As a proof-of-concept, a mini-module with a selected color is assembled by depositing an aperiodic multilayer structure with a numerically optimized configuration on top of a polymeric foil, using a roll-to-roll physical vapor deposition method. This allows not only to validate the numerical predictions regarding the structural color achieved, but also to demonstrate the low photovoltaic loss associated with this sort of multilayers and the scalability of the processes used to fabricate such colored modules.
1.3-I2
Extracting relevant material properties from experimental measurement is challenging, especially in the field of organic semiconductors. The models used to fit and reproduce experimental results are complex with multiple correlated parameters, which render the use of such model to extract relevant material properties very complicated. To overcome such limitations, we consider in this work the use of Bayesian inference for parameter estimation. Bayesian inference is a powerful tool to extract parameters distribution considering the experimental observations and the models considered [1].
In this study, we apply Bayesian inference techniques to analyze temperature-dependent photoluminescence spectra obtained from organic solar cells. We model the photoluminescence spectra of organic semiconductor films using a semi-classical marcus-levich-jortner expression [2, 3]. We model the spectra under different temperature and reproduce the change in spectral shape and relative intensity. Using the model and a Bayesian inference approach, we extract distributions for the different relevant properties of interest such as: Energy of the first excited state, the static disorder in energy, the reorganization energies (low and high frequency) as well as the dominant frequency mode. Our approach provides robust parameter estimation and quantifies uncertainties, enabling more accurate characterization of organic semiconductor materials. The results demonstrate the effectiveness of Bayesian inference in unraveling complex material properties and guiding future research in renewable energy applications.
1.3-I3
Organic light-emitting diodes have been successfully commercialized by the display industry, yet there are still basic challenges in modeling their operation and degradation. In this talk, I will highly recent work establishing the thermodynamic limit of OLEDs, which shows that strong exciton binding in these devices requires a higher voltage to achieve the same luminance as a comparable inorganic LED, and that the best OLEDs reported to date have likely reached this limit. I will discuss how the well-known Shockley-Read-Hall (SRH) expression for trap-mediated recombination in OLEDs is modified to account for the finite lifetime of dopant excitons and its implication for minimizing OLED drive voltage. Finally, I will discuss recent work focused on understanding blue OLED degradation where exciton-polaron-based degradation kinetics are implemented into a drift-diffusion-based device model. The results suggest that OLED luminance loss and voltage rise largely originate from different sets of degradation-induced defect states formed in the emissive and transport layers, respectively, which opens up new opportunities to optimize the performance and lifetime of these devices.
1.1-I1
On of the most fascinating properties of tetrahedrally coordinated solids is their negative thermal expansion. For Si, Ge and a number of binary compounds (cubic materials crystallizing in the diamond-type and sphalerite- or wurtzite-type crystal structure) it was shown that their thermal expansion coefficient becomes negative at temperatures between 50 to 100 K [1-3]. In ternary AIBIIIX2VI chalcogenides, crystallizing in the tetragonal chalcopyrite-type structure, the linear thermal expansion behaviour is described by the both independent linear thermal expansion coefficients αa and αc, which are anisotropic. It was shown that CuBX2 chalcopyrites (B=In,Ga) exhibit negative linear thermal expansion at temperatures below 30 K, for both a and c. The lattice parameter in these compounds first decrease with decreasing temperature going through a minimum and increase at low temperatures [4-8]. In the exceptional case of AgBX2 semiconductors the lattice parameter c increases with decreasing temperature in the whole temperature range [9].
Because of negative linear thermal expansion coefficients αa and αc, negative Grueneisen parameters may be expected in the low-temperature region and as a consequence the existence of low-energy lattice vibrational modes [10]. Moreover high-pressure induced structural phase transitions are caused by low-energy lattice vibrational modes with negative Grueneisen parameters. It is known from literature that the binary compounds ZnS and ZnSe as well as ternary CuInSe2 show a pressure induced structural phase transition to the rocksalt-type structure [11-12].
Not much is known about the low temperature behaviour of quaternary chalcogenide compound semiconductors, like A2IBIICIVX4VI. According to the tetrahedrally coordinated crystal structure, a negative thermal expansion can be expected. This assumption is strenghtened by the observation of a pressure induced structural phase transition in Cu2ZnSnS4 [13]. This compound shows a transition from the tetragonal kesterite-type structure to ta distorted rocksalt-type structure at ~ 15 GPa [13].
The compound semiconductors discussed above are used as absorber layers in thin film solar cells. Photovoltaic (PV) devices with chalcopyrite-type Cu(In,Ga)Se2 absorbers show very high efficiencies [14]. The quaternary semiconductors Cu2ZnSn(S,Se)4, crystallizing in the tetragonal kesterite-type structure, are the absorbers in the only critical raw material free PV technology. Record efficiencies have been reached with an (Ag,Cu)2ZnSnSe4 absorber layer [15].
Thin film solar cells are the most ubiquitous and reliable energy generation systems for aerospace applications, because of their appealing properties such as lightweightness, flexibility, cost-effective manufacturing, and exceptional radiation resistance [16]. For longer missions, PV devices in conjunction with rechargeable batteries are the only available option to provide uninterrupted, sustainable and stable electrical power. Especially satellites on inner planets missions employ solar cells, because at these distances the power density of sunlight is sufficient for the production of electricity [16]. For these applications the harsh conditions in space, like radiation and low temperatures, have to be taken into account. The temperature in outer space far away from earth is just 3 K [17], and extreme temperature swings occur. Thus the low temperature behaviour of absorber materials in solar cells potentially used in space applications, are of extreme importance.
The presentation will give an overview of our detailed in situ neutron diffraction based structural investigation comparing the thermal expansion behaviour of Cu2ZnSnSe4, Ag2ZnSnSe4 and (Ag,Cu)2ZnSnSe4 mixed crystals from room temperature to 3 K. These materials crystallize in the tetragonal kesterite-type structure in the whole temperature range studied. The linear thermal expansion coefficients αa and αc are highly anisotropic. The end member Cu2ZnSnSe4 shows negative thermal expansion coefficients below 50K. In case of Ag2ZnSnSe4 the lattice parameter c increases with decreasing temperature thus showing a negative thermal expansion coefficient over the whole temperature range studied, indicating the special behaviour of Ag-containing tetrahedrally coordinated semiconductors.
1.1-I2
Dr. Edgardo Saucedo studied Chemical Engineering at the University of the Republic, Montevideo, Uruguay, and received his PhD in Materials Physic at the Universidad Autónoma de Madrid, Madrid, Spain in 2007 with a FPU fellowship. In 2007, he joined the Institut de Recherche et Développement sur l’Énergie Photovoltaïque IRDEP (Paris, France), with a CNRS associated Researcher fellowship, working in the development and optoelectronic characterization of CIGS low cost based solar cells. In 2009, he joined NEXCIS, a spin-off created from IRDEP, to further pursue their training in photovoltaic technology. In 2010, he joined the Solar Energy Materials and SystemsGroup at the Catalonia Institute for Energy Research (IREC) under a Juan de la Cierva Fellowship first (2010-2011) and a Ramon y Cajal Fellowship afterwards (2012-2016), with the aim to develop new low cost materials and processes for thin film photovoltaic devices. In 2020 he joined the Polytechnic University of Catalonia (UPC) to continuous his scientific and professorhip career.
He holds five patents and has authored or co-authored more than 215 papers in recognized international journals, including: Energy and Environmental Science, Advanced Materials, Adv. Energy Materials, Journal of the American Chemical Society, Chemistry of Materials, Progress in Photovoltaics: Research and Applications, Solar Energy Materials and Solar Cells, NanoEnergy, J. Mater. Chem. A, J. Phys. Chem. C, etc. He has more than 350 contributions to the most important Congresses in Physics, Chemistry and Materials, and more than 35 invited talks around the world. He has been involved in more than 25 European and Spanish Projects (Scalenano, Inducis, Pvicokest, KestPV, Larcis, etc.), and he was the Coordinator of the ITN Marie Curie network Kestcell (www.kestcells.eu), the research and innovation project STARCELL (www.starcell.eu), and the RISE project INFINITE-CELL (www.infinite-cell.eu), three of the most important initiatives in Europe for the development of Kesterites. In 2019 he was granted with an ERC-Consolidator Grant by the European Research Council (SENSATE, 866018, 2020-2025), for the development of low dimensional materials for solar harvesting applications to be developed at UPC. Currently he is also the scientific coordinator of the European project SUSTOM-ART (952982), for the industrialization of kesterite for BIPV/PIPV applications.
He is frequently chairman and invited speakers in the most relevant Conferences in Photovoltaic (E-MRS, MRS, IEEE-PVSC, EUPVSEC, European Kesterite Workshop, etc.). He has supervised 11 PhD Thesis and is currently supervising 5 more. He has an h factor of 38 and more than 5000 citations. In 2020 he has been awarded with the ASEVA-Toyota Award for his contribution to the development of sustainable photovoltaic technologies using vacuum techniques (https://aseva.es/resolucion-de-los-primeros-premios-nacionales-de-ciencia-y-tecnologia-de-vacio-aseva-toyota/).
The synthesis of multinary semiconductors for solar energy conversion applications such as kesterite (Cu2ZnSn(S,Se)4, CZTSSe) is extremely challenging due to the complexity of this type of compounds. In particular, quaternary kesterite-type compounds are not the exception, and all these detrimental issues explain why during almost 10 years the world record efficiency was unchanged. But the very recent development of molecular inks route with special precursors, allows the accurate control of single kesterite phase with high crystalline quality, contributing to increase the conversion e"ciency record of kesterite based solar cells up to 15% in a short time.
This presentation will be focused first in demonstrating how the molecular inks synthesis route was of key relevance for the control of high-quality single phase kesterite, through the modification of the synthesis mechanisms. The relevance of the composition of the ink, the precursor salts, and the interaction between the solvent and the cations in the solution is key for a reliable and reproducible high efficiency kesterite production baseline. Then, diluted alloying/doping strategies will be presented including Cu, Zn and Sn partial substitution with elements such as Ag, Li, Cd or Ge, that allowed e"ciencies close to 15%. The positive impact of these cation substitutions will be discussed in regards of their impact on the kesterite quality, as well as on the annihilation of detrimental punctual defects, allowing for new efficiency records at 15% level. In addition, the main characteristics and challenges of key kesterite interfaces (front, back and grain boundaries) will be discussed. Finally, very recent, and innovative interface passivation strategies will be discussed, showing the pathway to increase the record efficiency beyond 20%.
1.1-O1
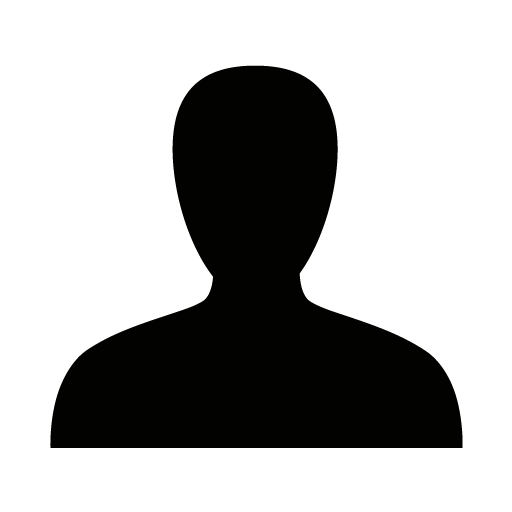
With the motivation of absorber layers which contain Earth-abundant, cheap and low-toxicity elements, the material Cu2ZnSn(S,Se)4 (CZTSe) has been a popular choice in the inorganic thin-film photovoltaics (PV) research community. After an extended period with no improvements to efficiency, recent records of ~13.8% [1] suggest a possible resurgence in research. Here we explore quantifying the environmental impacts of fabricating a CZTSe solar cell at the laboratory-scale using a mix of vacuum techniques (sputtering, electron beam evaporation, tube furnace annealing) and non-vacuum techniques (CZTS nanocrystal synthesis, slot-die coating, chemical bath deposition) with a structure of glass/Mo/CZTSe/CdS/i-ZnO/ITO/Ag,Ni. The intention is to determine which processes or layers are the most significant on the overall environmental impact of the fabrication and hence which may inhibit future scale-up. A life cycle assessment (LCA) is conducted which includes the materials used, electricity consumed as well as the waste produced. Previously we have used LCA to show how to reduce environmental impacts during the synthesis of CZTS nanocrystals [2]. We now extend the LCA analysis to the whole device. The selenization step (where selenium substitutes for sulfur), whilst essential for improved device performance, contributes significantly to the overall environmental impacts of cell fabrication. This results in the impacts from the solution-processed absorber layer to be similar to the vacuum-deposited transparent conducting oxide (TCO) layer due to the necessity of a high temperature, low pressure anneal. This work is hence a timely contribution to discussions surrounding the environmental impacts of vacuum versus non-vacuum deposition. In future work, a functional unit of 1 kWh is chosen such that device performance is included. This will allow for comparison to other research-scale solar cells with absorber layers such as Sb2(S,Se)3 and BaZrS3.
1.1-O2
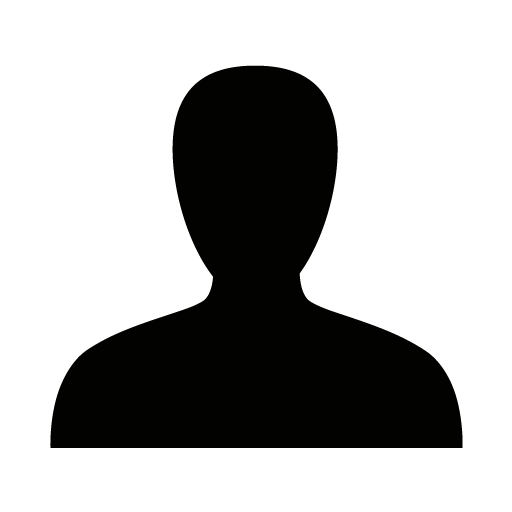
A new power conversion efficiency record of 15.1% was reported just recently for a CZTSSe-based thin film device in which the polycrystalline CZTSSe absorber layer shows an off-stoichiometric composition. Deviations from stoichiometry cause intrinsic point defects which determine the electronic properties of a semiconductor significantly. A special kind of structural disorder, the Cu/Zn disorder, is always present in these compounds and is discussed as a possible reason for band tailing as well.
To minimize or avoid Cu/Zn disorder cation mutation strategies can be applied. In this way the crystal structure of the material can change from kesterite- to stannite-type to completely avoid this disorder. Substituting Zn2+ with Cd2+ in CZTS is one of the options. In the resulting solid solution series, both end members adopt different crystal structures: Cu2ZnSnS4 crystallizes in the kesterite-type structure whereas Cu2CdSnS4 adopts the stannite-type crystal structure.
We studied crystal structure, cation distribution and intrinsic point defect scenario in Cu2(Zn1-xCdx)SnS4 monograins by neutron diffraction. This method enables us to differentiate the isoelectronic cations Cu+ and Zn2+ in the crystal structure analysis. At the same time the presence of Cd2+ in the samples introduces a huge challenge for neutron diffraction, as Cd is absorbing neutrons, in this way increasing the measuring times significantly.
These investigations enabled us to deduce that in the range between x=0 and 0.38 the mixed crystals adopt kesterite type structure with increasing Cu/II disorder with increasing Cd content. Starting with x=0.57 and until x=1.0 the material adopt stannite type structure, with a complete absence of Cu/II disorder. The change in the cation distribution being so abrupt suggests us that in this solid solution the complex cation re-distribution process within the crystal structure is happening in a very narrow compositional range 0.38 < x < 0.57.
1.2-I1
Chalcogenide-based solar cells are regarded as promising due to their use of abundant, low-toxicity materials
and their scalability for cost-efficient manufacturing. However, their performance is often constrained by
efficiency challenges, with non-radiative recombination identified as a significant limitation. The potential
to overcome these challenges is seen in the exploration of emerging chalcogenides, offering pathways toward
improved stability and higher efficiencies in next-generation photovoltaic devices. A Voc deficit, largely
attributed to non-radiative recombination, has been identified in prior analyses as a major obstacle for these
materials. In this presentation, findings will be extended to recently reported high-efficiency devices, with
key photovoltaic metrics compared to historical benchmarks, and future research directions proposed. The
influence of Zn substitutions in CXTS (where X includes Mn, Mg, Sr, Ba, Ni, Co, Fe) on structural,
optoelectronic, and photovoltaic properties will be discussed, with particular attention given to the role of Ag
and other cation substitutions in Cu₂CdSnS₄ photoabsorbers. Directional growth of high-mobility [hk1]
planes in Sb₂(S,Se)₃, which has enabled power conversion efficiencies exceeding 9%, will also be presented.
Lastly, the application of CZTS and Sb₂(S,Se)₃ as photocathodes in solar water splitting will be explored.
1.2-I2
The evolution of Sb2Se3 heterojunction devices away from CdS electron transport layers (ETL) to wide band gap metal oxide alternatives is a critical target in the development of this emerging photovoltaic material. Metal oxide ETL/Sb2Se3 device performance has historically been limited by relatively low fill-factors (FF), despite offering clear advantages with regards to photocurrent collection. In this work, TiO2 ETLs were fabricated via direct current (DC) reactive sputtering and tested in complete Sb2Se3 devices. A strong correlation between TiO2 ETL processing conditions and the Sb2Se3 solar cell device response under forward bias conditions was observed and optimised. Ultimately, a SnO2:F/TiO2/Sb2Se3/P3HT/Au device with the reactively sputtered TiO2 ETL delivers an 8.12% power conversion efficiency (η), the highest cadmium-free Sb2Se3 device reported to-date. This is achieved by a substantial reduction in series resistance (Rs), driven by improved crystallinity of the reactively sputtered anatase-TiO2 ETL, whilst maintaining almost maximum current collection for this device architecture. This paper will also discuss the role of organic hole transport materials ‐ namely P3HT, PCDTBT, and spiro‐OMeTAD to modify device performance. By comparing these against one another, and to a reference device, their role in the device stack are clarified. These organic HTM layers are found to serve a dual purpose, increasing both the average and peak efficiency by simultaneously blocking pinholes and improving the band alignment at the back contact.
1.2-O1
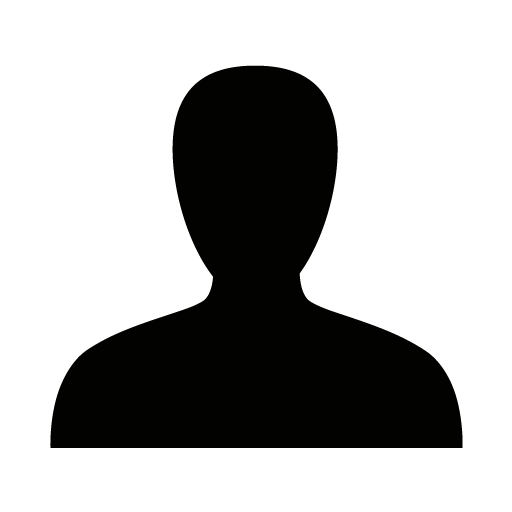
Antimony selenide (Sb2Se3) has emerged as a promising photoelectric material owing to its excellent material properties. The power conversion efficiency of Sb2Se3 thin-film solar cells has reached an impressive 10.57% within a decade. Despite rapid development, the efficiency of Sb2Se3 thin-film solar cells remains significantly below the theoretical prediction of 30%. Therefore, considerable efforts are still required to enhance the material quality of Sb2Se3, a critical factor in boosting solar cell efficiency. Post-deposition annealing treatments have been carried out as an effective method to improve the qualities of Sb2Se3 thin films, such as crystallinity and optical properties. However, these treatments typically require tens of minutes or more of thermal annealing at high temperatures (>300 °C), which severely limits both the throughput and substrate choice. For the first time, a low thermal budget annealing technique was used for post-deposition annealing of Sb2Se3, which was carried out to enhance the film properties of Sb2Se3 thin film solar cells. Photonic curing uses pulsed light annealing with a broadband light source and Sb2Se3 samples annealed with single pulse light in pulse lengths of 1 to 15 ms. This process heats the Sb2Se3 film above 400 °C within milliseconds without damaging the underlying layers of the material stack. Short pulses (less than 5 ms) having higher radiant power damage the Sb2Se3 thin films compared to longer pulses. In contrast, during longer pulses, the temperature rises quickly and decreases gradually, even while the light remains on. This is due to the power in the capacitor bank draining, which reduces the lamp’s output and limits the peak temperature at the sample surface. As a result, longer pulses cause less damage and lead to more crystalline films. Therefore, increasing pulse duration or reducing radiant exposure can minimise damage to the Sb2Se3 film. Photonic curing has increased the crystal orientations in the hkl, l ≠ 0 ([211] and ([221]) direction of Sb2Se3, reduced the surface roughness and decreased the leakage current of the solar cells. The reduced open circuit voltage effectively lowers recombination rates of charge carriers and improves carrier transport. These enhance the power conversion efficiency of Sb2Se3 by 46%. Hence, photonic curing shows the capability of curing Sb2Se3 thin films and creating high-quality Sb2Se3 thin films with high crystallinity without sacrificing surface coverage. This eliminates the rate-limiting annealing step and opens up new opportunities for Sb2Se3 photovoltaics.
1.2-O2
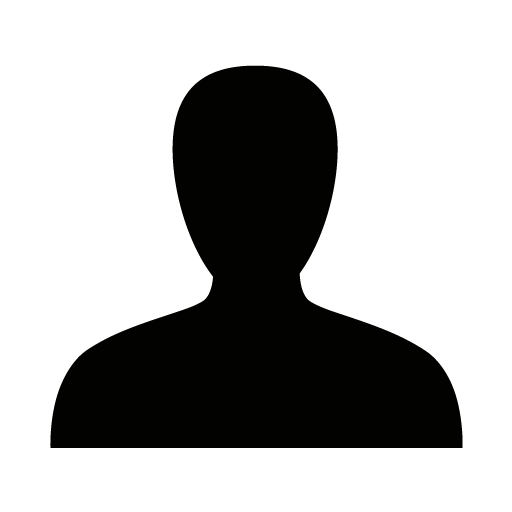
Low-dimensional antimony-chalcogenide materials have received an outstanding interest for photovoltaic (PV) devices in the last years. They show high stability, low environmental impact, low cost, low carbon footprint and high technological flexibility. Currently, efficiencies above 10% have already been achieved for Sb2(S,Se)3-based solar cells [1]. On the other hand, Sb-Ge chalcogenide material is well studied as phase change material for different applications, especially when using Te as chalcogen [2]. However, up to our knowledge, the combinations of Sb-Ge chalcogenide semiconductors have not been integrated in solar cell devices. In this work, Sb-(Ge)-Se thin films are grown by selenization of co-evaporated Sb-(Ge) films on Mo/SLG and SLG substrates. The properties of Sb-Ge-Se layers are compared with those of Sb2Se3 thin films. The effect of the selenization process on the structural, morphological, compositional and optical properties of the Sb-(Ge)-Se compounds is investigated. Different growth parameters, such as the maximum selenization temperature and Se added during the thermal treatment, have been investigated in these devices. Independently from the used growth parameters, all Sb2Se3 absorbers show an orthorhombic structure with [hk1] preferred orientation, and a compact structure free of pinholes, while the Sb-Ge-Se active layers show the co-existence of Sb2Se3 and GeSe2 phases. The co-existence of these two phases is corroborated by Fourier-transform infrared spectroscopy (FTIR). Sb-Ge-Se thin films´ band gap energy Eg varies from 1.4 to 1.8 eV depending on the Ge content, as determined by ellipsometry spectroscopy. First efficient Sb-Ge-Se thin-film solar cells have been fabricated using these new absorbers and device efficiencies of 5.4 % and 1.3 % are achieved for Sb2Se3 and Sb26Ge6Se68-based films respectively. C-V and DLCP measurements performed in both type of solar cells, indicate a high defects concentration when introducing Ge in the absorber layer, in agreement with the device performance. This is an indication that the (GeSe2)x(Sb2Se3)1-x system is more defective than the Sb2Se3 material. TEM investigation of Sb-Ge-Se and Sb2Se3 solar cells reveal a different CdS/Sb-(Ge)-Se heterojunction that can explain the limitation of the PV devices when Ge is incorporated in the structure.
More investigations are carried out to understand performance limitation in the solar cells based on these new thin-film absorbers. Finally, we will discuss the possible
improvements of the current devices and the potential of these promising and sustainable chalcogenide material for outdoor/indoor/thin film PV applications
1.3-I1
New materials of interest for photovoltaics or other applications are invariably multi-cation compounds or alloys. Therefore, to properly explore their properties, synthesis experiments must be carried out over a broad parameter defined by several compositional variables, core thermodynamic variables of temperature and pressure, and kinetic variables such as reaction time and heating rate. Each of these can affect the outcomes of synthesis: the phases formed and their microstructural and defect characteristics that are so important for functional properties. A special challenge in the context of inorganic materials such as multinary chalcogenides is that they can be grown over a very wide range of temperatures and pressures as well as off-stoichiometric compositions. Thus, the parameter space to be explored is particularly extensive.
In this contribution, we will present our concept for automated exploration of new inorganic chalcogenides in a self-driving lab. This combines a powerful and rapid PVD-based synthetic method with automation and machine learning, to rapidly map the phase space and functional properties of new materials, without needing prior information. The developments to-date will be presented, starting with our approach for automated generation of co-sputtering processes. This involves two machine-learning stages coupled to a geometrical model of the sputter flux, fitted in real time using input from a trio of QCM sensors. Outputs of the trained models can be used to produce co-sputtering recipes that yield a specified composition, while allowing other parameters (e.g. pressure) to vary. In addition, compositional maps for each sample are obtained directly without time-consuming mapping in an external system. We will preview coming developments in automation of the subsequent process stage – rapid thermal sulfurization – and some initial work in image-based high-throughput characterisation combined with simulations, to derive optical properties of the target materials. Perspectives for the application of self-driving labs in development of new device materials will be discussed.
1.3-O1
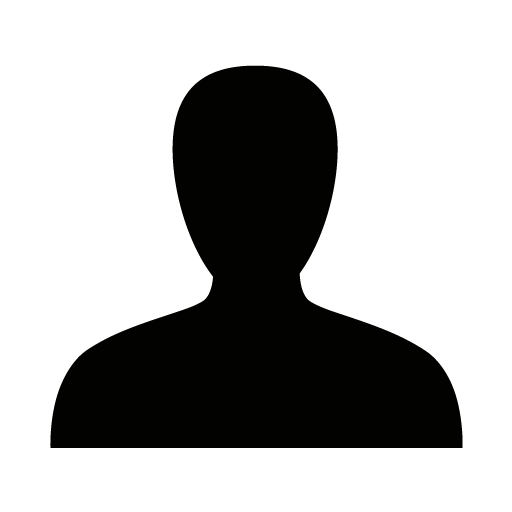
Chalcogenide perovskites, in particular BaZrS3, have gained a lot of popularity in the last few years due to its great potential as an alternative lead-free photovoltaic absorber material. This is due to promising optoelectronic properties such as defect tolerance, strong dielectric screening, and light absorption [1]. However some of the fundamental material physics, in particular polymorphic phase transitions, have not been explored in detail. Experimental studies have given conflicting results with Raman spectroscopy showing no signs of a phase transition[2], whilst XRD studies show an orthorhombic-to-tetragonal phase transition at 800K [3].
In this talk, we will introduce our machine learning potential model trained on perovskite structures with the neuroevolution potential method [4]. Through molecular dynamics calculations, we heat the experimentally reported orthorhombic Pnma phase and observe a first-order phase transition to a tetragonal I4/mcm phase at 610K. Upon further heating, we observe a second-order phase transition from the tetragonal phase to the cubic Pm-3m phase at 880K. We explain the order of these phase transitions through group-subgroup relationships and Landau theory.
Further analysis shows that the phase transitions are mediated through the M and R phonon modes associated with octahedral tilting, as is typically found in perovskite structures [5]. We analyze all possible Glazer tiltings to show that for the BaZrS3 perovskite only the Pnma --> I4/mcm --> Pm-3m phase transition route is accessible through heating. We also show temperature-dependent static structure factors and compare them to published experimental work[3]. To end, we highlight the dependence of stability of different polymorphs of the perovskite across various pressures and temperatures through a phase diagram.
1.3-O2
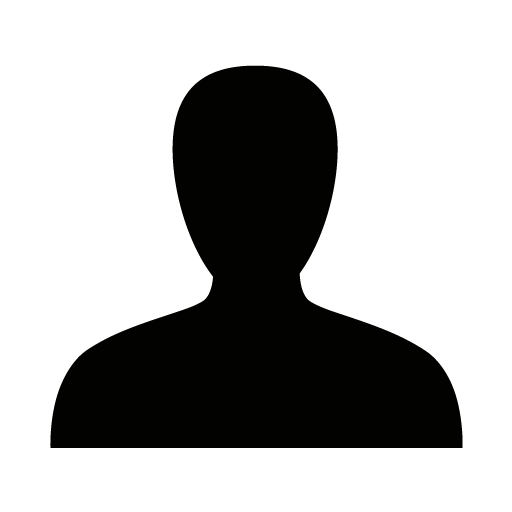
Barium sulphide (BaS) serves as a crucial precursor for advanced barium-based materials, including the emerging perovskite absorber BaZrS₃.[1] However, conventional BaS production methods are highly energy-intensive, requiring temperatures exceeding 1000 °C [2,3] and emitting large quantities of CO₂ and SO₂,[3,4] raising environmental concerns.
This work presents a novel solid-state synthesis route for BaS that drastically reduces the environmental and energy demands. By employing a finely milled mixture of barium hydroxide [Ba(OH)₂] and elemental sulphur, we achieve an efficient conversion (85%) to BaS at a remarkably low annealing temperature of 500°C.
The process is enabled by a unique low-pressure annealing environment, which facilitates the rapid vaporization of H₂O byproducts while maintaining a controlled sulphur partial pressure. This balance prevents unwanted side reactions and enhances the conversion efficiency. Furthermore, this method is highly scalable and compatible with industrial processes, offering a sustainable and economically viable pathway for BaS production.
1.3-O3
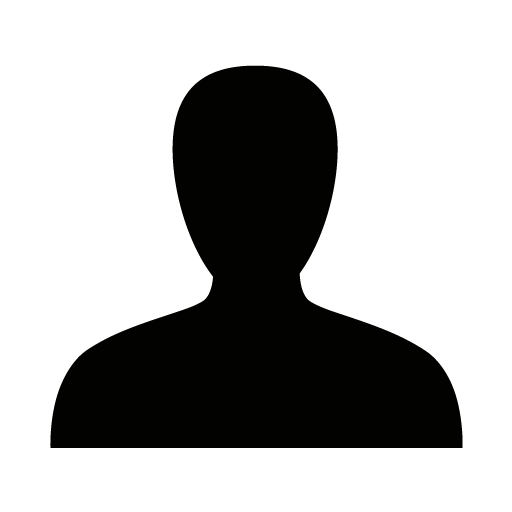
Ternary chalcogenide nanocrystals have emerged as a promising material in the field of renewable energy, particularly as absorber materials for solar cells. In recent years, there has been a notable focus on the development of environmentally friendly materials, such as AgBiS₂ and AgSbS₂, as an alternative to traditional quantum dots containing heavy metals, including lead and cadmium. The components of these materials are plentiful and less toxic, reflecting the growing emphasis on sustainability and green energy solutions. These materials display high absorption coefficients, tunable band gaps, efficient charge separation, and impressive stability, rendering them ideal for emerging applications in solar cells, photodetectors, photocatalysis, and thermoelectrics. [1-3]
Nevertheless, the synthesis of quantum dots has traditionally been performed using the hot injection method, which involves prolonged high-temperature and vacuum processes. This approach presents significant challenges in terms of scalability, cost, and reproducibility, which must be overcome for these materials to be suitable for commercial applications. In this study, we present a straightforward and low-temperature synthesis of AgBiS₂ and AgSbS₂ quantum dots via cation exchange. This study presents a novel approach to the synthesis of Ag2S nanoparticles (NPs) that employs unconventional sulfur precursors and a sequential exchange of silver ions for bismuth and antimony ions. This method allows the preparation of high-quality ternary quantum dots with precise control of size and atomic ratio. Furthermore, the incorporation of the ternary nanocrystals into photovoltaic devices provides evidence of the viability of the novel synthetic approach for the fabrication of high-performance solar cells.
2.1-I1
Despite its great potential, lead halide perovskite technology draws major scepticism from supporters of established optoelectronic technologies due to long-term stability and environmental compatibility issues. One of the suggested solutions in literature is the large family of non-conventional (other than CIGS and CdTe), defect-tolerant, non-toxic chalcogenide compounds which possess desirable optical band gaps in the visible range. However, the fabrication of highly-efficient photovoltaic devices is still challenging, as there is a clear gap in efficiency between metal halide and chalcogenide-based materials.
Here we present our efforts to fabricate thin films of acceptable optoelectronic properties and then build operational devices, where possible. We focus our experiments only on solution-processing methods, working on semiconductors at the nano- or poly-crystalline scale. We deal with compounds which have cubic crystal structure, such as AgBiS2, possessing 3D electron dimensionality and, thus, can work as effective absorbers in around 2% efficient photovoltaics. On the other hand, few of those materials can also present strong radiative recombination (PbS and CuInS2) and may act equally well as emitters in solar cells and LEDs. There are also cases where sulfides (or selenides) with lower dimensionality (such as Sb2S3) are adopted with very promising results (PCEs of over 5%). Additionally, we are also targeting at chalcogenide semiconductors which crystallize in the perovskite structure and are able to fabricate proof-of-concept liquid solar cells based on BaZrS3-modified TiO2 photoelectrodes [1]. Our ultimate goal is to replace Ba2+ with a di-protonated amine and fabricate, for the first time in literature, hybrid inorganic-organic chalcogenide perovskites.
2.1-O1
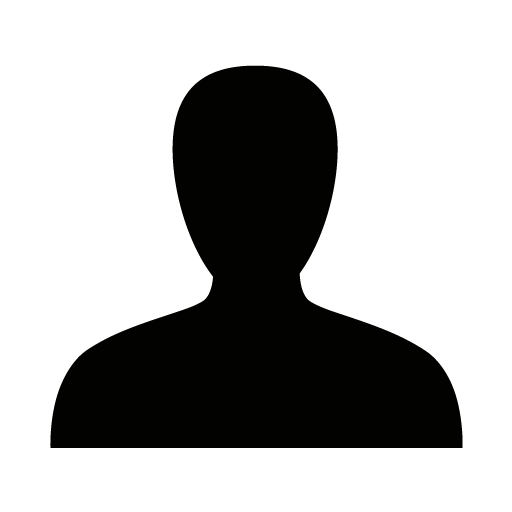
Bismuth sulfide (Bi2S3) is a nontoxic and inexpensive semiconductor that has potential for use in low-cost thin film photovoltaics owing to its near optimal 1.2eV bandgap and high absorption coefficients greater than 1x104cm-1 within the IR to UV range [1][2]. Although Bi2S3 has potential in photovoltaics, devices have performed poorly with typical efficiencies not surpassing 2% [3][4][5]. One of the challenges of creating high performance cells is from the bulk defects that can exist due to stoichiometric imbalances acting as effective recombination centres [6]. These defects such as the sulfur vacancy and interstitials act mostly as donors making p-type doping difficult [6][7]. The reported properties of Bi2S3 are also inconsistent and depend on how the film was prepared. Its lack of crystallinity for example can blue shift the band gap to as high as 1.8eV due to quantum confinement effects [9][4]. Carrier mobilities have varied greatly from 5 cm2V-1s-1 prepared by chemical bath to much higher values of 257 cm2V-1s-1 and 588 cm2V-1s-1 for rapid thermal evaporation and spray pyrolysis [9][4][10]. The latter tow is greater than the typical carrier mobilities reported of 21 cm2V-1s-1 to 50cm2V-1s-1 for monocrystalline Bi2S3 grown via the Bridgman technique, suggesting the importance of purity [12][11]. The choice of substrate can influence the charge transfer efficiency in photovoltaic devices due how the bands bend at the interface. Bi2S3 deposited onto fluorine doped tin oxide (FTO) for example exhibited better photocurrent than those on tin doped indium oxide (ITO), Molybdenum or Gold [8]. This research has embarked on a systematic study of Bi2S3 thin films for photovoltaic devices. These thin films were synthesised via sulfurization of sputtered bismuth films on FTO substrates within a tube furnace. By varying the holding temperatures, ramp rates and holding times different optical and structural properties can be obtained. The effect of different film properties such as crystallite size, stichometry and thickness will be tested in a ITO/ZnO/CdS/Bi2S3/FTO cell architecture. By gaining control over the film properties, they can be tuned to improve the performance as an absorbing layer in photovoltaic devices.
2.1-O2
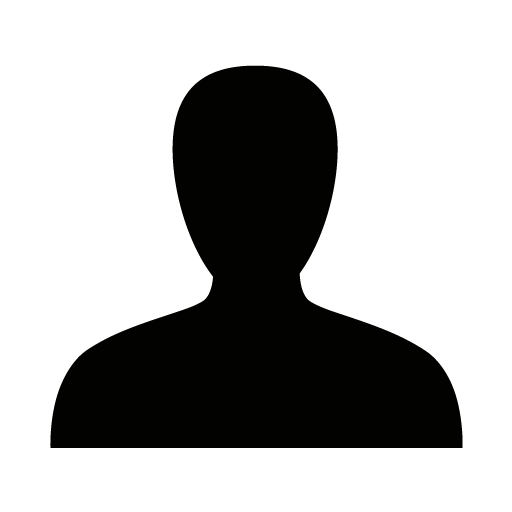
The currently demand for renewable energy sources, it is imperative to explore new configurations of solar cells that use multiple photosensitizers for enhanced efficiencies. Considering the aforementioned factors, the present study entails the fabrication of an innovative n/p/p heterojunction solar cell with a unique design, and it consists of an n/p-type photoanode made from TiO2/CdS/Cu2ZnSnS4(CZTS) and a p-type photocathode composed of Ag3SbS3/NiO/C/Ni foam, using a polysulfide gel electrolyte. This design captures light across the visible to near-infrared spectrum, achieving a high-power conversation efficiency (PCE) of 9.76%, significantly higher than standalone p-type (Ag3SbS3 ~1.6%) and n-type (CdS/CZTS, ~7%) solar cells. The alignment of various band gaps within the photoactive semiconductors, along with well-matched the energy levels at both photo-electrodes, effectively suppresses undesirable recombination process and enables efficient charge separation and transfer. An electrically conductive carbon interlayer, with a high work function of 5 eV, and it facilitates rapid and efficient electron transport from Ni to NiO. The p-type conduction of CZTS enables efficient hole extraction from CdS and their transfer to polysulfide species, while the narrow band gap of Ag3SbS3 quantum dots (~1.46 eV) facilitates broad-spectrum absorption, contributing to the high PCE. The n/p/p heterojunction solar cell also demonstrates impressive durability, retaining ~72% of its initial efficiency after 90 days of intermittent light exposure and storage. This resilience underscores its practical viability as a cost-effective, streamlined, and lead-free alternative to conventional perovskite, quantum dot, or Si/Ru-based solar cells.
1.1-I1
Halide perovskite semiconductors made a great impact on the field of solar cells with efficienies soaring beyond 26% [1]. On the other hand, halide perovskites are attractive materials for light emitting devices, i.e. LEDs and lasers. Perovskite lasers can be prepared from solution at low temperatures on a wide range of substrates, which provides exciting opportunities in the field of integrated optoelectronics.[2]
Amplified spontaneous emission (ASE) and optically driven lasing were achieved in so-called hybrid organic-inorganic lead halide perovskites, where the A-site cation is based on an organic compound, such as methylammonium or formamidinium. [3,4] Intriguingly, these crystalline compounds are soft and photonic nanostructures can be directly patterned into them by thermal imprint at moderate temperatures.[5]
All-inorganic halide perovskites, such as those where the A-site cation is Cs+, are frequently claimed to provide improved thermodynamic stability.[6] Among them, CsPbBr3 has been shown to be an excellent gain medium for perovskite lasers in the green spectral region. [7] More recently, we could show that b-CsPbI3 stabilized with 2.5wt% of PEO demonstrates a low lasing threshold of 45 μJ cm-2 at room temperature with tunable emission from 714.1 nm to 723.4 nm [8].
For the Cl-based representative, i.e. CsPbCl3, deposition of thin films from solution is essentially impossible due to the poor concomitant solubility of the precursor salts PbCl2 and CsCl.[9]
Here, we will show two concepts to achieve CsPbCl3 thin films as gain medium. As a first strategy, we use superlattice structures of PbCl2 and CsCl with a thickness of the sublayers on the order of 3-5 nm by thermal evaporation. We evidence the formation of CsPbCl3 at the interface of PbCl2 and CsCl. Already a 1 nm thick CsCl layer deposited on top of PbCl2 gives rise to a notable photoluminescence at 409 nm with a narrow line width of 8 nm (FWHM), which agrees with reports of CsPbCl3 single crystals. The superlattices show amplified spontaneous emission (ASE) in the deep blue spectral region at 427 nm above a threshold energy density of 190 µJ/cm2 at room temperature (RT).
In a second approach, we subject thin-films of CsPbBr3 to halide exchange. Upon pulsed exposure to TiCl4 gas in an atomic layer deposition system, the CsPbBr3 film is step-wise converted to CsPbCl3. Depending on the number of TiCl4 pulses, the emission of the resulting material can be tuned between 523 nm (CsPbBr3) to 413 nm (CsPbCl3). Thermal imprint is shown to significantly improve the material quality affording a narrow luminescence linewidth of 8 nm (FWHM). The resulting CsPbCl3 show ASE at 427.6 nm with a low threshold of 70 µJ/cm2. Our work states the first report of CsPbCl3 thin films showing ASE at room temperature.
1.1-O1
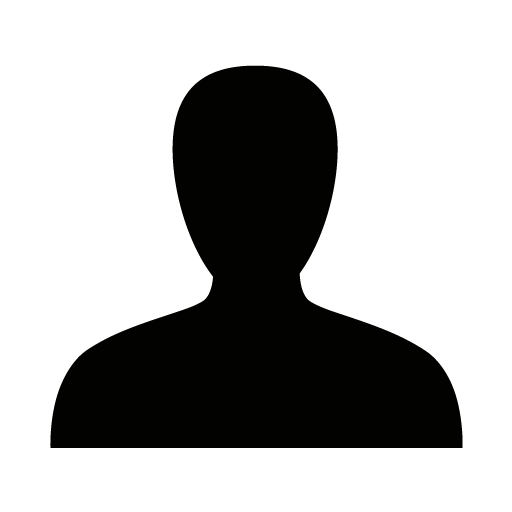
Lead halide perovskite (ABX3) nanocrystals are luminescent nanomaterials of significant interest for applications in displays, solar concentrators, and photodetectors due to their bandgap tunability across the visible spectrum, narrow emission profiles, and high radiative carrier recombination rates.[1] However, their emission properties are not uniform across the visible range. Blue emission remains challenging to achieve, while red emission often suffers from lower efficiency and stability compared to green, posing difficulties for pure perovskite-based white light generation. Porous scaffolds have been employed to fabricate various ligand-free perovskite nanostructures.[2-5] However, scaffold preparation methods have involved only the use of optically passive elements. In this work, we have developed GdVO4:Eu3+ and GdVO4:Dy3+ nanoparticles films with a regular pore size that can be infiltrated with perovskite precursors from the liquid phase. In this context, we prepare CsPbBr3 and Cs4PbBr6 nanocrystals, which emit green and blue light respectively. Upon ultraviolet excitation, Eu3+-doped nanophosphors emit red light, while Dy3+-doped nanophosphors emit light in the blue and yellow regions of the visible spectrum.[6] The combination of perovskite nanomaterials and multifunctional phosphor-based scaffolds enables the fabrication of transparent photoluminescent coatings with tunable spectral emission, controlled by the excitation wavelength. This synergy allows for the generation of white light with customizable hues ranging from warm (2300 K) to neutral (5500 K).
1.1-I2
Dr. Tze-Chien Sum is an Associate Professor at the Division of Physics and Applied Physics, School of Physical and Mathematical Sciences (SPMS), Nanyang Technological University (NTU) where he leads the Femtosecond Dynamics Laboratory. He is presently the Associate Dean (Research) at the College of Science. Tze-Chien received his Ph.D. in Physics from the National University of Singapore (NUS) in 2005, for the work in proton beam writing and ion-beam spectroscopy. His present research focuses on investigating light matter interactions; energy and charge transfer mechanisms; and probing carrier and quasi-particle dynamics in a broad range of emergent nanoscale and light harvesting systems. Tze-Chien received a total of 11 teaching awards from NUS and NTU, including the coveted Nanyang Award for Excellence in Teaching in 2006 and the 2010 SPMS Teaching Excellence Honour Roll Award. Most recently, he received the 2013 SPMS Young Researcher Award; the Institute of Physics Singapore 2014 World Scientific Medal and Prize for Outstanding Physics Research; the 2014 Nanyang Award for Research Excellence (Team); and the 2015 Chemical Society of Japan Asian International Symposium Distinguished Lectureship Award. More information can be found at http://www.ntu.edu.sg/home/tzechien/spms/index.html
Halide perovskites are forerunners for next-generation photovoltaics and light-emitting devices. The power conversion efficiencies of perovskite solar cells have exceeded 25%, while that of the external quantum efficiencies of perovskite light emitting devices have also breached the 20% mark. Their remarkable rise is driven by exceptional properties such as large absorption cross-sections, defect tolerance, significant spin-orbit coupling, long balanced charge diffusion lengths, slow hot carrier cooling, ion migration, and radiation tolerance etc. Furthermore, their versatile structures and diverse dimensionalities afford new levers for tunning their photophysical properties. Consequently, their applications have rapidly expanded beyond traditional optoelectronics into areas such as spintronics, radiation detectors, memristors, bioimaging, and quantum light sources. Of late, low dimensional halide perovskites have demonstrated great promise as single photon sources as well as bunched multiphoton sources. In this talk, I will focus on our recent efforts on the basic photophysics studies and engineering of perovskite quantum emitters [1-5].
1.2-I1
Exciton-polaritons are half-light, half-matter excitations arising from the strong coupling regime between cavity photons and excitons of semiconductors [1]. Behaving as superlative non-linear photons due to their hybrid nature, exciton-polaritons have been providing a fruitful ground for studying quantum fluid of light and realizing prospective all-optical devices. In this presentation, we present experimental studies on exciton-polaritons in resonant metasurfaces, which are composed of sub-wavelength lattices of perovskite pillars (see Figure). Room temperature polaritons are demonstrated with a remarkable Rabi splitting in the 200 meV range. We show that polaritonic dispersion can be tailored on-demand. This includes creating linear, slow-light, multi-valley shaped dispersions [2] as well as polarization vortex emission [3]. Finally, we observe experimentally the ballistic propagation of polaritons over hundreds of micrometers at room temperature, even with large excitonic components, some up to 80%. This long-range propagation is enabled by the high homogeneity of the metasurface, and by the large Rabi splitting which completely decouples polaritons from the phonon bath at the excitonic energy [4]. Our results suggest a new approach to study exciton-polaritons and pave the way for the development of large-scale and low-cost integrated polaritonic devices operating at room temperature.
1.2-I2
Materials that combine optoelectronic function with control over the spin degree of freedom are central for emerging quantum technologies, opto-spintronics, and provide exciting avenues for generating polarized light-emission. Hybrid metal-halide perovskites exhibit spin-split Rashba bands and strong spin-orbit coupling, which offer directions for extended spin life-times of excited states and efficient optical spin manipulation. To achieve optimal performance in applications, an understanding how material chirality links to spin state properties and dynamics needs to be established.
In the first part of this talk, we will present our efforts on gaining control over spin state properties and dynamics in solution-processable chiral hybrid perovskites through compositional and structural tuning. For this, we tailor the chiral crystal symmetry in novel chiral lead-free bismuth-based materials, highly-emissive low-dimensional chiral lead-halide systems, as well as chiral-achiral heterostructures.
In the second part, we will discuss time- and space-resolved investigations of excited state and spin dynamics in our novel chiral perovskites. We will present results on spin dynamics from ultrafast Faraday Rotation, polarized recombination dynamics, as well as spatio-temporal imaging of excitations using ultrafast transient microscopies.
1.2-I3
By combining the optoelectronic properties of halide perovskites (HPs) with chirality from inserted organic cations, chiral HPs brought new perspectives for chiroptical properties, such as non-linear optics or circularly polarized luminescence (CPL), or spintronic devices such as spin valves or spin-LEDs. Indeed, the emergent field of chiro-spintronics proposes to use chiral molecules as a substitute for ferromagnetic materials thanks to the spin-specific interaction between electrons and chiral molecules, a phenomenon called CISS, “chirality-induced spin selectivity”. Following this strategy, we prepared a series of chiral HPs and revealed both experimentally (mc-AFM) and theoretically (band structure and spin texture calculations) the influence of crystal symmetry elements on the spin polarization ability of this family of molecular materials (Figure a).[1] We also demonstrated the possibility to use such materials as spin valves. More recently, we reported a full series of lead-free chiral double perovskites showing strong structural distortions in the inorganic network.[2] In combination with their lead-based counterparts, such series will ultimately allow us to investigate the fundamental role of the metal ions on the CISS effect. On the other hand, revealing the ability of chiral HPs for chiroptical applications require a proper characterization of the thin film chiroptical responses, in particular circular dichroism (CD), considering the macroscopic interferences (linear dichroism LD, linear birefringence LB) inherent to solid-state samples, leading to the so-called antisymmetric LDLB effect (aLDLB) and symmetric LDLB effect (sLDLB). Since these macroscopic effects can be very strong in highly crystalline metal-halide thin films, an experimental guide to accurately discriminate between both CD, aLDLB and sLDLB was recently reported with the example of 1D chiral lead-halide networks.[3] However, in compounds with large optical anisotropy, such effects can be minimized by controlling the orientation of the polar axis with respect to the light beam propagation (Figure b). This strategy allowed us to characterize artefact-free CPL on both single crystals and thin films of 1D chiral lead-bromides with white-light emission (manuscript under revision).
1.3-I1
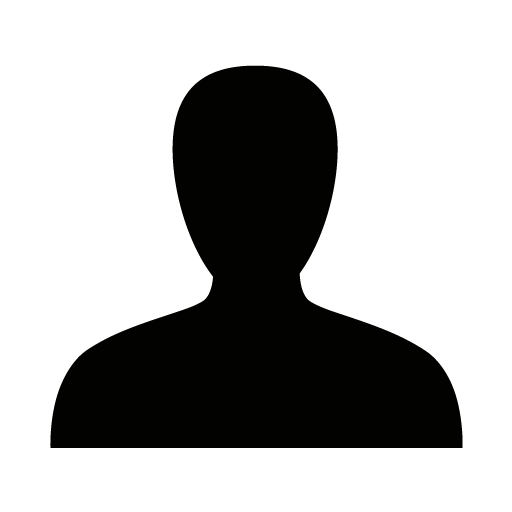
The solution processed semiconductors known as perovskites, or semiconductor perovskites (SP), has been widely studied as an idoneal platform to implement cost-effective integrated lasers. From this perspective, there is an important concern on developing a SP technology to implement photonic integrated circuits. In this context, we have recently demonstrated that a nickel acetate, Ni(AcO)2, sol-gel is an exceptional and non-expensive matrix for SP nanocrystals. The distinctive advantage of this alternative technology relies on the in-situ crystallization of the perovskite nanocrystals during the spin-coating deposition, resulting on thin films with PL quantum yields exceeding 80 % and outstanding ambient and mechanical stability. This work successfully demonstrates the potential of Ni(AcO)2 containing MAPbBr3 (MA:methylamonium) SPs nanocrystals for active photonics. These nanocomposites are easily spin-coated on a SiO2/Si to conform planar waveguides. Moreover, the concentration of nanocrystal in the matrix allows the tunability of the optical properties, resulting in thin films with a tailor-made refractive index and absorption. Under optimal concentrations, the nanocrystals are homogeneously dispersed in the film and the waveguide efficiently propagates the light with relatively low losses. Here, the excitation beam injected in the structure gives rise to the generation of photoluminescence (PL) of the nanocrystals, and under certain excitation and propagation conditions, the generation of amplified spontaneous emission (ASE) and the formation of narrow random lasing (RL) under relatively small threshold (µJ/cm2). These results mark a significant step towards realizing the potential of perovskite nanocomposites in the field of optical waveguides.
1.3-I2
Lead halide perovskite quantum dots have recently emerged as promising nano-emitters due to their excellent optical properties, including high brightness, tunable optical bandgap, reduced blinking, and easy and low-cost fabrication [1]. These properties make them potential candidates for realising a new generation of optoelectronic devices such as light-emitting diodes (LEDs), lasers, or photodetectors. At the individual quantum dot level, single photon emission at room temperature [2,3], long coherence times and photon indistinguishability with 50% visibility at cryogenic temperatures [4,5] have also been reported. These properties suggest that perovskite quantum dots could play a key role in the realisation of efficient single-photon sources based on solution-processed nanoemitters for applications in quantum optics and quantum communication. In this context, one of the main challenges is to couple individual perovskite quantum dots to optimised photonic structures in order to control and enhance the spontaneous emission properties of the nano-emitters using cavity quantum electrodynamics (cQED) effects.
In this talk I will present our recent results on cQED experiments on single perovskite quantum dots coupled to an optical microcavity. We have designed and implemented a reconfigurable open fibre-based Fabry-Pérot microcavity, specifically suited for CsPbBr3 perovskite quantum dots. It is based on a highly versatile setup that has previously been successfully optimised for single carbon nanotubes [6]. Unlike conventional monolithic microcavities, which are designed to ensure spatial and spectral matching to a specific nano-emitter and cannot be subsequently modified, this fibre microcavity is perfectly suited to solution-processed nano-emitters. It consists of a planar mirror on which the quantum dots are deposited and a movable concave fibre mirror. This geometry allows us both to ensure spatial and spectral matching for different perovskite quantum dots and to study the same nano-emitter in free space and in cavity configurations. I will show that previously characterised single CsPbBr3 quantum dots [7,8] have been successfully coupled to this microcavity. By comparing their photoluminescence lifetime in free space with that in the cavity configuration, a twofold acceleration of the emission lifetime due to the Purcell effect was consistently observed, corresponding to Purcell factors of up to 4.5. Furthermore, the reversible coupling of individual CsPbBr3 quantum dots to the cavity provides a highly interesting tool to precisely analyse the modification of the spectral features induced by the cavity coupling and to extract fundamental properties such as the vacuum Rabi coupling, which is of the order of 30 µeV in our system. These results pave the way for the realisation of a narrow-band efficient single-photon source at the cavity resonance frequency in the weak coupling regime using perovskite quantum dots.
1.3-O1
Halide perovskites show excellent optoelectronic properties including bandgap tunability, high radiative recombination rates and narrow emission lines that make them promising candidates for the next generation solar cells, LEDs and detectors.[1],[2],[3] Their optical properties and ease of processing make them very interesting to control light matter interactions to deliver devices with unique properties and enhanced performance. However, their thin film character is yet to be exploited to enable full control over the emission properties, something that would open avenues to surpass the luminous efficacies of conventional LEDs and facilitate their widespread adoption.
In this talk, we present a novel green perovskite LED architecture where enhanced emission and directionality on demand are achieved by means of a hybrid photonic-plasmonic structure.[4] We show how a code based on the transfer matrix model boosted by a genetic algorithm identifies the best combination of materials and thin film thicknesses to maximise outcoupled light with very narrow and controllable angular dispersion; all in a realistic fashion compatible with the fabrication of efficient LEDs. The experimental realization of the optimum designs allows us to demonstrate devices with amplified green emission selectively enhanced at different angles. Our low temperature process can tune the perovskite thickness on a nanometric scale to enhanced electroluminescence on demand from forward direction (0°) to up to 40°. This approach expands the role of the perovskite film from a mere emitter to an active photonic layer participating in the strong interference phenomena arising from the designed photonic-plasmonic nanostructures. Our methodology is versatile and easily integrable into cost-effective perovskite LEDs with emission lines covering the entire visible spectrum. Finally, we adapt this resonant cavity concept to demonstrate a highly spectral selective and robust perovskite photodetector, showing 2.4-fold EQE enhancement at the narrowband peak with respect to a broadband photodetector counterpart of the same perovskite thickness.[5]
2.1-O1
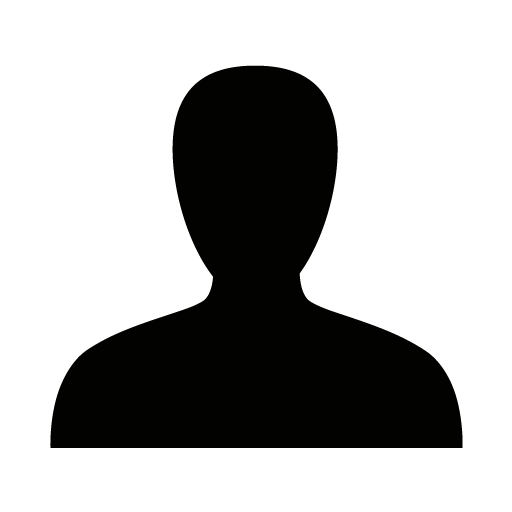
Metal halide perovskites (MHPs), particularly CsPbBr₃, have emerged as a transformative material for optoelectronic applications, offering unparalleled properties such as high carrier mobility, exceptional optoelectronic performance, large photoluminescence quantum yield, and superior stability under humidity and thermal stress. Its solution processability allows for easy fabrication using inkjet printing, an environmentally sustainable alternative to conventional manufacturing techniques [1].
In inkjet printing of perovskites, the characteristics of the functional ink and printing conditions are crucial in determining the final device performance, with annealing temperature playing a critical role in influencing the crystal structure and, consequently, the optoelectronic properties of the printed films. This study comprehensively investigates the impact of annealing temperature in vacuum oven from 45-200 °C on the properties of inkjet-printed CsPbBr₃ nanocrystal films printed on glass substrate [2]. The CsPbBr₃ nanocrystal inks were prepared in the ratio of 3:1 in dodecane and hexane solvent with each nanocrystal measuring 7-13 nm and the printing was performed using Dimatix inkjet printer.
The results reveal a significant dependence of photoluminescence (PL) intensity on the annealing temperature, with the optimal PL emission observed for devices annealed at 180°C. The change in the PL properties are related to the impact of annealing temperature on grain size, crystallinity, and film uniformity, which directly affect optoelectronic properties and stability corroborated by various characterizing techniques such as X-ray diffraction (XRD), X-ray photoelectron spectroscopy (XPS), scanning electron microscopy (SEM), Fourier-transform infrared (FTIR) spectroscopy, and ultraviolet-visible (UV-Vis) absorption spectroscopy providing conclusive evidence of the temperature-induced changes in crystal structure, phase purity, and optoelectronic performance. These findings underline the critical role of precise thermal processing in achieving high-performance inkjet-printed perovskite films, positioning CsPbBr₃ as a viable material to be printed on ITO coated glass substrate for LED applications [3].
2.1-I1
2.1-I2
Dr Stefania Cacovich is currently a CNRS researcher working at IPVF. Her research activity lies in the field of the advanced characterization of hybrid and inorganic materials for photovoltaic applications by employing a multi-scale and multi-technique approach.
Her research into hybrid devices started during her doctoral studies (2014-2018), carried out at the Department of Materials Science of the University of Cambridge (UK) under the supervision of Prof Caterina Ducati. Her thesis focused on the study of the chemical, structural and morphological properties of hybrid organic-inorganic thin films and photovoltaic devices using advanced analytical electron microscopy techniques. In 2018, she moved to Paris for a postdoctoral research position at IPVF to work on multidimensional spectrally and time resolved photoluminescence imaging methods. From 2020-2022, she was Marie Curie Individual Post-doctoral fellow in Physics at CNRS (UMR 9006) with a project aimed at exploring the fundamental photophysical processes underlying the operation of advanced optoelectronic devices.
Halide perovskite materials hold significant potential for solar energy and optoelectronic applications. However, enhancing their efficiency and stability necessitates addressing challenges related to lateral inhomogeneity. Photoluminescence imaging techniques are widely employed to measure their optoelectronic and transport properties1. While achieving high precision typically requires longer acquisition times, extended light exposure can significantly alter the perovskite layers due to their high reactivity, compromising data quality.
To address this issue, we propose a method to extract high-quality lifetime images from rapidly acquired, noisy time-resolved photoluminescence images2. Our approach leverages constrained reconstruction techniques, incorporating the Huber loss function and a specific form of Total Variation Regularization. This method effectively mitigates limitations imposed by local signal-to-noise ratios (SNR), allowing access to greater detail and features in the results. Through simulations and experimental validation, we demonstrate that our approach outperforms traditional pointwise techniques. Additionally, this analysis can be extended to determine the surface recombination rate, providing valuable insights for the advancement and optimization of halide perovskite materials. Furthermore, we identify optimal acceleration and optimization parameters tailored to decay time imaging of perovskite materials, offering novel perspectives for accelerated experiments essential to characterizing degradation processes.
Importantly, our methodology has broader applications. It can be extended to other beam-sensitive materials, various imaging characterization techniques, and more complex physical models for time-resolved decays.
2.1-O2
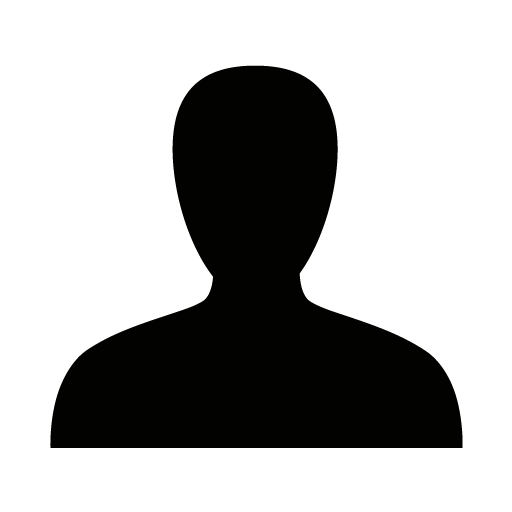
In 2025, the applicant became a Lecturer Professor at the Universitat Politècnica de Catalunya, within the Department of Electronic Engineering at the Escuela de Ingeniería de Barcelona Este, as a Serra Húnter Fellow. Previously, he spent six years as a postdoctoral researcher at the Universitat de Barcelona’s Department of Electronics Engineering.
With nearly 13 years of research experience, his expertise spans nanomaterials synthesis, ink formulation, electronic design, inkjet-printed device fabrication, and characterization of semiconducting and functional materials for optoelectronic and memory applications. His key contributions include advancements in flexible inkjet-printed metal oxides, 2D graphene materials, and perovskite-based optoelectronic devices (LEDs, solar cells, photodetectors), addressing challenges in sustainable flexible electronics.
The SH fellow has developed expertise in nanomaterials characterization, scalable inkjet-printed device fabrication, and independent project development, securing research funding. Notable collaborations include Saule Technology, Avantama AG, ETH Zürich, UJI, and Cambridge University. During a five-year postdoctoral fellowship, he co-supervised one PhD, two master’s theses, and three bachelor’s projects, demonstrating strong mentorship and communication skills. He has authored 19 papers (10 as first author), with an h-index of 12 and over 330 citations (Scopus), participated in 54+ conferences.
Metal halide perovskites (MHPs) are direct bandgap semiconductors with excellent potential and perspectives to become an alternative to traditional semiconductors in the implementation of future devices for optoelectronics and photonics. Furthermore, MHPs have shown remarkable performance for potential optoelectronic applications beyond photovoltaics. The continuous development in smart devices and microsystems for the control of industrial processes, biomedical sensors and instruments, visible and NIR light communications (in the internet of things, for example), object imaging, and cameras for artificial intelligence and robotics is triggering new demands for photodetection concepts and their integration in photonic chips. However, for the explosion of such future photodetector technologies, some other requirements are important: low cost and low CO2 footprint in fabrication, low operation voltage, small volume, high speed, flexibility, biocompatibility, solar-blind and many other features (for different applications). Therefore, a real and very interesting technology for a future generation of photodetectors can arise on the basis of MHPs, given their excellent optoelectronic properties and the fact that they can be synthesized at low temperatures via fast and simple processes, and films can be easily formed by low-cost solution processing techniques (spin-coating, spray-coating, dip-coating, doctor blading, inkjet printing). Among the MHPs, lead-free perovskite compounds are the most promising non-toxic alternative for developing photodetectors.
This study investigates the development of (PEA0.5,BA0.5)2FA9Sn10I31-based photodetectors fabricated using inkjet printing on both glass and flexible substrates, emphasizing their potential for advanced optoelectronic applications. The focus is on optimizing the crystallization process of (PEA0.5,BA0.5)2FA9Sn10I31 layers, ensuring high-quality films with minimal defects, and enhancing charge carrier mobility. The fabrication process employs solution-based techniques compatible with large-scale production, making the devices suitable for integration into wearable electronics and curved displays. Various strategies, such as interface engineering, compositional tuning, and passivation layers, were explored to improve stability and light sensitivity. The research demonstrates that inkjet-printed (PEA0.5,BA0.5)2FA9Sn10I31 layers (200 nm thickness) exhibit uniform crystal structures, enabling high responsivity and stable performance under different environmental conditions. The fabricated devices exhibited high responsivity across a wide range of light intensities and wavelengths, including visible and near-infrared regions. Persistent photoconductivity due to carrier trapping mechanisms was observed, highlighting the need for further engineering to enhance performance stability. Photoconductive properties were evaluated using continuous and modulated light sources. Devices on glass substrates showed higher efficiency and responsivity compared to flexible PET substrates, likely due to substrate interactions and defect levels. Responsivities ranged up to 50 A/W under low light intensity, with stability improvements observed even after 30 days of operation. Notably, the devices showed improved performance over time, indicating slow film curing and effective encapsulation. The results highlight the potential of inkjet-printed (PEA0.5,BA0.5)2FA9Sn10I31 photodetectors for next-generation photonic applications, where flexibility and low-cost fabrication are crucial. Despite the promising performance, challenges such as sensitivity to moisture and oxidation in tin-based perovskites remain, impacting long-term device stability. This research provides valuable insights into the practical deployment of lead-free perovskites in photodetection technologies.
2.2-O1
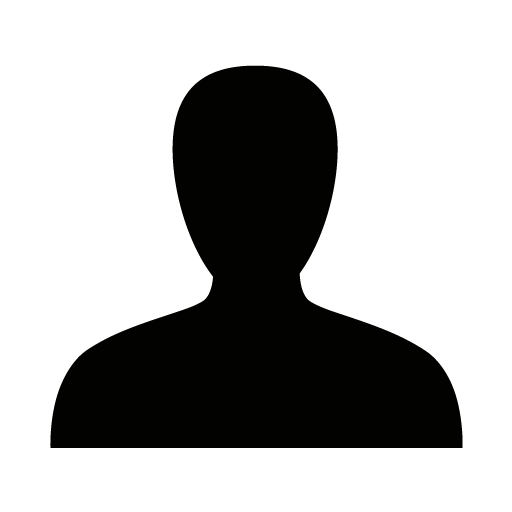
Halide perovskites have gained interest as light absorber materials due to their outstanding properties for optoelectronic devices, including record efficiencies in solar cells and ignited research in photodetectors. However, the challenges remain unresolved, including the inherent instability of hybrid perovskite materials and the formation of intermediate phases during solvent-based synthesis methods. To overcome these challenges, we developed the mechanosynthesis (MS) of hybrid perovskite powders and perovskite-graphite composites for visible light photodetector applications. Using this scalable and solvent-free ball-milling technique, we synthesized methylammonium lead iodide (MAPbI3) hybrid perovskites and MAPbI3-graphite composite powders, and characterizations have shown that nanograins (∼10 nm) in aggregates share common crystallographic orientations [1]. The synthesized composites were designated as MAPI-4h (without graphite), MAPI-Gr3%, and MAPI-Gr5%, with high yields of 86.3%, 84.3%, and 89%, respectively. The obtained powders were compacted into pellets via uniaxial compaction, and Ag electrodes were deposited on top of pellets to fabricate photodetector devices. The devices were designated as Ag-MAPI-4h, Ag-MAPI-Gr3%, and Ag-MAPI-Gr5%, respectively. The formation of ohmic contact was verified by stable linear current-voltage (I-V) curves in the dark. With the addition of Ag electrodes, the devices exhibited ten times higher current and more efficient charge extraction compared to pellets without Ag electrodes. An uniform distribution of few-layer graphite within the MAPbI3 matrix was noticed in pellets. The effect of graphite addition was also studied for improving the photodetection performance. The Photoresponsivity was measured around 750 nm wavelength. The photoresponsivity of Ag-MAPI-Gr5%, Ag-MAPI-Gr3%, and Ag-MAPI-4h were respectively 0,13 ⅹ 106 AW-1, 7,7 ⅹ 100 AW-1, and 58,87 ⅹ 10-3 AW-1. Fast rise time (τr) and fall time (τf) were recorded for Ag-MAPI-4h as 1.1 s and 1.68 s, respectively, under light. Graphite should enhance the conductivity and hence the charge transport; however, it diminishes the photoresponsivity. Graphite-enhanced Raman scattering was observed with the composite with 5 wt % graphite, showing its strong resistance to photodegradation and suggesting a charge transfer between graphite and MAPbI3. Such charge transfer is also in agreement with the quenching of the PL with an increasing amount of graphite. The presence of graphite would induce a local modification of the Coulomb interaction, “attracting” the charge carriers at the graphite and MAPbI3 interfaces and thus favoring the charge separation and transport. This high photoconductive gain matches with the trapping of one type of charge carrier, while the other one is multiplied via injection from the contact electrodes, thus contributing even more to the photoconductivity. These results demonstrated at first the great potential of mechanosynthesis, which is a green, easy scalable and eco-friendly powder synthesis technique to develop light-sensitive materials for photovoltaic and photodetection applications.
Keywords : Mechanosynthesis of perovskites, MAPbI3@Graphite composites, visible-light photodetectors, solvent-free synthesis, perovskite photodetectors
2.2-I1
Halide perovskites have garnered significant attention over the past decade due to their remarkable optoelectronic properties. Perovskite light-emitting diodes (PeLEDs) have surpassed the 20% external quantum efficiency (EQE) limit for planar organic LED (OLED)-like device structures [1]. A bottom-up approach in perovskite material science highlights the potential of solution-processing techniques for device fabrication. Most high-efficiency PeLEDs reported to date have been fabricated using the spin-coating technique, which facilitates the integration of diverse materials [2]. However, spin coating is unsuitable for mass production, as it results in considerable material waste, with only a small fraction of the solution contributing to the thin-film.
Inkjet printing has emerged as a versatile and cost-effective alternative for fabricating perovskite-based devices. This technique offers high precision, scalability, and seamless integration with electronic and photonic components. Inkjet printing enables controlled deposition of materials, yielding uniform and high-quality perovskite films essential for efficient device performance. In 2020, the first PeLEDs with inkjet-printed perovskite layers were reported, achieving moderate efficiencies below 10% [3,4]. Subsequent advancements include the development of fully inkjet-printed PeLEDs [5], lead-free inkjet-printed PeLEDs [6], and the first fully inkjet-printed inorganic PeLEDs [7].
This paper reviews the key features of the inkjet printing technique, emphasizing its advantages over traditional methods, such as scalability, material efficiency, and precision in layer deposition. The discussion includes the fundamentals of solution-processing methodologies and the optimization of precursor formulations, highlighting the challenges in achieving uniform crystal formation and stable film morphology. Despite its potential, inkjet printing faces several limitations, such as coffee-ring effects, nozzle clogging, and difficulties in controlling film thickness and homogeneity across large areas. Addressing these challenges requires innovations in ink formulations, printing protocols, and the design of advanced hardware.
Additionally, sustainable strategies are examined, including the use of lead-free perovskites and eco-friendly solvents, which aim to reduce environmental impact while maintaining device performance. Examples of recent advancements are presented, such as fully inkjet-printed devices integrating all functional layers, which demonstrate the scalability and versatility of the technique. Future perspectives focus on the integration of inkjet printing into roll-to-roll manufacturing systems, enabling large-scale, cost-effective production of PeLEDs. These developments could unlock new opportunities for commercial applications, including flexible displays, wearable electronics, and next-generation lighting systems.
2.2-O2
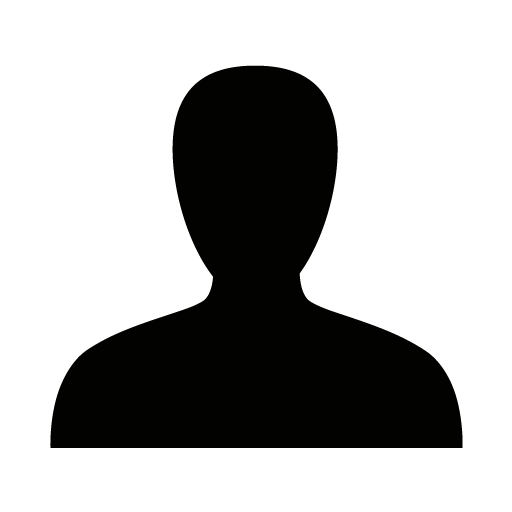
The demand for sustainable and efficient optoelectronic materials has led to significant interest in lead-free halide perovskites. Among these, CsCu2I3, a one-dimensional copper-based halide perovskite, has emerged as a promising material due to its excellent optoelectronic properties, high stability, and non-toxic composition. CsCu2I3 exhibits strong quantum confinement and broad absorption in the ultraviolet (UV) region, making it suitable for applications in photodetectors. Its self-trapped exciton (STE) emission mechanism ensures a high photoluminescence quantum yield in the visible range and substantial Stokes shift, critical for UV detection. [1], [2]
In this study, CsCu2I3 was processed into thin films using an environmentally friendly inkjet printing method. A stable precursor ink was synthesized by dissolving CsI and CuI in dimethyl sulfoxide (DMSO) at a 1:2 molar ratio to obtain a 0.5 M solution. Films were printed onto ozone-treated ITO-patterned glass substrates with interdigitated electrodes at varying distances (50–200 µm) in order to define our photoconductor, with a thickness about 50 nm. Inkjet printing parameters such as drop spacing and platen temperature were optimized to produce high-quality and uniform films. The printed films were annealed at 100 °C under vacuum to enhance their crystallinity and stability.
The fabricated photoconductors were characterized both optically and electrically. Transmittance and reflectance measurements of thin films revealed an absorption edge at Eg = 3.75 eV, i.e., wavelengths shorter than 330 nm are efficiently absorbed. Electrical measurements, including current-voltage and current-time responses under illumination across the 280–500 nm range, confirmed the significant photodetection capabilities of our photoconductors in the UV region (see Figure), with responsivities above 0.1 A/W at wavelengths below 300 nm at 5 V. These results position inkjet-printed CsCu2I3 as a versatile, lead-free material for the next generation of UV photodetectors. This work highlights the potential of scalable, low-cost manufacturing techniques for eco-friendly photonic applications.
2.2-O3
Metal halide perovskites are emerging as promising semiconductors for cost-effective and high-performance optoelectronic devices. Tremendous research attention has been attracted to perovskite layers, however, an in-depth understanding of how the buried charge transport layers affect the perovskite crystallization, compositions and film quality, though of critical importance, is currently unclear.
Here, we firstly, systematically studied synergy effects between perovskite precursor stoichiometry and interfacial reactions for high-performance perovskite LEDs (PeLEDs) and establish useful guidelines for rational device optimization. We reveal that efficient deprotonation of the undesirable organic cations by a metal oxide interlayer with a high isoelectric point is critical to promote the transition of intermediate phases to highly emissive perovskite films.[1] We further reveal that the deprotonation of FA+ cations and the formation of hydrogen-bonded gels consisting of CsI and FA facilitated by zinc oxide underneath, effectively removes the Cs-FA ion-exchange barrier, promoting the formation of phase-pure CsxFA1-xPbI3 films with emission filling the gap between that of pure Cs- and FA-based perovskites.Based on this discovery, we successfully fabricated a set of highly emissive CsxFA1-xPbI3 perovskite films with fine-tuning Cs-FA alloying ratio for emission-tuneable near-infrared light-emitting diodes (NIR-LEDs).[2]
We further developed a multifunctional display using highly photo-responsive metal halide PeLEDs as pixels following works mentioned above. by careful control of the interfacial reactions, we achieved strong photo response of the PeLED pixels. Therefore, the display can be simultaneously used as touch screen, fingerprint sensor, ambient light sensor, and image sensor without integrating any additional sensors. In addition, decent light-to-electricity conversion efficiency of the pixels also enables the display to act as a photovoltaic device to charge the equipment.[3] The multiple-functions of our PeLED pixels can not only simplify the display module structure and realize ultra-thin and light-weight display, but also significantly enhance the user experience by these advanced new applications. As such, our results demonstrate great potential of PeLEDs for a new generation of displays for future electronic devices.
2.3-I1
Low-dimensional metal halide perovskites are attracting great interest for photovoltaics and photonics. In particular, 2D tin perovskites have been shown to have good optical gain properties which make them promising for applications as coherent light sources. [1] On the other hand, the ability of lead-based 2D perovskites to sustain lasing remain highly controversial. [2] Here we show that both Sn and Pb-based 2D perovskites thin films can achieve amplified stimulated emission, and compare their properties as function of the optical pumping conditions as well as of the materials’ structural characteristics. By employing 1H, 13C, 15N, 119Sn and 207Pb solid state NMR spectroscopy, we were able to discern the local structural environments of the 2D perovskite of interest through their characteristic spectral fingerprints. Spin relaxation dynamics measurements reveal that the local supramolecular spatial arrangements, the molecular motions and structural rigidity are key factors shaping the energetic landscape of the material and its luminescence properties. Our work provides a deeper understanding of the structure-properties relationship of these soft semiconductors to assist the rational engineering of materials with improved optical properties for lasing applications.
2.3-O1
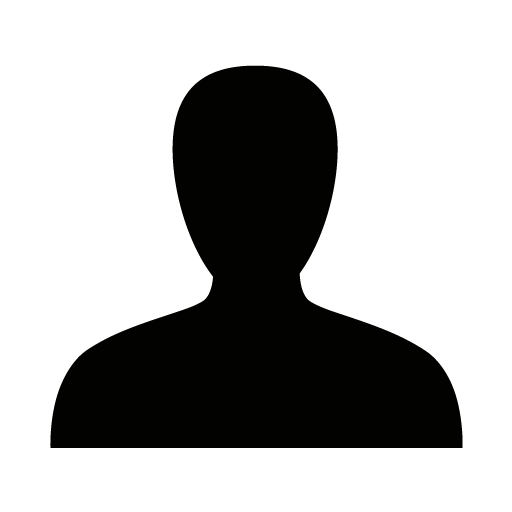
The study on lead halide perovskite nanocrystal (LHP NC) light-emitting diodes (LEDs), despite their recent existence, has seen a substantial progress in the research community. With their significantly increased surface area, passivation of surface with appropriate ligands is crucial. While advances in stability and optical properties are outstanding, the electrical accessibility of NCs has gained less attention so far. Specifically, efficient electroluminescence in LEDs rely on a thorough comprehension of the influence of charge carrier injection into the emitting material1.
In this study, we investigate commercial cubic CsPbBr3 NCs passivated with oleyl amine/oleic acid (OLA/OA) and ligand-exchanged NCs with didodecyldimethylammonium bromide (DDABr). It is known that long capping ligands impede carrier injection, making them ineffective in LED fabrication2. Transmission electron microscopy (TEM) images after ligand exchange show a reduction in interparticle distance along with nuclear magnetic resonance (NMR) spectroscopy indicating minimal ligand coverage on ligand-exchanged particles. Improved carrier balance is observed in DDABr capped NCs and photoelectron spectroscopy reveals a reduction in hole injection barrier. Resulting LEDs fabricated with ligand exchanged NCs exhibit a higher and almost constant external quantum efficiency (EQE) at high current densities, indicating a better carrier balance3. Density functional theory (DFT) studies reveal the occurrence of trap states with excess OLA4, whereas a favorable bandgap shift is expected with DDABr capped NCs. These results suggest that the issue of LHP NCs in PeLED fabrication is not just the long insulating ligands alone; ligand coverage and the type of anchoring group is important as well. Thus, a deeper understanding of the interaction of the ligand attached on the NC surface is required.
1.2-I1
Doctor Min Zhou. Lectuer in School of Electrical and electronic engineering, Huazhong University of Science and Technology (HUST).
Graduate from Wuhan University in 2014, getting the doctor’s degree. Postdoctoral research in HUST from 2014 to 2016. Research interest in advanced energy storage systems and novel energy storage materials for grid scale energy storage applications. Research work has been published on Advanced Materials, Advanced Energy Materials, Energy & Environmental Science et al.
Grid-scale energy storage technologies is important and in urgent need for the renewable energy applications. Among the most energy storage technologies, electrochemical energy storage technologies, such as batteries, shows the great advantages of simple structure and high efficiency, which is developing quickly and widely applicated for varied fields of EES. For the electrochemical energy storage technologies, the electrode/electrolyte play the most important roles for the mass and ion transport. The ionic conductivity and mechanical strength of electrode/electrolyte layers directly influence the energy/power densities and cycling stability of the batteries. Thus, engineering a stable artificial SEI layer with sufficient strength and high interface energy is an efficient strategy to accelerate the ion/electron transfer kinetics as well as guarantee the stable and reversible redox reactions.
Plasma is generated by high voltage ionization, constituted of highly reactive species, which can easily form a large number of active sites on the material surface to construct interface layers with certain components. Lithium metal is considered as the most ideal anode for high energy batteries due to its ultrahigh theoretical capacity. However, the low coulombic efficiency and lithium dendrites lead to the poor cycling stability of lithium metal batteries. To solve these problems, artificial SEI layers with certain species of LiF, Li2C2 and polythiophene are prepared through the plasma treatment. Benefiting from the high mechanical strength of LiF, low Li+ diffusion barrier of Li2C2 and flexible structure of the polythiophene, modified Li anodes exhibit an long-term cycling stability of 8000 h with dendrite free structure. When coupling with LiFePO4, the cell using P-PTh-Li can obtain a reversible capacity of 94% after 500 cycles, compared to that of 62% capacity retention of Li. Furthermore, CF4 plasma was further employed for the treatment of PP separator. The grafted polar groups enhance the ionic conductivity and lithium-ion transference number of the separator. Moreover, the introduction of fluorine-containing functional groups participates in the formation of LiF-rich SEI film, which can regulate the uniform deposition of lithium ions and inhibits lithium dendrite growth.
1.2-I2
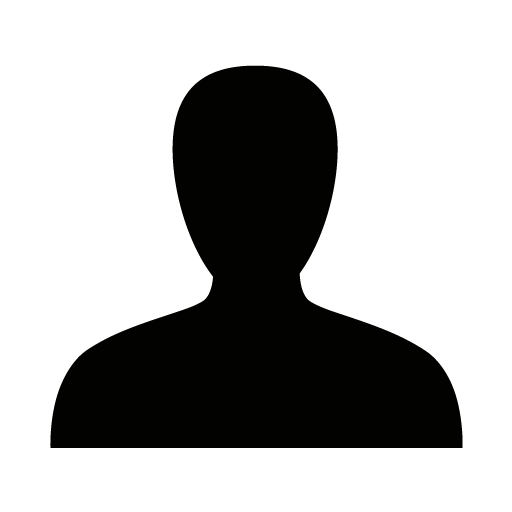
Liquid metal batteries (LMBs) are highly promising for grid-scale energy storage due to their outstanding kinetics, scalability, and long lifespan enabled by their unique three-liquid-layer structure. However, challenges remain with the positive electrode, including fluctuations at the electrode/electrolyte interface during charge and discharge cycles, as well as poor wettability on the current collector. These issues introduce excess electrical resistance and hinder rate capability, ultimately compromising cycling stability. To address the rate capability, we propose an operando strategy for the formation of Li₂Te with a multi-channel structure. This engineered structure enhances ion transport during cycling, significantly improving rate performance. The Li || Sb-Bi-Te₅ cell demonstrated exceptional capacity retention of 84.4% at 1000 mA cm⁻², compared to only 43.8% for the Li || Sb-Bi cell. To enhance electrode wettability, we incorporated 4 mol% Se into a Bi-based metal, forming a highly surface-active interface layer. This layer effectively reduced the contact angle with 304 stainless steel (SUS304) from 144.7° to 74.3°, significantly improving adhesion. The resulting 20 Ah Li || Bi-Se₄ cell (where Se constitutes 4 mol% of Bi) exhibited remarkable cycling stability, achieving 1200 cycles with a minimal capacity fade rate of just 0.00174% per cycle.This straightforward and efficient approach provides a viable pathway to producing stable LMBs with extended lifespans, advancing their practical implementation for large-scale energy storage.
1.2-O1
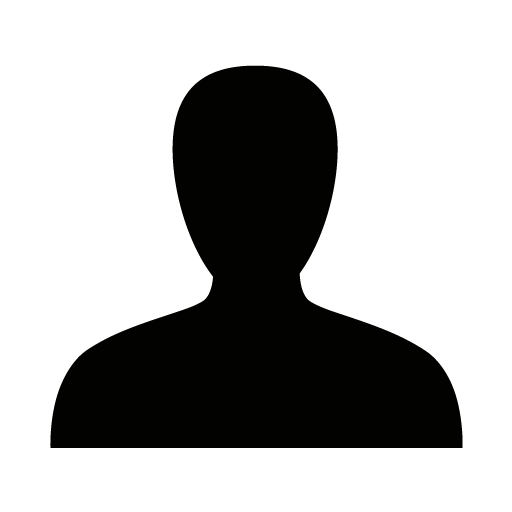
Present-day technologies contribute significantly to environmental strain through the emission of exhaust gases, underscoring the need for cleaner alternatives. Electrocatalysis offers promising solutions to replace traditional processes in both the manufacturing and energy sectors. More precisely, various electrocatalyst surface modifications have proven effective in improving the Oxygen Reduction Reaction (ORR), a reaction of critical interest in fuel cell technology. Nonetheless, several obstacles impede the commercialization of electrocatalyst surface modifications, one being inadequate quantification of active sites.
Here, we focus on enhancing electrocatalytic interfaces by electrochemically modifying the polycrystalline platinum (Pt-poly) surface to create a more favorable environment for electrocatalytic reactions. We achieve surface modification by electrochemical biasing in the presence of dicyanamide anions (DCA) and then characterize the modified electrode electrochemically. Using rotating disc electrode (RDE) measurements, we analyze surface probe reactions, including N2O reduction (Potential of Zero Total Charge determination), Oxygen Reduction Reaction (ORR), and Hydrogen Oxidation Reaction (HOR).
Our findings indicate that the newly formed species, transforming platinum into a +2 oxidation state, partially block the platinum surface. Nevertheless, sufficient free active sites remain available for proton, hydrogen, oxygen, and nitrous oxide adsorption and manifest higher catalytic activity towards corresponding reactions. This is likely due to electrostatic interactions, which inhibit water discharge and adsorption of anions. Furthermore, we present a new, non-destructive surface area determination method based on N2O reduction probing. Overall, the selective nature of this modification holds significant promise for applications in electrocatalytic reactions.
1.3-O1
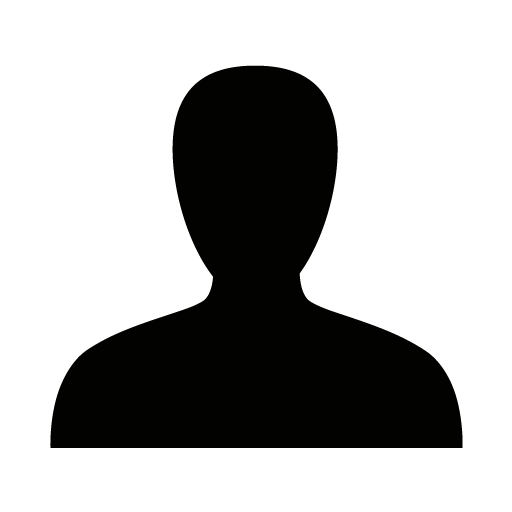
Understanding the electronic and ionic transport properties inside active cathode materials and through its surroundings in lithium-ion battery cathodes is crucial for optimizing battery performance. Such studies are most often done by EIS measurements in combination with rate performance and cyclability. However, these measurements can at most link changes in performance with changes in average resistance values which can, with at least some ambiguity, be attributed to certain interfaces and interphases. For a particle composite electrode with intrinsic distribution in porosity, particle size and tortuosity, the number of interfaces and their variations are innumerable. The number and positions of the electronic contacts made to one single active material particle alone is difficult to predict. The distance between the carbon nanoparticle chains wrapped around the particle determine the resistive drop and current/potential distributions. Furthermore, the electrical contact of the (semiconductor) active material with current collector and carbon black is simply assumed to be Ohmic with inconsequential contact resistance independent of the state-of-charge. Especially at high C-rates, these electronic effects might affect battery operation. We present a methodology to build experimental models to characterize and quantify the individual interfaces using thin-film stacks and patterning of metallic contacts with controlled area and pitch. The quantitative characterization of single interfaces can be used as input parameters for mathematical modeling of simulated electrode structures.
In this work, we will present a strategy for patterning Lithium Manganese Oxide (LMO) thin films, while preserving both the electrochemical activity and the structural morphology of the films. Having successfully established this process, we patterned metallic test structures on top of LMO films. Furthermore, employing the transmission line method [1], we calculated the conductivity of LMO and the contact resistance between LMO and the metal test structures. This approach enabled us to effectively benchmark the electron transport capabilities of different materials that serve as current collectors. Next to quantification of contact resistance, our results provided insights in the effect of grain or crystal size on conductivity of the LMO films. The grain size is controlled by the thermal budget during crystallization of the deposited films
In a further example, we address the use of titanium dioxide (TiO2) protective coating, deposited via atomic layer deposition (ALD) on our LMO films. The few nanometers thin film thickness permits lithium ions to traverse the film, while it slows down cathode degradation and suppress side reactions with electrolytes [2]. Although TiO2 is a dielectric and generally not expected to enhance electron transport directly, it can significantly affect the interface where electron transfer occurs. Our study aimed to determine the impact of TiO2 presence on contact resistance and to establish how its thickness could influence both electron transport characteristics and lithium-ion insertion/extraction dynamics. This analysis provides insights into the optimal thickness of TiO2 layers before they begin to compromise the cathode’s performance, offering a refined understanding of how such protective layers interact with both the electron and ionic transport.
The developed methodology already provides valuable insights into the effects of coatings and allows for the benchmarking and exploration of new materials. Looking ahead, this approach will enhance our understanding of the processes occurring within the composite cathode.
1.3-I1
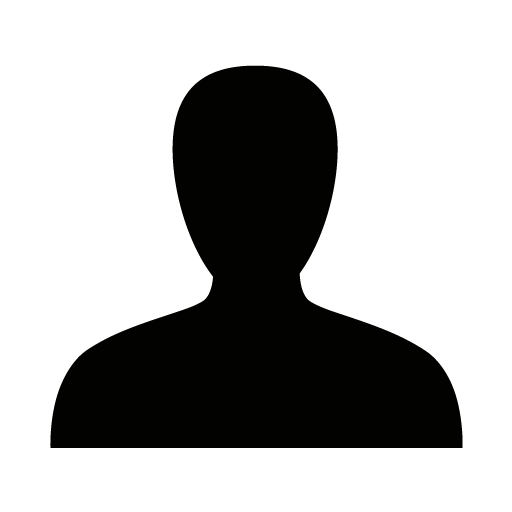
Silicon monoxide (SiO)-based anode materials are one of the most intensively investigated class of materials for use as high-capacity anodes in lithium-ion batteries. However, their low initial Coulombic efficiency (ICE), resulting from the irreversible electrochemical reaction of the amorphous SiO2 (a-SiO2) phase in the SiO, restricts the wide-spread adoption of SiO-based anode materials in lithium-ion batteries. In this work, we deomonstrate Si/M-silicate(M: Li, Mg, Ca) nanocomposite materials based on the dehydorgenation reaction of metal hydrides to improve the electrochemical performance of SiO. The resulting Si/M-silicate nanocomposite materials showed much improved electrochemical performance compared to pristine SiO. Laser-assisted atom probe tomography(LA-APT) combined with high resolution transmission microscopy(HR-TEM) clearly revealed that two exothermic reactions during prelihitation process related to microstructural evolution are key in optimizing the domain size of Si active phase and metal silicate buffer phase, and their topological arrangements in prelithiated SiO materials. We also report that the pre-emptive formation of irreversible phase combined with high-energy mechanical milling (HEMM) process can simultaneously improve both the ICE and long-term cycle performance by effectivley mitigating the volume expansion of Si during cycling, resulting in improved long-term cycle stability.
1.3-I2
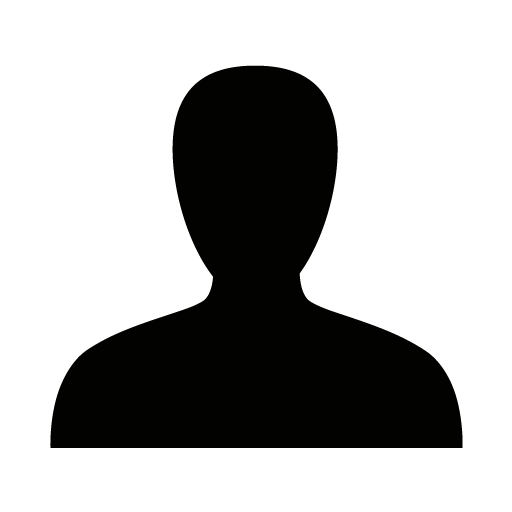
Electrocatalysts is essential for the commercilization of fuel cells. Currently, Pt is the key element on both anode and cathode. Since Pt is costly and scarce, it poses a question of how to lower the Pt loading and increase the efficiency. Most of previous studies focused on Pt alloyed with some 3d-transition metals, such as Fe, Co, Ni, etc. However, the activity and stability are not good enough for fuel cell applications. Recently, ordered intermetallic nanoparticles have attracted some attention, since the ordered intermetallic phase provides definite composition and structure. They can provide predictable controls over structural, geometric, and electronic effects, which are not afforded by alloys. However, previous reports on ordered intermetallic are mainly used as anode catalysts, such as formic acid oxidation. And the synthesis procedure is very complex. More importantly, the particles are unsupported and it is not easy to clean the particle surface. In our studies, we present that carbon supported ordered intermetallic nanoparticles can be easily formed using a simple impregnation-reduction method followed by high temperature pretreatment. Besides, by selecting special 3d-transition metals, Pd-based ordered intermetallics can also be formed. When used as electrocatalysts for fuel cells, the ordered imtermetallics exhibit both the enhanced activities and stabilties.
1.3-O2
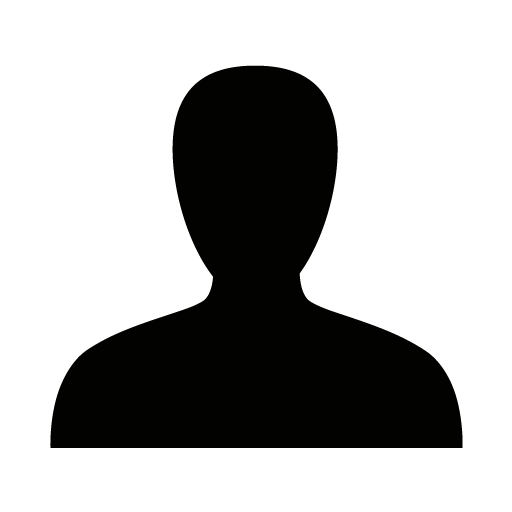
Lithium metal all-solid-state batteries promise to deliver a step-change in cell energy density and safety.[1,2] However, at charge/discharge rates on the order of 1 mA cm2, plating of lithium results in growth of lithium filaments through the solid electrolyte (dendrites),[3,4] and stripping of Li leads to contact loss between the lithium anode and solid electrolyte (voids).[5-7] This talk will cover our recent findings on the mechanisms of dendrite growth and voiding, and the testing protocols used to determine the maximum failure-free rates of charge/discharge.
Mechanism: Synchrotron-source X-ray computed tomography enables us to image the evolution of interfaces in a solid-state battery during operation. Visualization of the formation of dendrites and voids, in combination with modelling, provides insights into how these failure mechanisms might be prevented.[3,4,6]
Testing protocols: As solid-state batteries move towards commercialisation, it is increasingly important to determine the maximum rates at which they can be charged/discharged without formation of dendrites/voids. Our recent work investigates some conventionally used testing protocols, and identifies how these can be improved to increase accuracy and reproducibility.
1.1-I1
After her PhD degree in Telecommunications and Microelectronics Engineering on flexible dye solar cells, awarded by University of Rome ‘Tor Vergata’ in 2014, Dr De Rossi spent nearly 4 years abroad, working as a Technology Transfer Fellow in SPECIFIC Innovation and Knowledge Centre at Swansea University (UK). She was part of the PV team led by Prof T.M. Watson, focusing on the upscaling of printable perovskite solar cells, and lead of the stability activity within his group.
She is currently a fixed term researcher (RTDa) in the group led by Prof F. Brunetti, working on smart designed, fully printed flexible perovskite solar cells and photocapacitors.
Several photovoltaic technologies have been investigated so far for indoor applications: hydrogenated amorphous silicon, which reached a maximum power conversion efficiency (PCE) of 21% at 1000 lux [1], Dye Sensitized Solar Cells (DSC), which achieved a record of above 30% [2], organic photovoltaics (OPV), that reached a maximum efficiency of above 30% [3], and lead halide perovskites (PSC) that have recently led to an outstanding indoor PCE of 40.1% [4].
When moving to flexible substrates, that are very attractive for the low cost manufacturing, the easy integration and the possibility to achieve high power-to-weight ratio [5], the overall efficiency of the devices drops down to about 12% for DSC [6], to about 33% in the case of OPV [7] and to 32.5% for PSC [8].
In this talk, we will focus on and explore the application of flexible perovskite solar cells for indoor light harvesting, spanning from photocapacitors [9] to possible strategies to increase the performance, e.g. tandem configuration with other PV technologies [10], from more sustainable materials (charge transport layers, top electrodes, solvents, etc) [11] to large area deposition techniques with reduced material wastage and energy consumption [12].
1.1-I2
The escalating demand for sustainable energy solutions in recent years has highlighted the potential of indoor photovoltaics (IPVs) as a viable alternative for powering Internet of Things (IoT) devices. While lead halide perovskites have emerged as frontrunners due to their remarkable power conversion efficiencies (PCE) nearing 45% under indoor lighting conditions, their inclusion of toxic lead has prompted a thorough investigation into safer alternatives. This communication focuses on the sustainability of lead-free perovskite-inspired materials (PIMs), particularly those containing pnictogens such as bismuth (Bi) and antimony (Sb), as promising candidates for IPV applications.[1],[2]
The development of eco-friendly IPV technologies is crucial for reducing reliance on batteries which contribute to environmental degradation through resource extraction and waste generation. Traditional IPV technologies based on amorphous hydrogenated silicon (a-Si:H) offer PCE values up to 30%. However, the innovative use of lead-free PIMs has shown PCEs approaching 10% in early research phases, indicating significant potential for future advancements. This study utilizes a life-cycle assessment (LCA) approach to evaluate the environmental impacts of various PIMs, emphasizing their raw material availability, energy consumption, and waste generation.
Our findings reveal that while the PCE of PIMs plays a pivotal role in their overall environmental footprint and components such as the metal electrode and charge transport layers significantly influence their sustainability. Among the evaluated materials, a Bi-Sb alloy emerged as the most promising candidate, demonstrating a reduced environmental burden compared to a-Si:H under industrial-scale processing conditions. Extended simulations indicate that the industrial-scale implementation of Bi-PIMs can lead to a notable decrease in cumulative energy demand and carbon emissions, showcasing their potential as sustainable IPV technologies.
Moreover, this research underscores the critical importance of exploring the toxicity and criticality of raw materials used in the synthesis of these PIMs. While bismuth is recognized for its negligible toxicity and has been utilized in medical applications, antimony presents concerns regarding its occupational exposure risks.
In conclusion, the sustainability of lead-free PIMs for IPV applications is multi-faceted, encompassing not only their energy conversion efficiencies but also their environmental impacts throughout their lifecycle. Our study provides the first robust evidence of the potential for pnictogen-based PIMs to serve as viable, eco-friendly alternatives to lead-based materials, thereby addressing the growing need for sustainable energy solutions in the rapidly expanding IoT landscape. Future research efforts should focus on optimizing the efficiency of these materials while minimizing their environmental footprints, advancing the development of cost-effective and sustainable IPV technologies. The findings presented herein are crucial for guiding the scientific community and policymakers towards the realization of sustainable energy solutions that are not only efficient but also environmentally responsible.
1.1-O1
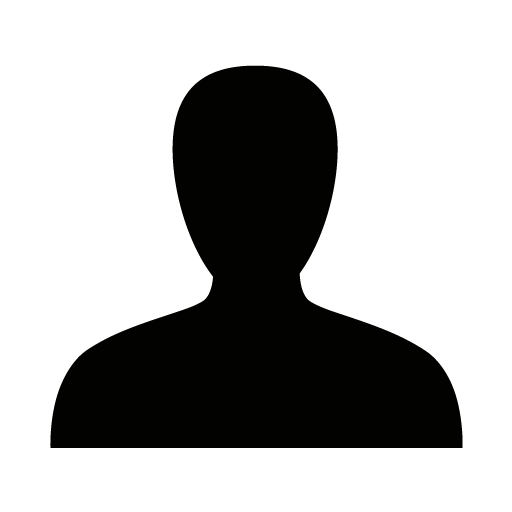
Two-dimensional layered metal-halide perovskites (2DLPs) are an emerging class of materials, characterized by a semiconducting metal-halide octahedral layer sandwiched between two layers of bulky organic cations. This unique structural arrangement allows for high in-plane mobility of excitons and charge carriers, while the predominantly insulating nature of the organic cations restricts out-of-plane mobility [1], [2]. The latter prevents studies of charge and energy transfer processes in vertical heterostructures. On the other hand, lateral heterostructures, where compositional changes occur along the in-plane direction, offer an interesting platform to explore potential charge transfer mechanisms at the junction interface [3].
To improve the accessibility and quality of heterostructures in 2DLPs, we developed a one-pot synthesis strategy for preparing lateral heterostructures that consist of different halide compositions. Compared to our previous solution-based anion exchange method [4], the presented approach produces consistently heterostructures with high-quality crystalline interfaces between the Br-rich core and the I-rich frame. We systematically investigated the effects of antisolvent, concentration, injection rates, and the Br-to-I ratio. Interestingly, when the iodine content in the precursor solution is increased, the core is eventually consumed, resulting in an I-rich frame-only structure. We attribute this to a combination of partial ion migration on top of the stepwise crystallization process. Additionally, we extended this concept to 2DLPs heterostructures featuring different metal cations including lead-free heterostructure.
The formation of heterojunctions within the in-plane semiconducting layer offers the potential to direct charge carriers or energy flow toward either the edges or the center of these microstructures, which is appealing for applications in energy harvesting and photocatalysis.
1.1-O2
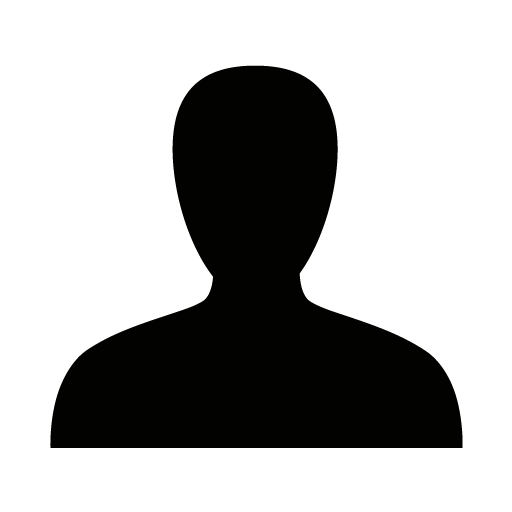
Two-dimensional layered lead-halide perovskites (2DLHPs) are currently in the spotlight for their high photoluminescence quantum yield and large structural and functional variety. Their layered structure gives them unique optoelectronic properties such as strong quantum and dielectric confinement[1]. This makes them highly promising for photonic and optoelectronic applications, and in that respect, achieving precise control over the quality and morphology of the synthesized crystals is crucial for unlocking their full potential.
Here, we developed a solvent-antisolvent recrystallization method to fabricate high-quality 2DLHPs microcrystals (MCs) with flat, nearly step-free surfaces. Our approach involves dissolving pre-synthesized 2DLHPs powder[2] and fine-tuning of parameters such as solvent/antisolvent choice and ratio, temperature, and saturation conditions. By optimizing these factors, we gained control over their lateral dimensions and thickness, and consistently produced high-quality MCs[3] on various substrates, including glass, silicon oxide, and gold, as well as on functionalized or pre-patterned areas. The compatibility with diverse substrates broadens the applicability of our MCs, offering seamless integration into devices. Moreover, our protocol demonstrates adaptability to multiple 2DLHP powders, broadening its applicability across different material systems.
The high structural quality and low defect density of the resulting MCs make them particularly suitable for photonic and optoelectronic applications, including light-emitting devices, waveguides, and quantum optics[4]. Our results represent a significant advancement in the fabrication of 2DLHP MCs, opening promising prospects for future integrations of this material.
1.2-O1
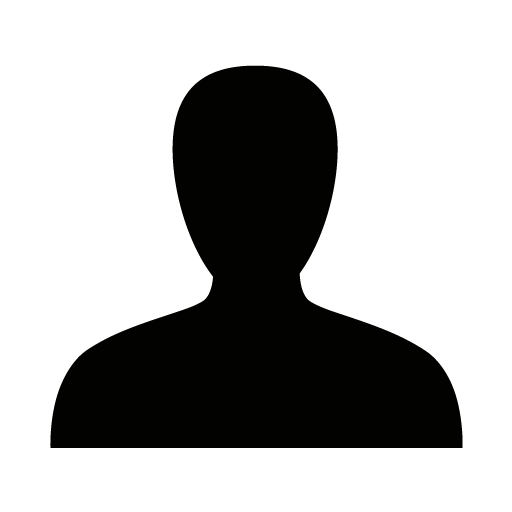
Hybrid organic-inorganic perovskites (PVKs) have attracted large interest due to their chemical variability, structural diversity and favourable physical properties [1].
In this work, a new thermochromic composite based on 2D PVKs is presented. Our material is able to switch reversibly from a transparent state (transmittance > 80%) at room temperature to a coloured state (transmittance < 10%) at high temperatures. The process occurs very quickly, requiring only a few seconds for the transition between the bleached and coloured states, and vice versa. Analyses conducted by X-ray diffraction, Fourier-transform infrared spectroscopy, differential scanning calorimetry, rheological and optical measurements during heating and cooling cycles showed that the thermochromic phenomena is based on a reversible disassembly/assembly of PVKs, mediated by polymer chains intercalation. This mechanism occurs through the formation and breaking of hydrogen bonds between the polymer and perovskite [2].
By varying the type and concentration of the organic cations in the formulation, we can regulate the interaction between polymer and perovskite, modulating the switching temperature and kinetics.
This work demonstrates a novel potential of perovskite-based composite, paving the way for their applications in thermoresponsive devices.
1.2-I1
Laura Herz is a Professor of Physics at the University of Oxford. She received her PhD in Physics from the University of Cambridge in 2002 and was a Research Fellow at St John's College Cambridge from 2001 - 2003 after which she moved to Oxford. Her research interests lie in the area of organic and organic/inorganic hybrid semiconductors including aspects such as self-assembly, nano-scale effects, energy-transfer and light-harvesting for solar energy conversion.
A plethora of new semiconductors have recently emerged as versatile materials for solar cells and photocatalytic applications. Combinatorial analytical probes have played a pivotal role in uncovering the mechanisms underpinning light-harvesting performance even before device optimisation has been attempted.
Ultrafast optical probes of photoconductivity dynamics are particularly useful here, uncovering the generation, localisation and ultimate recombination of charge carriers following photon absorption. We report on a peculiar ultrafast self-localisation process observed across wide classes of new bismuth-based semiconductors, including bismuth halides and chalcogenides. We have most recently shown such dynamic transitions from large to small polaronic states to dominate the dynamics of charge carriers in Cs2AgSbxBi1–xBr6 double perovskites,[1] (AgI)x(BiI3)y Rudorffites,[2] and AgBiS2 nanocrystals[3] and discuss the influence of alloying, cation disorder and stoichiometry on such charge-carrier localization events.
Probing charge-carrier motion in highly anisotropic semiconductors poses particular challenges. We show how such charge transport can be probed successfully in layered, two-dimensional (2D) metal halide perovskites that have been found to improve the stability of metal halide perovskite thin films and devices. We show that the 2D perovskites PEA2PbI4 and BA2PbI4 exhibits an excellent in-plane mobilities and exhibit unexpectedly high densities of sustained populations of free charge carriers, surpassing the Saha equation predictions even at low temperature.[4] In addition, we examine the effects of the high anisotropy of transport in thin films comprising layers that are highly oriented either parallel or perpendicular to the substrate plane.[5] We further demonstrate a powerful technique to the degree of transport anisotropy in these materials.
1.2-I2
Lorenzo obtained his PhD in Chemistry in 2003 and since 2008 is Assistant Professor at the Chemistry Department of the University of Pavia. In 2021 he was appointed Full Professor in the same department. He was the recipient of the Young Scientist Award for outstanding work in the field of perovskites at the International Conference on Perovskites held in late 2005 in Zürich, of the “Alfredo di Braccio” Prize for Chemistry 2008 of Accademia Nazionale dei Lincei awarded to distinguished under 35-year-old chemists and contributed the Journal Materials Chemistry and Chemical Communications“Emerging Investigator” issues in 2010 and 2011. He is working in several areas of solid state chemistry with particular interest in the investigation of structure–properties correlation in different kinds of functional materials, in particular electrolyte materials for clean energy, hybrid organic-inorganic perovskites and catalysis materials. He is author of more than 200 papers on international peer-reviewed journals. Since 2018 he is member of Academic Senate and Vice-Director of the Chemistry Department. He is Director of the INSTM Reference Center “PREMIO” devoted to the synthesis of innovative materials and member of the Directive Board of INSTM. Since 2014 he is member of the Academic Board of the PhD in Chemistry of Pavia University. He is Editor of Journal of Physics and Chemistry of Solids.
Chiral hybrid organic–inorganic metal halides, including low-dimensional perovskites, showing peculiar nonlinear optical and spin-dependent properties, are triggering a huge interest for their potential use in different applicative areas such as chiroptoelectronics and spintronics. In addition, their intrinsic noncentrosymmetric structure may be exploited in ferroelectric and piezoelectric devices. To date, the number of chiral systems is growing very quickly, and among the different structures reported, 2D chiral perovskites represent the vast majority. However, the structural family of chiral metal halides extends well beyond 2D perovskites and includes several 0D and 1D systems as well as 3D and quasi-2D motifs, which have been prepared using the commercially available chiral amines. As for other metal halide perovskites and low-dimensional systems, a wide range of tuning strategies can be put in place to modulate the (chiro)optical properties also going beyond Pb-based materials. In this presentation, we will show the recent progress of our group in the design of novel chiral metal halides making use of: i) chiral cation modulation (also considering ad-hoc synthesized cations); ii) modulation of B-site metal (i.e., Sn. Ge, Bi, Sb, Cu, Ni, Mn); and iii) halide substitution. The range of structural motifs arising by playing with "chemical degrees of freedom" is huge, providing also novel crystal structures not yet observed in current chiral (and achiral) metal halides. This work on the chemical modulation allows to understand which are the parameters mostly affecting the chiroptical properties of chiral metal halides thus paving the way for a rational design of novel materials [1-3].
1.2-O2
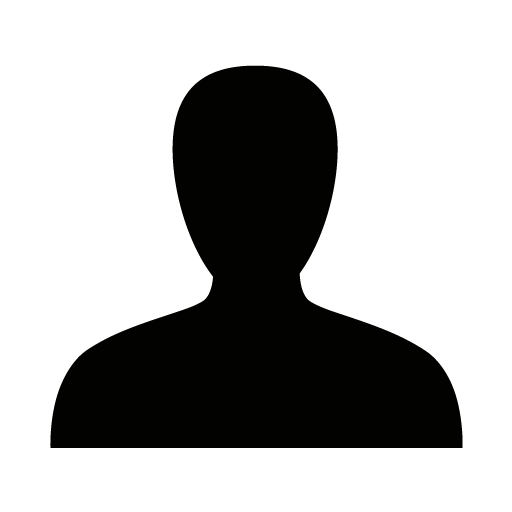
Chiral 2D double halide perovskites have become a new trend the past few years due to their lead-free character but also for their promising optoelectronic applications. In this context, we report new 2D double halide perovskite compounds, following the general chemical formula A(2 or 4)AgIMIIIBr8 where A is either the cystaminium dication (Cyst2+, chiral conformation) or the chiral cation (S/R)-1-(4-bromophenyl)ethylammonium (S/R-4BrMBA) and MIII = Sb3+, Bi3+. Cystaminium is known for its conformational axial chirality change in the solid state,[1] resulting in the synthesis of chiral perovskites and compounds which exhibit phase transition, leading to potential switchable SHG materials.[2] Driven by this objective, we synthesized and fully characterized the 2D (Cyst)2AgSbBr8 compound. DSC measurments showed that this compound exhibits three reversible phase transitions going from Phase 1 (Ph 1) to Phase 2 (Ph 2) (C2/c to C2/m, T = 40°C) upon heating, and upon cooling from Ph 2 to Phase 3 (Ph 3) (C2/m to C2, T = 60°C) and from Ph 3 back to the original compound at T < RT. Therefore, we performed temperature-dependent SHG measurements, highlighting the switchable SHG properties of such compound. The results of the measurement revealed that upon heating the transformation from Ph 1 to Ph 2 occurs via Ph 3 as a SHG signal is observed at 45°C. Upon cooling, the expected SHG signal originated by Ph 3 appears, with the maximum of it being at 11°C. (Figure 1a). The second spacer that we used was the chiral molecule S/R-4BrMBA. The effects of chirality in 2D lead halide perovskites is a newly grown field and based on the lack of chiral 2D double perovskites in the literature, a complete series of 2D bromide-based and iodide-based double perovskites was fully characterized. Thin films were prepared in order to study the chiroptical properties of the materials. The strong modulation of the structure and circular dichroism (CD) properties at the nanoscale is unprecedented, since the thin films of (S/R-4BrMBA)4AgBiBr8 strongly evolve from a single-phase compound with small intrinsic CD to a polymorphic material showing a strong increase in the chiroptical signal due to macroscopic effects, something which is not observed for the iodide derivatives (Figure 1b).[3] Such series of compounds will allow us to explore their chirality-induced spin selectivity (CISS) for both the fundamental understanding of the CISS effect and the practical aspect of preparing lead-free spintronic devices.
1.3-I1
Over the last decade, metal halide perovskite nanocrystals (LHP NCs) and their derivatives, in the form of nano and bulk crystals, have emerged as a promising class of semiconductor materials with many interesting linear and nonlinear optical properties.1 The light emission of LHP NCs is not only tunable by their dimensions and composition2 but also through self-assembly into ordered architectures.1-4 Interestingly, LHP NCs spontaneously self-assemble into superlattices and exhibit interesting optical properties such as polarized emission.5 On the other hand, low-dimensional metal halide crystals with chiral ligands exhibit chiroptical properties. This talk will be focused on the latest developments in achieving polarized absorption and emission from metal halide crystals either by self-assembly of NCs, using chiral filters, or helical structural engineering.
References
1. Dey et al., State of the Art and Prospects for Halide Perovskite Nanocrystals. ACS Nano 2021, 15 (7), 10775-10981
2. Otero-Martínez et al., Dimensionality Control of Inorganic and Hybrid Perovskite Nanocrystals by Reaction Temperature: From No-Confinement to 3D and 1D Quantum Confinement. Angew. Chem. Int. Ed. 2021, 60 (51), 26677-26684.
3. Vila-Liarte et al., Templated-Assembly of CsPbBr3 Perovskite Nanocrystals into 2D Photonic Supercrystals with Amplified Spontaneous Emission. Angew. Chem. Int. Ed. 2020, 59 (40), 17750-17756.
4. Gu et al. Color-Tunable Lead Halide Perovskite Single-Mode Chiral Microlasers with Exceptionally High glum. Nano Letters 2024. 24, 13333-13340
5. Mendoza‐Carreño et al. Nanoimprinted 2D‐chiral Perovskite Nanocrystal Metasurfaces for Circularly Polarized Photoluminescence. Adv. Mater. 2023, 35, 2210477
1.3-O1
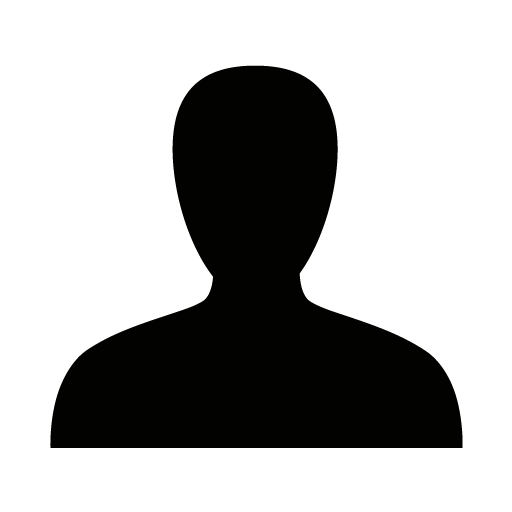
Perovskite single crystals have emerged as a promising alternative to polycrystalline samples in optoelectronics and photonics, owing to their exceptional properties such as reduced trap states, enhanced carrier mobilities, and extended diffusion lengths. Despite these advantages, their effective use in devices requires significant effort, particularly in developing specialized growth methods to produce structures with precise dimensions and geometries on a variety of substrates. This talk explores tailored growth strategies, including the capillary bridge and microfluidic-assisted approaches, which enable the synthesis of crystals with predefined shapes, sharp edges, and uniform surfaces.[1,2] By finely tuning growth conditions and controlling interactions within the precursor solution, these methods produce crystals with superior optical properties and performance, making them highly suitable for applications as waveguides and whispering gallery resonators. By achieving such well-defined features, perovskite single crystals demonstrate significant potential to address the specific demands of advanced optoelectronic and photonic devices, paving the way for innovative applications in these fields.
1.3-O2
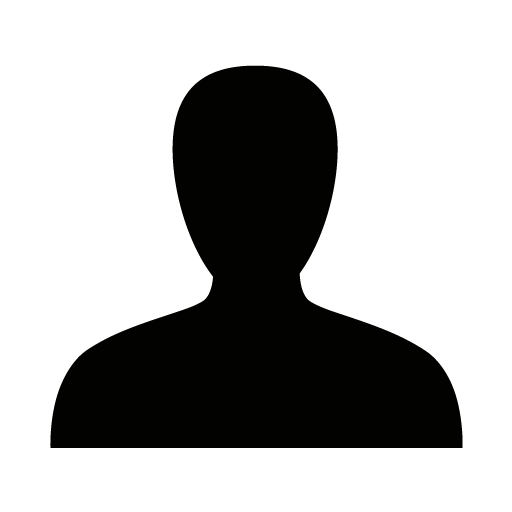
The integration of plasmonic nanoparticles (NPs) into perovskite-based optoelectronic devices offers transformative opportunities to significantly enhance the performance of both perovskite light-emitting diodes (PeLEDs) and perovskite solar cells (PSCs). Perovskite materials, renowned for their exceptional properties—including strong absorption, long carrier diffusion lengths, tunable bandgap, high quantum yield, and narrow emission profiles—are at the forefront of next-generation optoelectronics. However, their full potential remains untapped due to persistent challenges: PeLEDs require further optimization of quantum yield, color purity, and angular light control, amongst others, while PSCs, particularly tandem configurations with narrow-bandgap perovskites, face limitations in absorption capacity that hinder efficiency improvements.
Our research considers meticulous simulation to embed plasmonic NPs randomly distributed into perovskite structures (Figure 1), yielding significant enhancements in their optical properties. By optimizing parameters such as NP composition (Ag, Au, Cu), size, and volumetric concentration, we establish robust design principles to harness plasmonic resonances in optoelectronic devices [1-3].
Rigorous simulations based on the Finite-Difference Time-Domain (FDTD) method demonstrate a three-fold increase in photoluminescence from CsPbBr3 films embedded with spherical Ag NPs, in comparison to reference films without NPs. This NP design also enables precise control of light directionality, improving device performance across diverse applications. For solar cell applications, our modeling predicts substantial absorption enhancements in perovskite films containing plasmonic NPs. For all-perovskite tandem solar cells, we achieve a 2% absolute improvement in power conversion efficiency for Sn-based perovskites [4]. These gains stem from synergistic near- and far-field plasmonic effects, which also mitigate parasitic absorption and enable the use of thinner perovskite films. Thinner films enhance charge collection and reduce the amount of lead required, addressing both performance and environmental concerns.
We present recent experimental results demonstrating the stable combination of CsPbBr3 nanocrystals and plasmonic NPs in polar solvents [5]. This approach eliminates the need for encapsulation, allowing seamless integration of near- and far-field plasmonic effects, and expanding practical applications.
1.3-O3
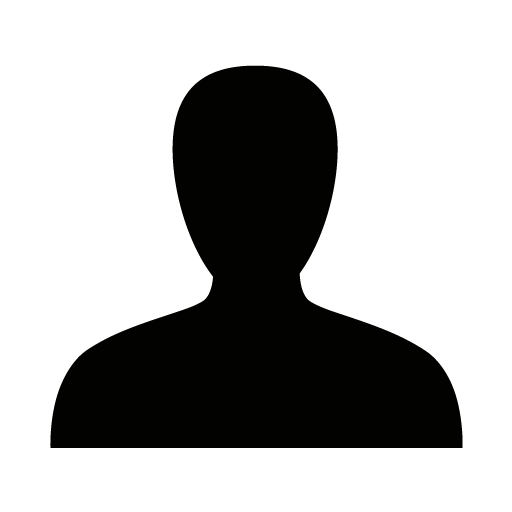
Nowadays, the existence of high-entropy perovskite oxides is well established, and since their discovery, their fields of application have been continuously studied. The main advantage of these materials, with the general formula ABO₃, lies in the high tunability of their properties through variations in chemical composition. However, the systematic study of their stability, fields of existence, and solubility limits remains underdeveloped compared to the extensive research on their applications, despite the availability of large datasets and computational studies. In our work, we investigated the structure and solubility limits of two families of perovskite oxides using a chemometric approach. We selected lanthanum as the A-site cation due to its stability, while for the B-site, we explored various cation mixtures based on Cr, Mn, Fe, Co, Ni, and Zn. Our goal was to determine the experimental domain by integrating diverse data, including crystal structure, oxygen vacancy content, temperature dependence, and composition. The synthesized samples were analyzed using x-ray diffraction (XRD) followed by Rietveld refinement to extract crystallographic parameters. Neutron diffraction experiments were also performed to obtain precise structural details, especially regarding oxygen positions and non-stoichiometry. Using multivariate analysis, we correlated elemental concentrations with phase stability, crystal symmetry, and cell parameters. This structural study aims to identify potential links between crystal symmetry and the catalytic properties of the materials. Additionally, thermogravimetric analysis was conducted to study non-stoichiometry and phase transitions, which were incorporated into the designated experimental domains. This comprehensive dataset will enable the identification of optimal compositions for desired applications, such as heterogeneous catalysis, solid-oxide fuel cells, and oxygen transport membranes.
2.1-O1
Grigorios Itskos obtained a B.Sc. in Physics in 1997 from University of Thessaloniki, Greece and carried out his PhD studies at SUNY at Buffalo, USA (Ph.D. in Physics 2003), under the supervision of Prof. Athos Petrou within the newly-born field of semiconductor spintronics. He worked as postdoctoral researcher (Imperial College London, 2003-2007) under the supervision of Profs. Donal Bradely and Ray Murray, focusing on photophysical studies of hybrid organic-inorganic semiconductors. In September 2007 he was hired as a faculty member at the Department of Physics, University of Cyprus (Lecturer 2007-2011, Assistant Professor 2011- 2017, Associate Professor 2017- now). His group research activities focus on optical studies of inorganic, organic and hybrid solution-processed semiconductors, with recent emphasis on the characterization and optoelectronic applications of semiconductor nanocrystals.
The Achilles heel of Sn-based perovskites is their susceptibility to Sn2+ to Sn4+ oxidation. Chemical instability becomes more severe in nanocrystal (NC) form due to the large surface area, while NC durability deteriorates further in the solid state because of higher ligand loss and greater exposure to humidity and oxygen. Recently, an optimized synthetic route was developed, allowing the production of robust and monodisperse three-dimensional (3D) CsSnI3 nanocrystals (NCs) that coexist with residual amounts of 2D Ruddlesden-Popper nanosheets (NSs) [1].
Herein, the exciton structure and stimulated emission properties of thin films of the tin iodide perovskite nanostructures are discussed. Incorporation of the CsSnI3 NCs in polystyrene matrixes, increases substantially the optical stability in the oxygen-free environment of the spectroscopic measurements. At ambient temperature, strong NC and weak NS excitonic bands in the red and yellow spectral range, respectively are observed and monitored by transient absorption and photoluminescence (PL). The transient decay of the bleaching of the ground exciton band and the NC luminescence is found to occur at timescales of the order of 0.1 and 1 ns respectively, being accelerated compared to the respective dynamics of lead-based perovskite NC films, most probably due to non-radiative recombination at surface defects. At cryogenic temperatures, two emissive species of the NC structures are observed, identified as bound and free excitonic complexes. The relative population of the two species depends on temperature, aging and surface passivation. Photoexcitation of the CsSnI3 NCs films with nanosecond pulses activates amplified spontaneous emission (ASE) at temperatures as high as 150 K. The ASE threshold and net modal gain is optimized via the fabrication of NC-polymer multilayer structures, allowing the observation of room temperature ASE.
2.1-I1
to be confirmed to be confirmed to be confirmed to be confirmed to be confirmed to be confirmed to be confirmed to be confirmed to be confirmed to be confirmed to be confirmed to be confirmed to be confirmed to be confirmed to be confirmed to be confirmed to be confirmed to be confirmed to be confirmed to be confirmed to be confirmed to be confirmed to be confirmed to be confirmed to be confirmed to be confirmed to be confirmed to be confirmed to be confirmed to be confirmed to be confirmed to be confirmed to be confirmed to be confirmed to be confirmed to be confirmed to be confirmed to be confirmed to be confirmed to be confirmed to be confirmed to be confirmed to be confirmed to be confirmed to be confirmed to be confirmed to be confirmed to be confirmed to be confirmed to be confirmed
2.1-I2
Double perovskites and chalcogenide perovskites: variations on the theme.
Lead halide perovskites are photovoltaic absorbers with excellent optoelectronic properties and impressive efficiencies. However, their instability in air and moisture, and the presence of lead (which raises health concerns), pushed the research community for searching for alternatives. Lead-free perovskites have therefore attracted the researchers’ interest, thanks to their varied compositions and diverse properties. In this talk, two different types of lead-free perovskites will be discussed: double perovskites and chalcogenide perovskites. Double perovskites are obtained through heterovalent substitution of Pb2+ with a mono and trivalent cation, with a resulting “doubling” of the structure. Cs2AgBiBr6, the representative of this family, presents excellent stability in air atmosphere, but low solar cell. On the other hand, chalcogenide perovskites present the traditional perovskite ABX3 structure but, being X=S2-, the cations have higher valency (such as Ba2+ and Zr4+ in BaZrS3). Even if these chalcogenide perovskites have promising optoelectronic features, their synthesis is challenging, resulting in little characterization available and no devices reported.
In this talk, I will present these materials, the reasons behind the discussed limitations and possible strategies to overcome them.
2.1-O2
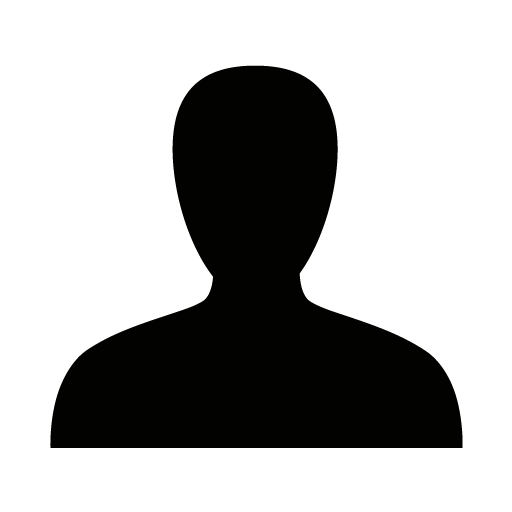
Perovskite solar cells (PSCs) show great promise due to their high efficiency and low manufacturing costs, yet they encounter notable challenges such as optical losses and stability issues, mainly due to high surface reflection and degradation from UV irradiation, and heat[1-3]. In this study, we present a novel, bio-based composite material comprising pectin, polymethyl methacrylate (PMMA), and a spirobifluorene compound designed to mitigate these issues. This innovative composite exhibits high optical transparency, up to 85%, and significant haze (48% at 550 nm), which helps in minimizing reflection-induced losses. The composite incorporates spirobifluorene, which facilitates down-conversion of UV radiation around 350 nm to higher wavelengths above 400 nm, thereby enhancing both photostability and overall device performance. This material, with its lower thermal conductivity compared to glass, also cools the solar cell surface by serving as a thermal barrier. This composite was subsequently attached to the front side of PSCs, which were structured in an inverse architecture (ITO/Me4pacz/SiOx/PSK/C60/SnOx/Cu) for performance measurements and in a different configuration (FTO/c-TiO/m-TiO/PSK/spiro-meotad/gold) for stability tests ( see Figure 1). The composite's implementation leads to up to a 5 ±0.1% increase in the current density and power conversion efficiency of perovskite solar cells. Additionally, it significantly delays initial photodegradation, enhancing T80 life by 1.1-fold for PP-0.25TSBF and 1.9-fold for PP-0.75TSBF. These advancements highlight the potential of this innovative composite to significantly improve the efficiency, stability, and durability of perovskite solar cells, offering a promising route for future photovoltaic technologies.
2.2-O1
Heavy-metal chalcohalides are inorganic semiconductors [1], with potential as next-generation optoelectronic materials based on earth-abundant elements. Compared to other semiconductors, usually obtained through colloidal synthesis, chalcohalides combine the simple chemistry of metal halides like CsPbBr3 with the improved stability of metal chalcogenides like CdS, making them ideal candidates to be explored in the form of nanocrystals. Among them, bismuth-based compounds [2],[3] are particularly promising because of their low toxicity and the high absorption cross-sections, which makes them optimal choices for light harvesting applications. Despite these potential advantages, however, these materials have been explored relatively sparsely so far and primarily in the form of bulk or microcrystals [4].
Herein, a new synthetic route to obtain metallic Bi/Bi13S18Br2 colloidal nano-heterostructures with dumbbell morphology is presented. This one-pot procedure was developed by modifying the synthesis of Bi-S-X nanocrystals [5] with the controlled introduction of a tertiary amine, which acts as a mild reducing agent. This induces an in-situ nucleation of the metallic bismuth domains on the surface of the semiconductor. By combining electron microscopy and X-ray diffraction we elucidated the non-trivial growth mechanism of these heterostructures, which proceeds via dual nucleation event (first Bi13S18Br2 and then metallic-Bi on its surface) followed by a controlled deposition of material at the interface between the two domains.
The combination of a semiconducting and a metallic domain makes our Bi/Bi13S18Br2 heterostructures interesting materials for photocatalytic applications, as the presence of a heterojunction is expected to enhance the separation of photogenerated carriers and increase their availability for promoting chemical reactions [6]. To assess the photocatalytic activity of these heterostructures, we tested them for the photodegradation of organic dyes like Rhodamine B and Methylene Blue as a proof of concept. Our tests revealed that the Bi/Bi13S18Br2 heterostructures can efficiently photodegrade the dyes without relevant structural, morphological, and compositional modification for several reuse cycles, demonstrating excellent robustness and stability in polar solvents, like ethanol. The mechanism of photocatalysis will be also discussed. These promising results motivated us to pursue a more in-depth exploration of these and similar heterostructures for photocatalytic applications, which is currently ongoing.
2.2-I1
The advent of metal halide perovskite has revolutionised the field of optics and optoelectronics. In particular, the high efficiency of solar cells demonstrated at laboratory scale, now exceeding 26% [1], has stimulated the interest of both researchers and industry in photovoltaic applications.
In this context, the processing of perovskite materials from solution is very attractive, since large amounts of material can be deposited using high-throughput roll-to-roll deposition techniques [2]. Nonetheless, given the strict correlation between the properties of perovskite precursor solutions and the formation of a perovskite film with the desired properties, [3-4] the composition of the ink and the use of additives have been shown to be a crucial aspect in the development of metal halide perovskites with good optoelectronic quality.
In this talk, I will first give an overview of how the incorporation of polymers as additives in precursor inks can afford control over the crystallisation process of metal halide perovskite materials, which is a key aspect for reproducible fabrication of robust films. In particular, I will show how the polymer can influence the stability, mechanical properties and processability of metal halide perovskites that can eventually be printed via roll-to-roll. [5-8]
Finally, I will give an insight into novel functionalities that can be imparted to metal halide perovskites by a judicious selection of polymer matrix, namely the thermochromism and the printability via additive manufacturing techniques for the development of plastic scintillators. [9-10]
2.2-I2
The presence of mobile ions in metal halide perovskite materials and charge transport layers have been shown to adversely affect the efficiency, hysteresis and stability of perovskite solar cells (PSCs). Li-TFSI doped Spiro-OMeTAD is the most used hole transport material in n-i-p perovskite solar cells. In the process of device preparation, Li+ ions diffuse on the surface of metal electrode, which promotes the oxidation of Spiro-OMeTAD, improves the conductivity of Spiro-OMeTAD. Meanwhile, it is accompanied by the migration of Li+ into the cells and enrichment at the perovskite/SnO2 interface, which promote the extraction efficiency of electrons. [J. Mater. Chem. A. 2021, 9,7575–7585.] However, during the operation of the device, the migration of Li+ in SnO2 will cause hysteresis and "burn-in" degradation. By introducing a thin layer of cross-linked fullerene (CL-PCBM) at the SnO2/perovskite interface, the "burn-in" degradation can be suppressed. It was revealed that CL-PCBM can fix Li+ ions in the SnO2/perovskite interface, and the introduction of CL-PCBM can increase the built-in potential of the device and improve the electron extraction efficiency. Finally, the power conversion efficiency of 24.19% was achieved, and the "burn-in" degradation process was also eliminated. [Adv. Mater. 2023, 35, 2207656; Adv. Energy Mater.,2023, 2301161]
1.2-I1
Organic semiconductors are an emerging class of materials with various optoelectronic applications and huge potential in supporting the development of greener electronics.In my talk, I will introduce sustainable routes to manufacture solution-processed organic photovoltaics. Most organic electronic devices are fabricated from halogenated and non-halogenated aromatic solvents, primarily due to the good solubility of the materials in the solvents and the ideal microstructure/crystallinity they are forming in those. For large-scale production and further commercialisation, this is a key limitation, as they can be carcinogenic or toxic to the human reproductive systems and negatively impact the environment. Here, I will show high-performing organic solar cells developed from novel, more sustainable organic semiconductors (in terms of less waste during synthesis and less energy consumption) as well as eco-friendly solvents. In particular, solvents derived from biomass have been explored for their application in delivering high-performing organic photovoltaics based on PTQ10:Y12 and FO6-T:Y12 with PCE > 14%.
Finally, I will discuss possible degradation mechanisms of the bulk heterojunction systems containing Y6 or Y12 as the acceptor, after degradation with indoor and outdoor light. A combination of electrical, morphological, and spectroscopic techniques were used to evaluate the degradation pathways of the OPVs.
1.2-I2
The rapid expansion of the Internet-of-Things (IoT) is driving the demand for energy-autonomous devices. With billions of wireless sensors expected to be installed in indoor environments over the next decade, battery usage in IoT is growing, leading to higher management costs and waste. Indoor photovoltaics (IPVs) offer a sustainable power solution by reducing battery waste. [1] Air-stable perovskite-inspired materials (PIMs) are promising low-toxicity semiconductors with wide bandgaps (1.8–2.0 eV) for efficient indoor light harvesting, with theoretical IPV efficiencies approaching 40% or more. However, the inherent low-dimensional nature of PIMs and high defect densities pose challenges, such as carrier localization, in achieving very high IPV efficiencies. [2]
In this talk, I will present our recent results on two-dimensional PIMs comprising Group VA pnictogen cations, such as antimony (III) (Sb3+) and bismuth (III) (Bi3+). Through thorough compositional engineering at each crystallographic site (A, B, X) of the PIM structure, we achieved the highest indoor power conversion efficiency for IPVs based on halide PIMs. [3-5] Notably, the IPV devices of Cs2AgBi2I9 PIM maintained consistent performance under different light color temperatures, demonstrating the versatility of Cs2AgBi2I9 as a reliable IPV absorber in various indoor environments. Our research paves the way for sustainable indoor light harvesting by identifying or developing 2D pnictogen-based PIMs with adaptable structural and photophysical properties.
1.2-O1
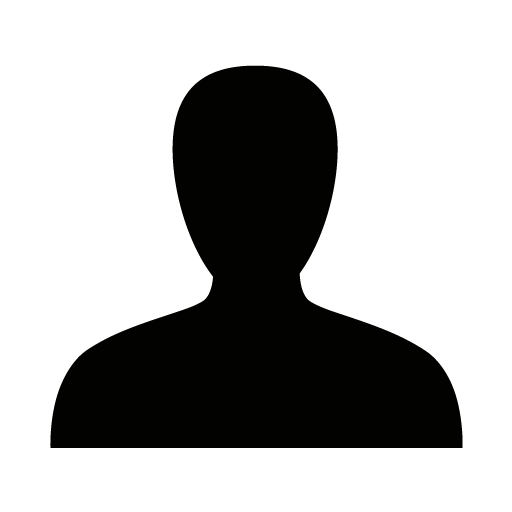
The potential of perovskite-based photovoltaics is rapidly becoming recognised as a probable and significant contributor towards attaining climate neutrality by 2050. Indeed, perovskites highly tuneable optoelectronic properties coupled to its facile, low-cost and scalable fabrication allow for a wide variety of innovative applications such as tandem configurations (silicon-perovskite or perovskite-perovskite), space applications due to their high power-to-weight ratio and even indoor light harvesting. [1] Whilst most of the attention is being put towards maximizing performance, critical sustainability aspects are often being overlooked. Indeed, the sustainability of these devices should also be at the forefront of development in order for this emerging technology to be commercialized and accepted by the market, allowing for its widespread deployment.
In the last 10 years, Perovskite Solar Cells (PSCs) have experienced a meteoritic rise in performance, arriving most recently at 26.1% power conversion efficiency, (PCE) directly competing with silicon-based photovoltaics. [2] Nevertheless, comprehensive solutions on reducing the environmental impact of the device are still lacking in literature. More specifically, high performing devices are still manufactured with materials that require, out of necessity, toxic and environmentally damaging solvents that could pose a threat towards the ambient-air, large-scale (e.g. Roll-to-Roll) manufacturing aspects. [3] Furthermore, the use of critical raw materials [4] is still highly favoured (i.e dopants), discouraging the technology impact at a larger scale. Finally, materials employed in the cell (e.g. organic hole transport layers (HTL)) are usually very expensive due to their lengthy and inefficient synthetic and purification procedures, resulting in no technology readiness levels (TRL) associated for TW levels of production. [5]
To help fill the research gap between sustainability and performance, we developed an array of cheap, novel HTL layers, based on the state-of-the-art poly(triarylamine) (PTAA) [6], that can be processable in “greener” solvents. This was achieved by the modification of the triphenylamine polymeric backbone via the incorporation of a phenothiazine organic scaffold, which shows good solubility in common organic solvents. [7] Additionally, the methyl substitution of the TPA phenyl unit was modified to evaluate the trade-off effect on solubility and performance. An additional benzothiadiazole unit was also included due to its promising nature in organic semiconductors. [8]
The resulting polymers presented good solubility in our proposed, more sustainable solvent: tetrahydrofuran (THF) that is non-aromatic, non-halogenated, cheap, has a low environmental risk and a low human health toxicity. [9] A full structural, optoelectronic, and thermal characterization resulted in polymeric compounds P1-4 that displayed suitable HTL properties. These were then applied to flexible n-i-p devices with PTAA as the reference. One of the polymers (P1) revealed competitive efficiencies when PTAA is deposited using toluene (conventionally used in literature) and even outperforms the reference when processed with THF. P1 also displayed a remarkable enhancement in unencapsulated light soaking stability, with respect to PTAA devices. A solid-state film analysis allowed us to determine that the synthetic tailoring of structural components is key for performance enhancement.
Following on from this, a doping optimization was also conducted using multi-variate analysis approach (i.e. Design of Experiment), aiming at obtaining the right combination of HTL and dopant concentrations, resulting in enhanced PCEs with a better efficacy in material use. Finally, a thoughtful revisit of the synthetic protocols was considered targeting innovation and more eco-friendly processes. In fact, we were able to substitute conventional organic solvent procedures that are lengthy, generate waste, typically low to moderate yielding and employ high temperatures, to protocols employing more sustainable and recyclable mediums (i.e. water), permitting for reactions that are very high yielding, selective, fast and above all, scalable, simple, low-cost and can progress to higher TRL levels.
1.2-O2
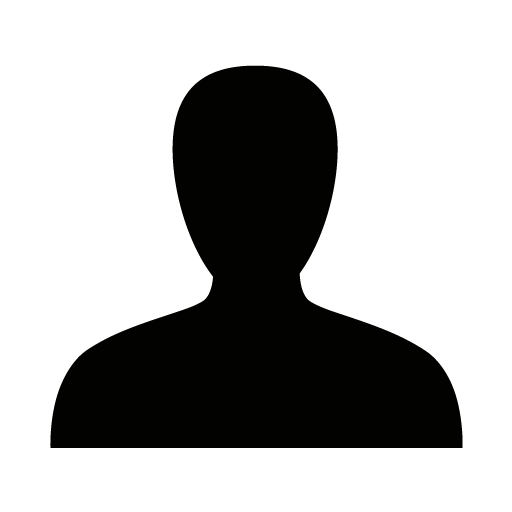
Indoor photovoltaics (IPV) is increasing the scientific interest due to the expected internet of things (IoT) market increase, doubling its size by 2030. IPV can be used as a sustainable energy supply for sensors and low consumption applications, as an alternative to batteries. Thus, reducing the need of replacement and the amount of waste generated along the lifetime of the devices. Research is ongoing to improve relevant materials and devices for this application, focusing in indoor (inside of buildings) environments. In this sense, organic photovoltaics (OPV) is an interesting alternative due to the bandgap tuning of these materials, their high absorption in thin layers and their good performances (>30%[1,2]) already demonstrated under low illumination. Furthermore, the possibility of using more sustainable processes: printing in air with short high temperature steps; meaning lower energy consumption and cost than other PV technologies. Positioning IOPV as an alternative for this application.
IOPV creates a new field of application with strong differences compared to outdoor applications. First, illumination in indoor is based on fluorescent or LED lamps with different color temperatures, so absorption needs to be tuned to the visible region considering the emission spectra of those different lamps. Second, devices need to be performant at low illumination (200-1000lux). These requirements need device optimizations using material choices that reduce defect-driven recombination in the different layers of the devices. Meaning that, in order to improve their performance (PCE) at low illuminances, a high open-circuit current and low shunt resistance are required. Further, a good compromise of low series resistance (depending on the device behavior at low illumination) is required. Finally, stress factors in indoor operation conditions are milder than outdoor, being a favorable scenario for the use of OPV devices. However, research is still needed to investigate the stability of indoor cells and modules [3].
In the IOPV-Lab, the common laboratory between CINaM, IM2NP and the company Dracula Technologies we are working on an OPV blend system based in non-halogenated solvents processed in air with PCEs over 20% at low illumination. We are also studying the ageing behavior of this system, trying to understand the impact of cosolvent and additives utilization on their stability under combined indoor conditions. This study may allow us to understand if the devices are stable under operation for IoT applications in the long term. First tests seem to show that the most performant formulation is not stable after ageing, so a detailed study is ongoing to proof the formulation variability on the stability of the device. Opening up an alternative non-halogenated formulation that represents a suitable option for IOPV devices
IOPV samples have been fabricated in air by doctor-blade coating (with a final evaporation step), as scalable technique for industrial transfer. Samples have been characterized in different indoor conditions and illumination ranges before and after encapsulation. Then, an “indoor ageing test”, which includes temperature, humidity and indoor lighting conditions, is ongoing to check the interaction of the different stress-factors under real degradation conditions. Further, different optical (such as absorbance), opto-electronical (IV, EQE, LBIC, …) and morphological characterization methods are used at different times of the ageing test to understand the stability behavior of the different sample types. Results are expected to give us an overview of the best combination of solvent/cosolvent + additive in terms of stability.
The oral presentation expects to give an overview about indoor applications field and to present the best results of the studied system. It will include the stability using different non-halogenated formulations and using scalable techniques. Thus, providing valuable information of the real feasibility of IOPV devices use for indoor-PV applications.
1.3-O1
We present an isolated plasma soft deposition (IPSD) technique for the plasma damage-free deposition of an amorphous InGaTiO (IGTO) top cathode on semi-transparent perovskite solar cells (PSCs). Unlike conventional DC or RF magnetron sputtering, where plasma directly faces the target, the IPSD process utilizes an isolated plasma region that prevents plasma irradiation, ensuring the deposition of the IGTO cathode without damaging the soft perovskite active layer. The confinement of high-density plasma in isolated regions, achieved through a Nd-Fe-B45 magnet array, further protects the active layer by minimizing the impact of energetic particles from the sputtered IGTO targets. Additionally, linear scanning of the glass substrate within this isolated plasma region enables low-temperature, large-area IGTO deposition. Under optimal conditions, the IPSD-processed IGTO film, with a thickness of 150 nm, exhibited a low sheet resistance of 31 Ω/square, high average optical transmittance of 91.41%, and a work function of 4.1–4.5 eV. To demonstrate the feasibility of this technique, we fabricated semi-transparent PSCs with spin-coated perovskite layers. The PSCs with IPSD-processed IGTO cathodes achieved a power conversion efficiency (PCE) of 17.52%, comparable to the 4.35% PCE of PSCs with magnetron-sputtered ITO cathodes, due to the absence of plasma-induced damage. Furthermore, we successfully integrated the IPSD technique into the fabrication of PSC and Si-based tandem solar cells, achieving PCEs ranging from 24% to 28%. These results highlight the significant potential of the IPSD-based deposition process as a key technology for the commercialization of semi-transparent PSCs and tandem solar cells.
1.3-I1
To achieve higher electricity generation at a low cost, the most substantial impact on the energy generation from solar cells is to make existing technologies more efficient per area. This forms the focus of tandem solar cells, which utilize different photoactive materials with different bandgaps, allowing benefiting from a broader range of the solar spectrum. Recent advances in perovskites have dramatically improved their efficiency, with tandem devices—particularly those using silicon bottom cells—showing great potential for further performance enhancement. However, the phase instability of wide-bandgap perovskite top cells under prolonged operational conditions, including exposure to light and heat, poses a significant challenge. To solve the issue, different approaches will be discussed; grain boundary, bulk of perovskite, and the interfaces with charge transport layers. For example, recently, we addressed this by improving cation interactions within the perovskite lattice, enhancing phase stability under light and heat. Furthermore, improving the optical properties and reducing recombination losses has been key to achieving a certified efficiency of 33.7% in perovskite-silicon tandem cells, demonstrating both enhanced performance and operational durability. I will discuss how we are addressing these challenges, utilizing optical spectroscopy and comprehensive analysis techniques.
1.3-I2
Erkan Aydın is leading the “Aydin Group” in the Chemistry Department of LMU Munich. His research group is focusing on the development of “realistic ultra-efficient tandem photovoltaic solutions for earth and space applications“ by currently prioritizing multijunction solar cells, specifically perovskite/silicon tandem solar cells and all-perovskite tandem solar cells. Erkan Aydın obtained his PhD (2016) degree from the Micro and Nanotechnology Program at TOBB ETU (University of Economics and Technology) in 2016 and he pursued his postdoctoral research at the KAUST for seven and half years before establishing his team at LMU Munich. His extensive work at KAUST led to several record-breaking efficiencies in perovskite-silicon tandem solar cells. Erkan Aydın is the principal investigator of the INPERSPACE ERC StG Project.
Over the past decade, substantial strides have been made in enhancing the performance of tandem cells, culminating in dual junction configurations achieving PCEs nearing 34%, marked by various record-breaking updates.[1] In 2023, our research contributed significantly to this progress, unveiling three PCE records of 32.5%, 33.2%, and 33.7%.[2] These achievements were made possible by introducing solution-processed perovskites on micron-sized pyramidally textured c-Si bottom cells and a series of improvements at the interfaces and the bulk of the perovskite. As for the interfaces, we had to solve several issues, such as introducing a dielectric interlayer between perovskite and fullerene contacts to mitigate induced defect states [3], enhancing the recombination junction through ultrathin indium zinc oxide electrodes, introducing alternative hole selective contacts including polymers,[4] nickel oxide,[5] and self-assembled monolayers, and using alternative transparent electrodes. Each step of these advancements and their corresponding stability assessments revealed the pivotal role of interfaces. We also discovered that interfaces play a crucial role even during the encapsulation process, as thermomechanical stresses drive the degradation of solar cells, making interfacial strengths critically important. Furthermore, from a sustainability perspective, reducing the use of critical elements on the contacts is essential. To address this, we minimized the use of indium in the transparent electrodes of our tandem solar cells, thereby enhancing light coupling in the devices and making the process more sustainable [6]. Despite the remarkable achievements in perovskite-silicon tandem solar cells, their use in space remains a concern due to the radiation sensitivity of the silicon heterojunction bottom cells, while other extremes are still yet to be demonstrated. In this invited talk, I will present our systematic solutions to solve stability issues in dual junction tandem solar cells, along with insights into their potential for space applications.
1.3-O2
Luigi Angelo Castriotta is a post-Doctoral fellow from the University of Rome Tor Vergata, focusing on flexible perovskite solar cells and modules. He joined Prof. Huang's group at UNC (USA) in June 2023, as a Global Marie-Curie Post-Doctoral Fellow and as a Principal Investigator of the "EFESO" Project. He got his Ph.D. in Electronics Engineering in 2021 from University of Rome Tor Vergata (Italy) as a Marie-Curie Fellow as part of the Innovative Training Network MAESTRO; He did his bachelor’s degree in chemistry at University of Rome Tor Vergata (Italy) and Masters’ in "Nanoscience and Nanotechnology" at Universitat de Barcelona (Spain) and in "Organic Molecular Electronics" at Technische Universitat Dresden (Germany).
The field of photovoltaic (PV) technologies is undergoing a rapid transformation, driven by the demand for high efficiency, cost-effective, and scalable solutions. Emerging PV materials, including perovskites, organic photovoltaics (OPVs), and tandem architectures, are pushing the boundaries of efficiency and stability, with record-breaking power conversion efficiencies (PCE) now at 27% for single-junction perovskites and surpassing 34% in perovskite-silicon tandems. The industry is shifting towards scalable fabrication techniques such as blade coating, slot-die printing, and vapor deposition to enable large-area, high-throughput production while maintaining performance. A key focus in the field is the improvement of long-term stability, as operational lifetimes continue to be a primary barrier to commercialization. Advances in encapsulation, interfacial engineering, and composition tuning have significantly enhanced the durability of next-generation PVs, with stability benchmarks extending beyond 1,000 hours under accelerated aging tests. Additionally, flexible and lightweight PVs are gaining momentum, enabling applications in portable electronics, wearable technology, and building-integrated photovoltaics (BIPV). Mechanical resilience, particularly in thin-film and flexible devices, is becoming a crucial parameter, alongside efficiency and environmental stability. The integration of artificial intelligence (AI) and automation in material discovery and device optimization is also accelerating innovation, allowing for rapid screening of new compositions and processing conditions. As research continues to bridge the gap between laboratory-scale performance and real-world implementation, the standardization of testing methodologies and upscaling strategies will be critical in determining the commercial viability of emerging PVs. The ongoing advancements in materials science, device engineering, and manufacturing technologies position photovoltaics at the forefront of the renewable energy transition, paving the way for next-generation solar energy solutions.
1.3-O3
Flexible perovskite solar cells (f-PSCs) have the potential to revolutionize various applications, including IoT, portable and wearable electronics, and space technology, where flexibility, conformability, lightweight design, and a high power-to-weight ratio are highly desirable. In addition to their impressive efficiency (reaching up to 25%[1]) f-PSCs offer several other advantages: they are fabricated using abundant materials through cost-effective solution-based processes [2]. However, even more than their rigid glass counterparts, the long-term stability of f-PSCs remains a challenge due to both intrinsic and extrinsic factors [3]. Effective encapsulation is crucial for ensuring durability by protecting the devices from external elements such as moisture, water, and oxygen, without compromising their flexibility. Thermosetting polyurethanes (PUs) emerge as promising encapsulants due to their chemical inertness toward the perovskite layer and their ability to cure at room temperature directly on the PSC [4]. Additionally, minor structural modifications in PU precursors can enhance flexibility, barrier properties, and transparency. This study demonstrates the successful use of a low-cost thermosetting PU resin for encapsulating 1 cm² f-PSCs. Two encapsulation approaches were explored: one where PU is applied solely to the back (in contact with the metal electrode) and another where PU is applied to both the back and the front (on the PET substrate). While maintaining device flexibility as proved by bending tests, the dual-layer encapsulation strategy significantly improves stability under high humidity conditions (relative humidity >75%), achieving a T80 lifetime of over 550 hours (23 days). This represents a substantial improvement compared to unencapsulated devices, which exhibit a T80 of only 6 hours.
2.1-I1
Dr. Clara Aranda Alonso, received her doctorate degree in Science from University Jaume I in 2019 at the Institute of Advanced Materials (INAM) (Castellón, Spain). She worked as postdoctoral researcher at the Forschungszentrum Jülich and Institute for Photovoltaics (ipv) at the University of Stuttgart (Germany) for two years. Then she moved to the Institute of Materials Science (ICMUV) at the University of Valencia (Spain) as a Margarita Salas fellow. Currently, she is working at Universidad Pablo de Olavide in Seville (Spain). Her work is focused on the synthesis and characterization of wide band gap perovskite materials, both in thin film and single crystal configuration, for photoconversion devices such as solar cells, photodetectors and memristors, using impedance spectroscopy as the main characterization tool.
Perovskites face significant stability challenges arising from both extrinsic factors like humidity and intrinsic issues linked to their ionic nature. These stability limitations hinder the long-term performance of perovskite-based devices. Mastering crystallization processes, whether in thin films or bulk, is a critical step in overcoming these obstacles. High-quality, highly crystalline materials are essential not only for enhanced stability but also for reliable characterization, which is key to understanding the mechanisms governing device operation.
Monocrystalline perovskites offer a compelling alternative to conventional thin films, benefiting from the absence of grain boundaries and their associated defects. Achieving these high-quality materials demands precise control over synthesis methods to improve both material properties and stability.
In this contribution, we will explore diverse synthesis approaches for perovskite single crystals, including the widely used inverse temperature crystallization (ITC) with seed-assisted growth, adaptations for multi-halide-core crystals and confined-growth methods. We will also introduce two innovative techniques: a continuous-flow reactor for large-scale crystal growth and a novel dry synthesis methodology for narrow-bandgap perovskites.
Furthermore, we will present advanced and non-conventional characterization techniques applicable to these materials, both as stand-alone crystals and in optoelectronic devices. Finally, we will demonstrate the remarkable stability of high-crystallinity perovskite single crystals, including their performance under extreme conditions such as gamma radiation, highlighting their potential for space applications.
2.1-I2
Perovskite solar cells (PSCs) have attracted intensive attention due to their ever-increasing power conversion efficiency (PCE), low-cost materials constituents, and simple solution fabrication process. In printable mesoscopic PSCs, the perovskite is deposited on a triple-layer scaffold, made of screen printed mesoporous TiO2 layer, ZrO2 spacer layer and carbon electrode; such devices use carbon electrodes to replace the noble metal back contacts and do not require a hole-conducting layer.
Controlling the crystallization of organic–inorganic hybrid perovskite is of vital importance to achieve high performing perovskite solar cells. The growth mechanism of perovskites has been intensively studied in devices with planar structures and traditional structures. However, for the printable mesoscopic perovskite solar cells, it is difficult to study the crystallization mechanism of perovskite owing to the complicated mesoporous structure. In this talk, I am going to share the development of the printable mesoscopic PSCs and how we manage to control the crystallization in mesopores.
2.1-O1
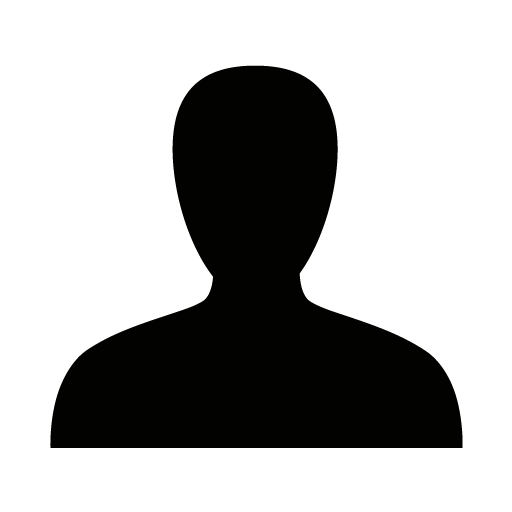
The commercialisation of perovskite photovoltaic (PV) technologies requires advancements in large-area module efficiency, scalable and cost-effective manufacturing, and long-term operational stability. Stability issues in perovskite solar cells (PSCs) often stem from the materials used in the hole-transporting layer (HTL). Innovative, sustainable, and affordable hole-transport materials (HTMs) are crucial to address these challenges. Cu₂ZnSnS₄ (CZTS), an earth-abundant p-type semiconductor traditionally used as a light-absorbing material in heterojunction solar cells, has recently gained attention as an HTL for PSCs due to its desirable electronic properties.
This study explores the synthesis and application of CZTS nanoparticles (NPs) as an HTM in PSCs. The nanoparticles were produced using a hot-injection method under an oxygen-free environment and subsequently processed into an ink formulation for spin-coating. The resulting CZTS thin films, approximately 50 nm thick, were annealed to ensure structural and optical transparency in the visible solar spectrum. Comprehensive material characterisation—including transmittance, Raman spectroscopy, UV-Vis spectroscopy, scanning electron microscopy, and X-ray diffraction—confirmed the quality and stability of the CZTS layers.
Preliminary findings indicate that PSCs employing CZTS as an HTL demonstrate superior stability compared to conventional organic HTM devices. Over one month, the CZTS-based devices retained or even improved their photovoltaic efficiency, unlike organic HTL-based devices, which experienced significant degradation. Current-voltage measurements, external quantum efficiency and photoluminescence spectroscopy analyses revealed enhanced charge injection in the CZTS HTL. These results suggest that CZTS is a robust and sustainable alternative to traditional HTMs, paving the way for more durable perovskite PV technologies.
2.2-I1
Perovskite-silicon tandem solar cells offer exceptional promise for high efficiency photovoltaics. However, integrating perovskite top cells uniformly onto textured silicon wafers remains challenging. Physical vapor deposition (PVD) methods, such as pulsed laser deposition (PLD)1, enable conformal and precise thickness control for perovskite deposition but are underexplored for this application.
Following our work on PLD of MAFAPbI3 for single junction devices2, we discuss two PLD-based approaches towards wide band gap halide perovskites deposited conformally onto texture silicon bottom cells. First, we discuss our recent developments on PLD of CsxFA1-xPb(BryI1-y)3 films for p-i-n single-junction and monolithic tandem devices. We discuss how the application of a PbI2-based template results in phase-pure, uniform CsxFA1-xPbI3 films with dense coverage on both planar and textured substrates. Cs0.2FA0.8PbI3 composition and a bandgap of 1.58 eV are confirmed. By controlling bromide ion incorporation in the PLD target, we achieve tunable bandgap energies (1.58–1.68 eV), aligning with silicon absorbers for current matching.
Additionally, we leverage the conformal properties of PLD, to fabricate inorganic scaffolds of PbI2:CsBr at deposition rates above 50 nm/min. The formation of the CsxFA1-xPb(BryI1-y)3 perovskite layer is finalised with a spin coating of organic cation solution containing FAI:FABr in ethanol. This also results in uniform films with dense coverage on both planar and textured substrates. Finally, both approaches are compared in terms of device performance, with preliminary results reaching above 13% PCE on 1 cm2 cells and textured silicon bottom substrates. This work highlights the importance of PVD techniques and their optimization for next-generation photovoltaics.
References
1. https://doi.org/10.1021/acsenergylett.4c01466
2. DOI: 10.1016/j.joule.2024.09.001
2.2-I2
Dr. Edgardo Saucedo studied Chemical Engineering at the University of the Republic, Montevideo, Uruguay, and received his PhD in Materials Physic at the Universidad Autónoma de Madrid, Madrid, Spain in 2007 with a FPU fellowship. In 2007, he joined the Institut de Recherche et Développement sur l’Énergie Photovoltaïque IRDEP (Paris, France), with a CNRS associated Researcher fellowship, working in the development and optoelectronic characterization of CIGS low cost based solar cells. In 2009, he joined NEXCIS, a spin-off created from IRDEP, to further pursue their training in photovoltaic technology. In 2010, he joined the Solar Energy Materials and SystemsGroup at the Catalonia Institute for Energy Research (IREC) under a Juan de la Cierva Fellowship first (2010-2011) and a Ramon y Cajal Fellowship afterwards (2012-2016), with the aim to develop new low cost materials and processes for thin film photovoltaic devices. In 2020 he joined the Polytechnic University of Catalonia (UPC) to continuous his scientific and professorhip career.
He holds five patents and has authored or co-authored more than 215 papers in recognized international journals, including: Energy and Environmental Science, Advanced Materials, Adv. Energy Materials, Journal of the American Chemical Society, Chemistry of Materials, Progress in Photovoltaics: Research and Applications, Solar Energy Materials and Solar Cells, NanoEnergy, J. Mater. Chem. A, J. Phys. Chem. C, etc. He has more than 350 contributions to the most important Congresses in Physics, Chemistry and Materials, and more than 35 invited talks around the world. He has been involved in more than 25 European and Spanish Projects (Scalenano, Inducis, Pvicokest, KestPV, Larcis, etc.), and he was the Coordinator of the ITN Marie Curie network Kestcell (www.kestcells.eu), the research and innovation project STARCELL (www.starcell.eu), and the RISE project INFINITE-CELL (www.infinite-cell.eu), three of the most important initiatives in Europe for the development of Kesterites. In 2019 he was granted with an ERC-Consolidator Grant by the European Research Council (SENSATE, 866018, 2020-2025), for the development of low dimensional materials for solar harvesting applications to be developed at UPC. Currently he is also the scientific coordinator of the European project SUSTOM-ART (952982), for the industrialization of kesterite for BIPV/PIPV applications.
He is frequently chairman and invited speakers in the most relevant Conferences in Photovoltaic (E-MRS, MRS, IEEE-PVSC, EUPVSEC, European Kesterite Workshop, etc.). He has supervised 11 PhD Thesis and is currently supervising 5 more. He has an h factor of 38 and more than 5000 citations. In 2020 he has been awarded with the ASEVA-Toyota Award for his contribution to the development of sustainable photovoltaic technologies using vacuum techniques (https://aseva.es/resolucion-de-los-primeros-premios-nacionales-de-ciencia-y-tecnologia-de-vacio-aseva-toyota/).
The development of photovoltaic (PV) technologies based on earth-abundant materials is a cornerstone for sustainable and cost-effective energy generation. Numerous solar-to-X applications, including single junction, indoor, tandem, and semi-transparent PV devices, as well as photoelectrocatalysis, require a broad spectrum of light absorption and band position. This wide variety of solar-driven niche markets necessitates the development of a PV technology with customizable band gaps for specific applications. Mature PV technologies such as crystalline Si, CdTe, and GaAs have fixed bandgap absorbers of 1.1 eV, 1.45 eV, and 1.3 eV, respectively, even though exhibit impressive power conversion efficiencies exceeding 22%. Some emerging PV absorbers like perovskite, organic, and Sb2(S,Se)3 also exhibit bandgap tuning properties from 1.2 eV to 1.95 eV, 1.49 eV to 2.0 eV, and 1.2 eV to 1.7 eV, respectively, enhancing the feasibility of tandem solar cell design and other PV applications. However, it is challenging for these materials groups to tune the bandgap below 1.0 eV, limiting the utilization of infrared photons. Moreover, these materials face environmental, cost, and stability concerns, limiting their potential for widespread deployment.
Kesterite semiconductors are gaining attention as they are earth-abundant, nontoxic, and have excellent stability properties and bandgap tuning ability. Recently, this technology has achieved 15% power conversion efficiency using a simple molecular ink process, demonstrating significant potential for widespread deployment. In this work, we will present the high adaptability of solution-based processes to achieve single-phase kesterite materials with high crystalline quality, resulting in devices with efficiencies beyond 10% under AM1.5G, with band gaps ranging from 0.9 to 1.7 eV. A systematic isovalent cationic (Ag, Cd, Ge) substitution for the selenide-based (Cu2ZnSn(S,Se)4) and sulfur-based kesterite (Cu2ZnSnS4) using advanced molecular ink solutions is the key strategy and will be detailed in the presentation. For the first time, we present a lower bandgap of 0.9 eV kesterite absorber with an efficiency exceeding 12%. A champion device with a 14.4% efficiency kesterite solar cell is achieved with an absorber bandgap of 1.15 eV. Furthermore, we showcase kesterite solar cells with decent efficiency under AM1.5G, with wide bandgaps up to 1.9 eV to 2.2 eV, ideal for indoor and underwater PV applications. Systematic photoluminescent, Time-Resolved Photoluminescence, phase structural, composition, and element distribution analysis, as well as optoelectronic analysis of the device, are performed in this work. This provides valuable insight into reducing recombination in the bulk of the different bandgap absorbers and at the heterojunction interface with different band alignment structures.
Furthermore, two important case studies of bandgap tuning will be presented. Firstly, the performance of the devices in indoor conditions will be measured using a tunable LED solar simulator, to systematically investigate the behavior of wide bandgap kesterite solar cells under a wide range of simulated indoor conditions (from 6000K to 2700K illumination). Over 18% efficiency kesterite solar cells under indoor conditions will be presented. Secondly, the series bandgap-tuned kesterite absorber are used as photocathode for investigating photoelectrochemical properties for water reduction.
In conclusion, this work will demonstrate that the low cost and high tolerance isovalent cationic substitution can lead to a very wide range of bandgap tunability in kesterite absorbers, with their customizable bandgaps enabling them to suit various specific application scenarios. This work provides critical insights into the widespread deployment of kesterite PV technology, offering a roadmap for future advancements.
2.2-I3
Prof. Marina Freitag is a Professor of Energy and a Royal Society University Research Fellow at Newcastle University. She is developing new light-driven technologies that incorporate coordination polymers to solve the most important challenges in the research area, including issues of sustainability, stability and performance of hybrid PV. The development of such highly innovative concepts has given Marina international recognition, including recipient of the prestigious 2022 Royal Society of Chemistry Harrison-Meldola Memorial Prize 2022.
Her research into hybrid molecular devices, began during her doctoral studies (2007-2011, Rutgers University, NJ, USA) where she was awarded an Electrochemical Society Travel Award and Dean Dissertation Fellowship 2011. Dr Freitag moved to Uppsala University (2013-2015) for a postdoctoral research position, which focused on the implementation of alternative redox mediators, leading to a breakthrough today known as “zombie solar cells”. Dr Freitag was invited to further develop this work at École Polytechnique Fédérale de Lausanne (EPFL) with Prof. Anders Hagfeldt ( 2015-2016). From 2016-2020 she was appointed as Assistant Professor at Uppsala University, Sweden, where she received the Göran Gustaffsson Young Researcher Award 2019.
Metal-organic liquids represent an emerging class of functional materials that combine the unique properties of coordination polymers with the versatility of liquid-state materials. In this work, we present the synthesis and comprehensive characterization of three novel copper-based MOLs and one silver-based MOL, all exhibiting melting temperatures below 100°C. These materials share the same [Cu] backbone structure but achieve different dimensionalities (1D, 2D, and 3D) through variation of the counter-cation, providing an unprecedented opportunity to correlate structural dimensionality with physical-chemical properties. Through detailed thermal analysis, we demonstrate glass transition temperatures ranging from -42°C to -21°C and melting points between 48°C and 88°C. Temperature-dependent Raman spectroscopy reveals the structural evolution during the solid-to-liquid transition, while computational modeling provides insights into the electronic band structures and charge transport mechanisms. The materials exhibit conductivities ranging from 10-10 to 10-6 S cm-1, with dimensionality playing a crucial role in determining transport properties. We demonstrate the practical application of these materials as hole transport materials in dye-sensitized solar cells (DSSCs) without requiring electrolyte additives. The materials' versatility is showcased through successful implementation in three different states - liquid, gel-like, and quasi-solid - offering flexible processing options for device fabrication. This work not only advances the fundamental understanding of metal organic liquids properties and phase transitions but also establishes their potential as multifunctional materials for energy applications.
2.2-O1
The widespread deployment of perovskite solar cells (PSCs) or other emergent PV technologies as a sustainable energy solution hinges critically on both their operational stability and the ability to efficiently extract maximum power under real-world illumination conditions. While significant advances have been made in PSC stability, particularly in triple-mesoscopic hole-transport-material-free architectures, conventional maximum power point tracking (MPPT) algorithms fall short when applied to these highly stable but hysteretic devices. This presentation introduces a novel galvanostatic power-tracking algorithm specifically designed to overcome the challenges posed by high-hysteresis PSCs, complemented by comprehensive real-world performance data.
Our approach utilizes cost-effective hardware[1,2] that enables parallel long-term stability measurements, addressing a critical gap in PSC characterization methodology. The system's architecture allows for continuous monitoring and precise power optimization, particularly crucial for devices incorporating power optimizers. We will present detailed performance metrics from extended outdoor testing, demonstrating the algorithm's superior tracking efficiency compared to traditional methods. The real-world data encompasses various environmental conditions, validating the system's robustness and reliability in actual operational scenarios. This work contributes to the broader goal of PSC commercialization by providing a practical solution for accurate power tracking, essential for both research and commercial applications.
Our findings have significant implications for the integration of PSC technology into various applications, including building-integrated photovoltaics (BIPV) and large-scale solar installations, where accurate power optimization is crucial for maximum energy yield. The combination of our innovative tracking methodology and real-world validation data provides valuable insights into the practical implementation of PSC technology, addressing key challenges in stability assessment and performance optimization for sustainable photovoltaic applications.
2.3-I1
Dr. Roberto Speranza is a postoctoral researcher at Politechnic of Turin where he obtained his Ph.d. in Electronic Engineering with a thesis entitled "Integrated Energy Harvesting and Storage Systems for a Sustainable Future". His main research interest are design, fabrication, and characterization of electrochemical technologies for energy harvesting and storage. Third-generation photovoltaic cells, with a focus on photoelectrochemical cells like dye-sensitized solar cells. Characterization and optimization of photovoltaic devices under non-standard illumination conditions (artificial lighting, indoor environments). Supercapacitors and their direct integration with photovoltaic technologies. Fabrication and characterization of photo-capacitors for direct energy harvesting and storage from natural and artificial light sources. Production and characterization of nanostructured materials for electrochemical devices. Polymer electrolytes.
The rapid development of low-power connected devices, widely used in fields such as the Internet of Things (IoT), building automation, smart agriculture, and wearables, has fueled research into novel technologies that can harvest energy from ambient sources and potentially store this energy, aiming to make these devices energy independent. A significant portion of the billions of IoT smart devices operates indoors, typically powered by batteries that require periodic recharging or disposal. This raises sustainability concerns related to maintenance and electronic waste production, highlighting the need for more efficient and self-sustaining solutions. As a result, there has been increased interest in developing indoor photovoltaics (IPV) for self-rechargeable IoT devices, addressing the limitations of traditional batteries and fostering the growth of large, energy-efficient IoT networks. [1] Moreover, the innovative concept of integrating photovoltaic cells for energy harvesting with an energy storage device, such as a supercapacitor (SC), to directly store this energy in a single unit is gaining recognition as a viable and sustainable alternative to batteries for powering low-power IoT devices. [2],[3] In this contribution, we address the sustainability of such integrated devices by selecting green, sustainable, and low-toxic materials that can be shared between the photovoltaic cell (i.e., a dye-sensitized solar cell, DSSC) and the supercapacitor, with a particular focus on their application in indoor environments. We demonstrate, for the first time, that γ-valerolactone (γ-VL), a sustainable, low-toxic solvent derived from cellulosic biomass, can be used for the preparation of the DSSC electrolyte as an alternative to more common but toxic and flammable solvents such as acetonitrile (ACN) and 3-methoxypropionitrile (MPN). Recently, γ-VL has also been shown to be well-suited for the preparation of high-voltage supercapacitors. [4] Our results indicate that γ-VL is unsuitable for outdoor DSSCs due to slower ion diffusion and reduced I3- reduction at the counter electrode. However, γ-VL-based DSSCs outperform those using ACN and MPN under indoor light (1000 lux), demonstrating equivalent short-circuit currents but with higher open-circuit voltages, improved fill factors, and enhanced overall efficiency, enabled by lower recombination at the photoanode. Thanks to the compatibility of γ-VL with both technologies, we fabricated an integrated energy harvesting and storage device using γ-VL for electrolyte preparation. Moreover, we also demonstrate that the same carbon-based materials used for the SC electrodes are also suitable as counter electrodes for the DSSC. The fabricated integrated harvesting and storage device showed optimal self-charging capabilities and stability under indoor illumination conditions, achieving high charging voltage and good charge retention over time, making it a promising alternative to disposable batteries for IoT devices.
2.3-I2
Organic solar cells and organic photodetectors have recently gained much interest due to their favorable properties like abundant materials, low-cost fabrication, machanical flexibility, and spectral tunability. In this talk, I will give an overview about our recent work on organic photodetectors. In particular, I will first address results on a key working principle, the exciton separation which is challenging in organic materials due to the high binding energy. We have recently shown that high-performance organic photodetectors can be realized without a needing a donor-acceptor heterojunction for separation: single-component devices based on the small molecule organic semiconductor DCV2-5T (an oligothiophene with dicyano-vinylene endgroups) show excellent properties: Due to low dark current and high external quantum efficiency, high specific detectivities of 10E13 Jones at zero bias are achieved. The single-component DCV absorber layer forms free charges rapidly and efficiently, without the need for a heterostructure with another material. The efficient charge generation in DCV2-5T is attributed to the strong electronic overlap of molecular excitons and intermolecular CT states. Furthermore, quantum chemical simulations predict a reduced electronic coupling for highly ordered (crystalline) DCV2-5T, which demonstrates that crystalline order is not a prerequisite for good performance. The exceptional performance of single-component OPDs demonstrates a successful strategy for simplified device fabrication and enhanced stability. In a second part, I will discuss high-performance narrow-band blue organic photodetectors through intended exciton quenching, realized by fine-tuning the optical and electrical properties of hole transport layers and introducing a MoO3 doped underlayer to the device. This filterless strategy ensures a high EQE of up to 50% at 0 V in thin-film devices. Doping can further improve EQE by assisting charge carrier dissociation. Ultralow dark currents can be obtained by planar heterojunctions, leading to a record-high detectivity D* of 6.35 × 10E14 Jones for blue OPDs, a performance exceeding that of most crystalline inorganic detectors in this wavelength range.
1.3-I1
Jonathan Owen received a B.S. in Chemistry from the University of Wisconsin-Madison, and a Ph.D. in Chemistry from CalTech. As a graduate student in the lab of Professor John Bercaw he studied the kinetics and mechanism of methane C-H activation. In 2005 he joined the lab of Professor Paul Alivisatos as a Petroleum Research Fund Alternative Energy Fellow to study the crystallization and derivatization of colloidal semiconductor nanocrystals. In 2009 he joined the faculty at Columbia University as an Assistant Professor of Chemistry where his group continues to study the synthesis and surface chemistry of colloidal semiconductor nanocrystals. For this work, he has received early career awards from the Department of Energy, the National Science Foundation, 3M, and DuPont.
I will describe the synthesis of colloidal GaP and InP nanocrystals in organic surfactant solution. Novel precursor conversion reactivity can be used to control the formation of solutes at a desired reaction temperature and to govern their concentration structure. That approach provides large (10-20 nm), highly crystalline GaP under mild conditions using convetional surfactant media. The high quality of the products is confirmed by their powder diffraction and Raman spectra which are narrower than previous reports under such conditions. The importance of the reaction temperature, solute composition, and the conversion reactivity to the final nanocrystal product will be discussed. The results will be used to highlight the central role of crystal growth in size and polydispersity control mechanisms. Moreover, the results highlight the essential role of precursor reactivity on the reproducibility of the synthesis and the value of carefully designing and tailoring reagents that afford control over that reactivity.
1.3-I2
Facet homogeneity in III-V nanocrystals is a critical factor for enhancing their optoelectronic properties and chemical stability. This talk explores recent advancements in synthesizing well-defined tetrahedral and tetrapodal geometries in indium-based III-V nanocrystals, emphasizing the facet-specific surface chemistry and its implications. By integrating halide-amine co-passivation strategies and advanced nuclear magnetic resonance analyses, we unveil the correlation between facet-dependent ligand dynamics and surface reactivity. These insights enable precise control over surface passivation, critical for reducing surface heterogeneity and enhancing photophysical properties. The discussion extends to the role of (111) facets in quantum confinement and their impact on device performance, including extended photoresponse in tetrahedral quantum dots. This comprehensive understanding of facet homogeneity not only addresses long-standing challenges in III-V nanocrystal synthesis but also opens new pathways for their application in next-generation optoelectronics.
Reference
[1] Semiconductor Nanocrystals: Unveiling the Chemistry behind Different Facets Acc. Chem. Res. 2023, 56, 1756
[2] Unraveling the Facet-dependent Surface Chemistry at Molecular Scale: Photo-assisted Oxidation of InP Nanocrystals J. Am. Chem. Soc. 2024, 146, 46, 31691
[3] Surface-Originated Weak Confinement in Tetrahedral Indium Arsenide Quantum Dots, J. Am. Chem. Soc. 2024, 146, 15, 10251
1.3-I3
Peter Reiss is researcher at the Interdisciplinary Research Institute of Grenoble (IRIG), France, and Head of the Laboratory Synthesis, Structure and Properties of Functional Materials (STEP). He graduated from University of Karlsruhe (Germany), and earned his PhD in Inorganic Chemistry under the supervision of Prof. Dieter Fenske (2000). His research activities focus on the synthesis and properties of colloidal semiconductor quantum dots and metal halide perovskites (nanoparticles and thin films). The studied applications range from biological imaging / detection over LEDs and displays to new strategies for energy conversion (photovoltaics, thermoelectrics, photocatalysis) and storage. Dr. Reiss acts as Associate Editor for Nanoscale Research Letters and Frontiers in Materials - Energy Materials, and is Editorial Board Member of Scientific Reports. He co-organizes the biennial conference NaNaX – Nanoscience with Nanocrystals (cf. http://nanax.org).
Indium phosphide quantum dots (QDs) have become the workhorse for visible light emission within the past decade and today, green- and red-emitting InP QDs are extensively used for color conversion in commercial displays. On the other hand, it turned out highly challenging to achieve efficient and narrow emission in the blue range around 450-470 nm, which remains an active field of research.
With a bulk band gap of 1.35 eV, InP QDs could be also of high interest for the near-infrared range, e.g., for in vivo biological imaging. However, for achieving an emission beyond 700 nm, large particle sizes > 7 nm are required, which turned out difficult to synthesize with established methods involving indium(III) halides or carboxylates and silyl- or aminophosphine precursors. While exploring the use of indium(I) halides for double use as the indium precursor and reducing agent of aminophosphine, we found that large tetrahedral InP QDs with edge lengths of around 10 nm could be obtained.[1] After overcoating with ZnS or ZnSe/ZnS shells, these QDs exhibit narrow NIR emission at wavelengths up to 730 nm. Additional coating with an alumina shell resulted in excellent chemical stability, demonstrated by transferring the QDs to the aqueous phase via surface ligand exchange while maintaining their photoluminescence quantum yield of around 40%.[2]
Going further in the near- and short-wave infrared range opens up a large space of additional applications for III-V QDs in various fields such as night-vision, plastic sorting, agriculture, surveillance and consumer electronics. Nonetheless, narrow bandgap III-V materials have been much less explored than lead chalcogenide QDs (PbS, PbSe) due to synthetic challenges related to their more covalent character, the scarcity of appropriate group-V precursors, and their high oxidation sensitivity. We extended the indium(I) halide / aminopnictogen synthetic platform to InAs and InSb QDs, which gave access to wavelengths up to 2 µm.[3] After overgrowth with appropriate shell materials, they can also act as efficient NIR/SWIR emitters.[4]
2.1-I1
Prof. Z. Hens received his PhD in applied physics from Ghent University in 2000, worked as a postdoctoral fellow at Utrecht University and was appointed professor at the Ghent University department of inorganic and physical chemistry in 2002. His research concerns the synthesis, processing and characterization of colloidal nanocrystals.
Quantum dots (QDs) made of III-V semiconductors have been investigated for many years as a more sustainable alternative for cadmium, lead or mercury based chalcogenides for applications involving visible, short-wave infrared or mid-wave infrared light. Led by progress in QD synthesis, these efforts have mainly focused on In-based materials, including InP, InAs and InSb. These In-based pnictides are all direct band gap semiconductors covering – when accounting for size quantization – a spectral range from the mid IR to the edge of the visible. Recently, synthetic methods for Ga-based pnictides have become available. GaP, however, is an indirect semiconductor, and GaAs and GaSb only cover a relatively narrow spectral range. Hence the question what to expect from Ga-based QDs for opto-electronic applications.
In this presentation, we introduce Bloch orbital expansion as a novel and unconventional computational approach to relate geometry and electronic structure in quantum dots. The method is based on the projection of the QD orbitals on bulk Bloch orbitals, and comparing the resulting QD fuzzy band structure with the bulk band structure computed at the same level of theory. Using this approach, strongly confined, delocalized QD orbitals will overlap with the bulk bands, while QD orbitals derived from bulk surface states will deviate from the bulk bands. Most notably, mid-gap surface states, which are most detrimental for the performance of opto-electronic devices, can be readily identified as falling within the bulk bands. Importantly, when using density functional theory (DFT) to compute QD orbitals for a given QD geometry, this approach provides a direct link between the QD surface termination and the appearance of such surface states.
In a first step, we apply the method to models of PbS, HgTe and HgSe QDs 3-4 nm in size. Interestingly, for all these QDs, we demonstrate that the orbitals are free from coupling to bulk surface states. This finding is rooted in the bulk band structure of these materials, and may explain why films of such QDs truly behave as printed semiconductors. Next, we use the coupling of QD orbitals to bulk surface states as an intrinsic quality-control method to screen the promise of different In- and Ga pnictides as an alternative to restricted Cd, Pb or Hg compounds. Using a fixed, 1116 atom QD model with chloride passivated (100) and (111) facets, we show that InP QDs exhibit a broad band of occupied surface states. These orbitals are related to the P-rich (-111) facets, and extend several 100 meV above the valence-band edge. Such a result can be expected for semiconductors with a p-type valence-band and an s-type conduction-band edge, and reflects charge accumulation at the (-111) facet. In line with this interpretation, we observe a gradual suppression of the coupling of QD orbitals with bulk surface states when reducing the difference in electronegativity between the anion and the cation. In particular, the frontier orbitals of GaAs and GaSb appear as delocalized states, which suggests that these compounds could be used as printed semiconductors with properties superior to In-based equivalents. We end by discussing the impact of these findings for research in III-V QDs.
2.1-O1
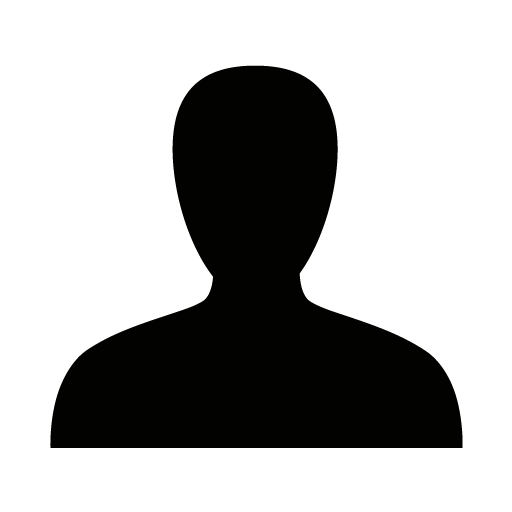
Colloidal quantum dots (QDs) are a versatile class of materials with notable potential in optoelectronics. Offering tunable optical and electronic properties and solution-processable fabrication, QDs have been widely explored in applications such as LEDs and photodetectors (PDs), where they can be used as printable inks. QDPDs are particularly interesting in the short wave infrared (SWIR) region (1 – 2µm), which is critical for many applications, such as environmental monitoring, biomedical and adverse weather imaging, and telecommunications. Current SWIR devices rely on costly fabrication techniques, but the integration of solution-processed QDs could substantially reduce the manufacturing costs of SWIR imagers, making them appealing for the consumer market.
Yet, efficient application of QDs to SWIR devices has been restrained to the use of lead sulfide (PbS), and mercury telluride (HgTe), which face significant regulatory restrictions due to their hazardous nature. In recently years, QDPDs based on III-V compounds, particularly In(As,P), have gained attention as a cost-effective, solution-based, and regulatory-compliant alternative for SWIR PDs, driving improvements on their synthetic chemistry, surface passivation and optimization of device structures. However, thus far, the performance metrics, such as quantum efficiency and dark current, of In(As,P) QDPDs have remained subpar relative to PbS-based devices, and have not significantly benefited from ligand engineering, suggesting other factors affect device performance.
Here, we investigate the relation between the properties of the In(As,P) QDs and the PD performance. QDPDs are fabricated using an established ligand exchange chemistry, involving the replacement of oleylamine and chloride by mercaptopropanediol and butylamine [1]. Current-voltage (I-V) measurements show that the resulting QDPD stacks are rectifying, and attain an external quantum efficiency (EQE) of up to 10-20% at 1050 nm. Impedance measurements are used to obtain deeper insight in the semiconductor characteristics of the In(As,P) QD film and the voltage distribution across the stack. A striking observation is the high dark current under reverse bias, which increases with adaptations to the stack that enhance the EQE. By combining temperature-dependent I-V measurements and transient absorption spectroscopy, we propose thermal generation of charge carriers within the QD film as the main source of dark current, and we discuss the prospects of adapting the QD surface termination so as to reduce dark current and enhance detectivity of PDs based on III-V QDs.
2.1-O2
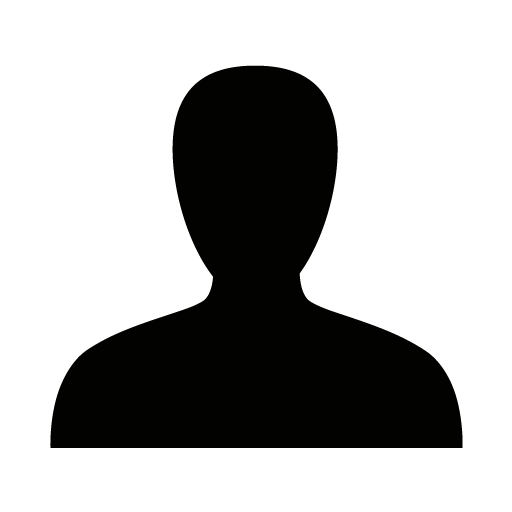
Quantum dots (QDs) are nanometer-scale semiconductors with tunable bandgaps, making them ideal for optoelectronic devices like LEDs, photodetectors, lasers, and bioimaging tools. Their strong light absorption, tunable properties, and suitability for solution-based processing have driven interest in materials like lead halide perovskites, and cadmium, lead, and mercury-based chalcogenide QDs. However, their use is limited by toxic heavy metals, restricted by RoHS regulations. This has spurred demand for environmentally friendly alternatives, such as III-V QDs—particularly indium phosphide (InP) and indium antimonide (InSb)—which offer a wide bandgap range, high electron mobility, and strong covalent bonds. Despite their potential, the development of III-V QDs has faced challenges, including issues with precursor availability, high nucleation temperatures, and polydispersity.[1]
In our research, we have made significant advancements in the colloidal synthesis and surface chemistry of InP and InSb QDs.[2] Using a heating-up method, we synthesized high-quality, monodisperse In-based QDs with size-tunable absorption features spanning from the visible to short-wave infrared range (445–1980 nm). To address the challenge of size tunability in InSb QDs, we developed various approaches to control their dimensions. For instance, different metal halides (InX3, SbX3, where X = Cl, Br, I) were employed as In and Sb precursors, with metal iodides producing the smallest InSb QDs among all tested halides. Additionally, the In-to-Sb ratio and the concentration of the reducing agent (super hydride) significantly influenced the QD size. A higher In/Sb ratio yielded larger QDs, while a higher concentration of super hydride resulted in smaller QDs, and vice versa. The resulting InSb QDs exhibited excellent colloidal and optical stability in non-polar solvents after four months. To enable their integration into highly conductive optoelectronic devices, we successfully exchanged the organic ligands of these QDs with various inorganic ligands, including metal halides, metal chalcogenides, and metal chalcogenide complexes. We elucidated the mechanisms behind the ligand exchange processes, facilitating the creation of QD inks capped with inorganic ligands. These inks were subsequently used to fabricate field-effect transistors, which exhibited enhanced conductivity. Our work marks a significant step in developing high-performance III-V-based optoelectronic devices, particularly in the infrared spectrum.
2.1-I2
Lutfan Sinatra holds the position of VP of Product Development at Quantum Solutions. He earned his PhD in Material Science and Engineering from King Abdullah University of Science and Technology (KAUST, Saudi Arabia). Additionally, he received his MS in Photonics from the Abbe School of Photonics at Friedrich-Schiller University Jena, where he conducted research on the interactions between light and nanoparticles. Over the past decade, his primary focus has been on nanomaterial synthesis and its diverse applications. Lutfan possesses extensive experience in designing and developing quantum dot materials for optoelectronic applications.
Colloidal quantum dots (QDs) offer a cost-effective and scalable solution for manufacturing thin-film semiconductors with tunable band-gaps. Among them, certain infrared-active QDs have demonstrated exceptional potential for sensing applications beyond the capabilities of conventional silicon-based sensors. Notably, QDs such as PbS, PbSe, HgTe, Ag₂Te, InAs, and InSb have been extensively studied, with some, like PbS QDs, already reaching commercialization for short-wave infrared (SWIR) applications. However, the development of these materials varies, with ongoing research focused on enhancing their performance and industrial viability. As one of the pioneers in the commercialization of QDs for SWIR sensors, we would like to provide an overview of the progress in colloidal QD sensor technology for SWIR applications. We will begin by examining the achievements of PbS QDs, addressing their limitations in industrial applications. We will then explore the current advancements in III-V QDs, with a particular focus on InAs QDs and their potential to meet industry demands and drive the next generation of SWIR sensing technologies.
2.2-I1
Alex earned his Ph.D. in physics of semiconductors from Chernivtsi National University, Ukraine for his work on electronic properties of nitride semiconductor alloys.
In 2004 he joined the Quantum Semiconductors and Bionanophotonics lab at University of Sherbrooke as a postdoc, working on theoretical modeling of laser-assisted quantum well intermixing and self-assembly processes of organic monolayers on metal and semiconductor surfaces for applications in bio-sensing.
In 2008 he moved to Quantum Theory Group at National Research Council of Canada in Ottawa, where he worked on many-body problems in epitaxial and colloidal semiconductor and graphene quantum dots; in particular, simulations of multi-exciton generation, Auger processes and optical properties of nanocrystals used in hybrid polymer-semiconductor solar cells.
Alex joined Ted Sargent’s Nanomaterials for Energy Group in 2011 and worked on characterization and modeling of the semiconductor nanocrystal surfaces and developing the synthesis methods for nanomaterials with improved optical and transport properties for photovoltaics.
In 2018, Alex joined the Department of Physical and Environmental Sciences at the University of Toronto, Scarborough as an Assistant Professor in Clean Energy. His topics of interest are materials for energy storage and novel materials discovery using high-throughput experiments and machine learning.
InSb has a larger Bohr radius and thus offers a wider range of infrared wavelengths compared to other III-V's. Provided the complexity of achieving good shape and size control for large QDs, InSb can stay within a smaller size regime to achieve the 1400-1500 nm wavelengths of interest.
Here the synthetic challenges associated with InSb CQDs are investigated and it is found that uncontrolled reduction of the antimony precursor hampers the controlled growth of CQDs. To overcome this, a synthetic strategy that combines nonpyrophoric precursors with zinc halide additives is developed. The experimental and computational studies show that zinc halide additives decelerate the reduction of the antimony precursor, facilitating the growth of more uniformly sized CQDs. The halide choice provides additional control over the strength of this effect.
I will also discuss our computational efforts in understanding the surface structure of InSb dots and ligand exchanges to reduce surface oxidation.
Our further efforts are focused on understanding the nucleation process that can lead to better monodispersity. Specifically, I will discuss the kinetic models that include both anions and cations as well as real-space models that consider the geometric shape effects on nucleation and growth.
2.2-O1
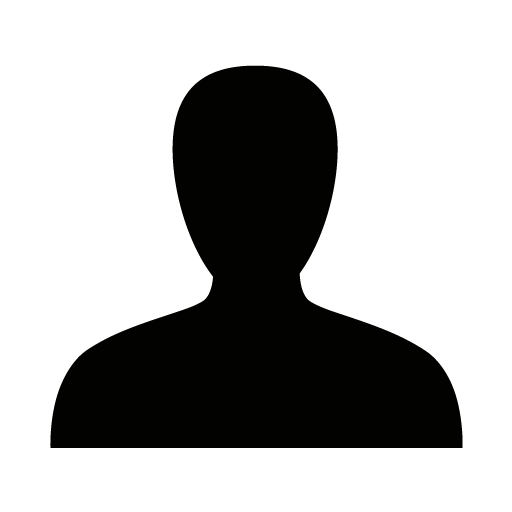
Metal oxides, like ZrO2 and HfO2, and fluorides (e.g. NaYF4 and NaGdF4), are two classes of nanocrystals serving as hosts for optically active lanthanides ions (e.g. europium).1-5 While doped fluorides are widely studied and their syntheses are well developed, the oxide hosts struggle with the synthetic challenge of producing colloidally stable nanocrystals with a complex architecture (as for example core/shells). In this work, we pioneer the synthesis of metal oxide core/shell nanocrystals where HfO2 epitaxially grows onto ZrO2, confirmed by high resolution transmission electron microscopy images and energy-dispersive Xray spectroscopy compositional maps. Furthermore, the beneficial effect of the shell on the optical properties is established by investigating the photoluminescence of ZrO2:Eu and of ZrO2:Eu/ZrO2 after growing a protective zirconia shell on it. After shelling, the lifetime of the europium doped zirconia nanocrystals increases to 5.3 ± 0.1 ms, showing that we successfully shut down non-radiative pathways. The long lifetime is unprecedented for the high doping percentage (9% Eu). The method has been demonstrated to improve the local environment of europium dopants in zirconia nanocrystals, giving access to novel heterostructures with improved optical properties. This opens up possibilities for their application in different areas, from microelectronics to scintillators.
2.2-I2
Achieving high photoluminescence quantum yield (PLQY) in magic-sized clusters (MSCs) of III-V semiconductors such as indium phosphide (InP) remains a significant challenge due to their strong oxophilicity and high sensitivity to surface defects. Here, we report the synthesis of highly luminescent InP MSCs through a novel kinetically controlled surface fluorination strategy. Utilizing the Friedel–Crafts acylation reaction, we generated hydrogen fluoride (HF) in a controlled manner, enabling effective surface passivation. This approach mitigated non-radiative recombination pathways by removing surface oxides and stabilizing surface defects, resulting in a PLQY of ~18%, the highest reported for InP MSCs. Comprehensive analyses, including PL lifetime measurement, transient absorption spectroscopy, and X-ray photoelectron spectroscopy, revealed that the enhanced luminescence arises from reduced surface trap states. Structural integrity and uniformity were confirmed through X-ray diffraction, Raman spectroscopy, and extended X-ray absorption fine structure analysis, demonstrating the preservation of the zinc-blende MSC framework. These findings not only advance the understanding of III-V MSCs but also highlight the potential of InP MSCs as environmentally benign, monodisperse, and highly efficient emissive materials for next-generation optoelectronic applications.
2.2-I3
Advances in the synthesis of III-V quantum dots, including InP and InAs, have led to their development for current- and next-generation solid-state lighting, wide color gamut displays, and infrared optoelectronics. The most widely adopted synthesis of these III-V quantum dots involves indium carboxylates and E(SiMe3)3 (E = P, As) and is understood to proceed through the formation of metastable, atomically-precise intermediates that are often referred to as clusters. In this work, we investigate the synthesis and growth pathways of III-V clusters to draw conclusions about kinetic differences in their formation. Along with synthetic experimentation, we analyze the single-crystal X-ray diffraction structures of some of the reported intermediates including In37P20(O2CR)51, In26P13(O2CR)39, and In26As18(O2CR)24(PR’3)3.[1],[2] The structural similarities between these materials and other II-VI materials of similar morphology have strong implications for understanding the landscape of accessible binary semiconductor clusters. Modifying these structures through the introduction of dopants has allowed for the formation of desirable, complex compositions including manganese, cobalt, and molybdenum. We find that the addition of L-type amine modifies the surface of these materials and leads to an amorphous single-source precursor primed for the formation of doped-InP nanomaterials. Expanding the library of cations in conjunction with exploring the basicity of the dopant-assisting L-type ligand could lead to alloys that are difficult to achieve with more conventional colloidal techniques.
2.3-O1
InP-based quantum dots (QDs) represent the major commercial success of colloidal semiconductor nanocrystals (NCs). A combination of the robust, mostly covalent, crystal structure and the non-toxic nature of the constituent elements makes them a QD material of choice for cutting-edge display and LED technologies.[1,2] Despite successful commercial realization, InP NCs lack convenient synthesis chemistry, as illustrated by a resent quest to substitute commonly used pyrophoric and expensive tris(trimethylsilyl)phosphine precursor.[3-5] Herein, we propose solid, non-pyrophoric, and synthetically easily accessible acylphosphines as convenient phosphorus precursors for the synthesis of InP QDs. When combined with suitable anionic nucleophiles, such as arylthiolates, both triacylphosphines and indium complexes of bisacylphosphines act as efficient sources of P3- anion, as corroborated by the results of NMR spectroscopy and powder XRD studies. This type of reactivity is utilized to synthesize uniform colloidal InP QDs with well-defined and tunable (460 – 600 nm) excitonic features in their absorption spectra. The final NCs size is controlled by the nature of acyl substituents and by the use of either indium or zinc long-chain carboxylates as ligands. Such adjustable precursor reactivity offers an improved control over the colloidal synthesis of InP, potentially opening a pathway to diverse InP-based hetero-nanostructures and InP NCs of anisotropic shapes. Furthermore, the proposed chemistry should be readily extendable to the synthesis of other metal phosphide and metal arsenide NCs.
2.3-I1
Colloidal InAs quantum dots (QDs) are gaining increasing interest as optimal infrared (IR) absorbers and emitters for the next-generation optoelectronic IR commercial devices.[1] This is due to their RoHS compliance and the tunability of their optical bandgap, which can be adjusted from approximately 700 nm to over 1600 nm. To date, the most advanced synthesis strategy for InAs QDs relies on pyrophoric, toxic and costly tris-trimethylsilyl arsine (or derivatives).[2] To reduce the cost and hazardousness of InAs QD production, several less toxic and more affordable arsenic precursors have been investigated in recent years, with tris(dimethylamino)arsine (amino-As) emerging as the most promising one.[3]
The current challenge is twofold: first, to enhance control over amino-As InAs quantum dots (QDs) to fine-tune their size and size distribution, thereby producing QDs with a tunable and narrow excitonic absorption peak; second, to develop tailored InAs@shell core@shell heterostructures with customized shell materials, achieving high efficiency as infrared (IR) emitters.
In this talk, I will describe our recent efforts to address these challenges. This involved further advancing our recently published procedure for synthesizing InAs@ZnSe QDs, utilizing amino-As and ZnCl₂ as an additive.[4,5]
2.3-I2
Metal halide perovskites (MHPs) possess a remarkable combination of properties that make them highly attractive for a wide range of optoelectronic applications. Their outstanding optical indicators such as high absorption coefficient, tunable bandgap through compositional adjustments, and strong photoluminescence are complemented by the low-cost, solution-based fabrication methods that enable scalable and economically viable production. One of the major challenges with MHPs is their inherent sensitivity to moisture, heat, and UV light, which raises concerns about their long-term stability in practical applications [1], [2]. Understanding the underlying mechanisms of degradation is therefore crucial.
In this context, a combination of in situ and ex situ electron microscopy techniques provide invaluable input. We conducted in situ experiments using both transmission electron microscopy (TEM) and scanning electron microscopy (SEM) at different scales, and compared our findings with ex situ results to corroborate the degradation pathways. In this manner, we could precisely characterize the black-to-yellow phase transition in both CsPbI₃ films and nanocrystals (NCs). Yellow phase CsPbI3 is formed as hollow microtubes elongated along the {100} plane after NC agglomeration and cannot be transformed back into the black phase upon heating at 350 0C, unlike films. Notably, our results indicate that oxygen does not play a significant role in the degradation process. Instead, one-time exposure to H₂O vapor is sufficient to initiate the detrimental phase transformation. For films, this transformation is further complicated by the formation of side phases, particularly inside pinholes. The combination of H₂O vapor and heat leads to the formation of PbO regions and decomposition of the CsPbI3 NCs into PbI₂ (P63mc) and CsI.
Obtaining high-resolution details of these crystal phase changes is extremely challenging due to the electron beam sensitivity of MHPs, often leading to Pb/PbX2-clusters formation or amorphization. Significant efforts have therefore been directed towards developing low-dose imaging protocols, e.g. based on “4D STEM” using event-driven direct electron detectors. Using doses of less than 500 e/Ų, we could characterize the local structure defects of MHPs. Moreover, this approach enables to visualize light elements within the perovskite lattice. As such, we observed for the first time the coexistence of CsPbI₃ and CsPbCl₃ domains, as well as the presence of mixed CsPb(I,Cl)₃ phases in perovskite/chalcohalide heterostructures.
A quantitative interpretation of TEM data is especially critical in the characterization of structural defects. For instance, stacking faults in MHPs frequently result in the formation of Ruddlesden-Popper (RP) phases. By applying statistical parameter estimation theory [3] together with molecular dynamics simulations, we have been able to quantify total column intensities and the probabilities that atomic columns belong to either the RP defect phase or the perovskite phase. This detailed analysis was vital in the study of CsPbI₃ nanocrystals (NCs), where RP-like phases were induced by dopants. The presence of these RP-like phases was correlated with phase stability measurements, offering valuable insights into the relationship between defect formation and the long-term structural stability of the material.
Finally, the influence of nanoparticle shape on the optical properties cannot be overlooked. However, 3D characterization methods such as electron tomography are electron-dose expensive. We have therefore quantified the number of atoms from a single projection and consequently modeled the shapes of perovskite NCs. Such advancements are crucial for understanding and optimizing the performance of perovskite-based devices, where both structural and morphological parameters influence their function.
Cruz-K1
Maksym Kovalenko has been a tenure-track Assistant Professor of Inorganic Chemistry at ETH Zurich since July 2011 and Associate professor from January 2017. His group is also partially hosted by EMPA (Swiss Federal Laboratories for Materials Science and Technology) to support his highly interdisciplinary research program. He completed graduate studies at Johannes Kepler University Linz (Austria, 2004-2007, with Prof. Wolfgang Heiss), followed by postdoctoral training at the University of Chicago (USA, 2008-2011, with Prof. Dmitri Talapin). His present scientific focus is on the development of new synthesis methods for inorganic nanomaterials, their surface chemistry engineering, and assembly into macroscopically large solids. His ultimate, practical goal is to provide novel inorganic materials for optoelectronics, rechargeable Li-ion batteries, post-Li-battery materials, and catalysis. He is the recipient of an ERC Consolidator Grant 2018, ERC Starting Grant 2012, Ruzicka Preis 2013 and Werner Prize 2016. He is also a Highly Cited Researcher 2018 (by Clarivate Analytics).
This year marks the first decade of colloidally synthesized lead halide perovskite quantum dots (LHP QDs), defining QDs as size- and shape-uniform ensembles with tunable quantum confinement and single-photon emission. Gradually, during this period, practically the entire compositional within a general formula APbX3 was thoroughly studied, with A being cesium (Cs), methylammonium (MA), formamidinium (FA), and azeridinium (AZ) was produced as high-quality nanocrystals. This journey is, arguably, at its very beginning. The LHP QDs are vastly different from conventional, more covalent semiconductors – they are ionic compounds with much lower formation energies, entropically stabilized, and structurally dynamic. The design of surface capping ligands turned out to be decisive for their stabilization at the nanoscale and for taming their photophysics. Currently, LHP NCs are prototyped as primary green emitters for television displays owing to facile and scalable production, higher emissivity-per-mass under blue excitation, and narrow emission linewidth. Their excitonic characteristics exceed initial expectations in many regards, opening opportunities as quantum light sources. In particular, at cryogenic temperatures, LHP QDs exhibit long excitonic coherence times, which start to match the fast sub-100 ps radiative rates. Both characteristics are optimized, to our surprise, in larger CsPbX3 QDs beyond the quantum confinement, namely, 20-40 nm, owing to the single-photon superradiance effect (giant oscillator strength at the single-exciton per NC regime). Single-component and multicomponent QD superlattices exhibit collective emission, known as superfluorescence, characterized by the oscillating, ultrafast (10-30 ps) radiative decays. This presentation will walk you through both the most essential progress over this first decade, including our current work, and outline future prospects.
Cruz-K2
Liquid phase exfoliation has been proved to be a cheap, scalable method for the mass production of 2D sheets. This talk will first discuss the galaxy of existent layered materials, with emphasis on synthesis, liquid-phase exfoliation, and characterization, focussing on some key applications recently developed in our laboratories, ranging from energy storage to printed electronics.
We will for example discuss how two-dimensional nanomaterials can be formulated in aqueous and organic viscous inks for conventional slurry castin, as well as extrusion printing, inkjet printing, and aerosoljet 3D printing, and demonstrate direct printing on various substrates
1.2-I2
Laura Herz is a Professor of Physics at the University of Oxford. She received her PhD in Physics from the University of Cambridge in 2002 and was a Research Fellow at St John's College Cambridge from 2001 - 2003 after which she moved to Oxford. Her research interests lie in the area of organic and organic/inorganic hybrid semiconductors including aspects such as self-assembly, nano-scale effects, energy-transfer and light-harvesting for solar energy conversion.
Organic-inorganic metal halide perovskites have emerged as attractive materials for solar cells with power-conversion efficiencies of single-junction devices now exceeding 26%. However, defective interfaces with charge extraction layers, the low hurdle for ionic migration, and the structural flexibility of the perovskite structure still pose both opportunities and challenges to their commercialization in light-harvesting applications. Combinatorial characterization approaches are vital for probing and analysing such instabilities.
We demonstrate a combined modelling and experimental approach[1] towards exploring the effects of energy-level alignment at the interface between wide-bandgap mixed-halide perovskites and charge-extraction layers, which still causes significant losses in solar-cell performance, focusing on FA0.83Cs0.17Pb(I1-xBrx)3 with bromide content x ranging from 0 to 1, and poly[bis(4-phenyl)(2,4,6-trimethylphenyl)amine| (PTAA). Through a combination of time-resolved photoluminescence spectroscopy and numerical modeling of charge-carrier dynamics[1] we reveal that open-circuit voltage losses associated with a rising energy-level misalignment derive from increasing accumulation of holes in the HOMO of PTAA, which then subsequently recombine non-radiatively across the interface via interfacial defects. These findings highlight the urgent need for tailored charge-extraction materials exhibiting improved energy-level alignment with wide-bandgap mixed-halide perovskites.
We further demonstrate optical-pump THz-probe spectroscopy with controlled intervals of air exposure as an ideal technique to monitor air-induced degradation of optoelectronic parameters such as charge-carrier mobilities and recombination rates in low-bandgap lead-tin iodide perovskites.[2][3] We explore the best choice of A-cation in lead-tin iodide perovskites with intermediate lead-tin ratios and find that air exposure induces hole doping to a similar extent, for methylammonium (MA) formamidinium (FA), FA cesium (Cs) and FA-only cations. However, we find that MAFA-based perovskites are unstable under heat exposure owing to decomposition of MA, and FACs perovskites suffer from A-cation segregation and an accompanying non-perovskite phase formation.[3] Thus we propose that from a stability perspective, efforts should refocus on FASn0.5Pb0.5I3 which minimizes all three effects while maintaining a suitable bandgap for a bottom cell and good performance.
We further utilize a combination of ultra-low frequency Raman and infrared terahertz time-domain spectroscopies to provide a systematic examination[4] of the ultra-low frequency vibrational response for a wide range of metal-halide semiconductors: FAPbI3, MAPbIxBr3–x, CsPbBr3, PbI2, Cs2AgBiBr6, Cu2AgBiI6, and AgI. We examine the cause of a frequently reported “central Raman peak” and rule out extrinsic defects, octahedral tilting, cation lone pairs, and “liquid-like” Boson peaks as causes.[4][5] Instead, we propose that the central Raman response results from an interplay of the significant broadening of Raman-active, low-energy phonon modes that are strongly amplified by a population component from Bose–Einstein statistics toward low frequency.[4] These findings elucidate the complexities of light interactions with low-energy lattice vibrations in soft metal-halide semiconductors emerging for photovoltaic applications.
1.2-O3
The charge carrier lifetime is a widely used metric for characterizing recombination in semiconductors. However, in the case of lead halide perovskites, there is considerable ambiguity surrounding reported lifetime values and their interpretation. This raises two fundamental questions: how should the lifetime be measured, and more importantly, what does it truly represent?
In theory and experiment, a multitude of different “lifetimes” can be defined, most of which are functions of the carrier density instead of constant. In doped semiconductors, mechanisms like detrapping and photodoping due to trapping can often be ignored, allowing different lifetime definitions to converge, so that no distinction is necessary. Additionally, their values are simplified to constants in many situations, streamlining the interpretation. None of these simplifications can be done for semiconductors with low intrinsic doping concentrations such as lead halide perovskites.
This presentation will explore the differences between the various definitions of time scales in theory and photoluminescence experiments and their connection to each other in intrinsic semiconductors. We provide experimental evidence indicating that high densities of shallow traps dominate transient photoluminescence decays in lead halide perovskites. Furthermore, we will clarify how these observations contrast with lifetimes derived from steady-state measurements, offering insights into the complexity of recombination dynamics in these materials.
1.2-I1
Two-dimensional hybrid perovskites are a highly intriguing class of materials, composed of alternating inorganic and organic molecular layers. Their reduced dimensionality combined with weak dielectric screening leads to the formation of tightly bound excitons that efficiently absorb and emit radiation. A central questions for excitons in perovskites from the perspectives of both fundamental physics and applications is their mobility. In addition, the flexibility of the material design allows for the integration of a variety of functional compounds including chiral molecules to enable polarization control of the optical response. In this talk I will focus on the transport of optically detected excitons in 2D perovskites via transient, ultrafast microscopy, featuring different regimes of propagation featuring free and localized states. I will show how the exciton transport is directly monitored using optical means, how it depends on conditions favoring either free particle diffusion or hopping, as well as how non-equilibrium populations lead to intriguing time-dependent dynamics. Finally, exciton propagation in chiral 2D perovskites will be demonstrated and its key relationship to the disorder discussed.
1.2-O1
Quantum dots (QDs) offer unique physical properties and novel application possibilities like single-photon emitters for quantum technologies. While strongly-confined II-VI and III-V QDs have been studied extensively, their complex valence band structure often limits clear observations of individual transitions. In recently emerged lead-halide perovskites, band-degeneracies are absent around the bandgap reducing the complexity of optical spectra. These QDs exhibit several distinct absorption resonances,[1] which can be assigned to excitons confined with respect to their center-of-mass motion within the weak confinement regime. [2] The well-defined excitonic energy landscape with significant confinement energies of these QDs suggests a high potential for light amplification. However, there is still debate on the nature of gain in perovskite QDs, which has been attributed to different origins such as biexcitons, trions, and single excitons. Here we study amplified spontaneous emission and optical gain of monodisperse spherical CsPbBr3 QDs and conclusively assign the gain to biexcitons.[3] This is based on the gain threshold and its spectral position which we study via femtosecond transient absorption spectroscopy. Furthermore, the optical gain vanishes within 30 ps, matching the biexciton lifetime, demonstrating the strong correlation to the biexciton population. By identifying the intrinsic mechanism of optical gain in CsPbBr3 QDs and its limiting factors, our findings show the direction for future work on optimizing their gain threshold and lifetime.
1.2-O2
In order to implement quantum dots (QDs) into most optoelectronic applications, from photovoltaic to light emitting devices, one needs to organize them into an interconnected array in the shape of a QD film. In this configuration a certain degree of electronic coupling among QDs is achieved, allowing for charge injection/extraction, at the expense of a reduction of the quantum confinement that characterizes these structures.
In this presentation we will discuss recent results where carrier cooling and recombination have been studied in QD solids as a function of connectivity. In order to exert control on electronic coupling among QDs we vary their average separation through their concentration when grown within the pores of nanoporous metal-oxide matrices. [1] We perform a thorough photophysical characterization comprising linear spectroscopy, PL quantum yield and ultrafast spectroscopic measurements while tuning the average QD separation and observe a transition in carrier recombination from isolated nanostructures to interconnected ones. The effects on global emission properties in terms of isolated and bulk-like behavior are pointed out in detail. [2]
Similar studies in the initial instants after carrier photo-excitation allow us to unveil the role of electronic coupling in the intra-band cooling of excited carriers. A retardation of the cooling dynamics is observed as we reduce QD connectivity and associated with carrier reheating taking place as a consequence of Auger-like processes. [3]
Thus a broad picture is provided on the fate of photoexcited carriers in QD films as a function of connectivity that can help to build an intuition when planning the use of these structures in devices operating in different carrier density regimes.
1.3-O1
There is recent evidence that, upon polaron formation, excitonic interactions in bulk lead halide perovskites experience different dielectric screening at short and long distances.[1,2] Above the polaron radius, static dielectric screening prevails. Under the polaron radius, dynamic screening prevails instead.
Here we investigate how such non-hydrogenic interactions affect the electronic structure of excitons, trions and biexcitons in confined halide perovskites: nanoplatelets and nanocrystals. To do so we develop a theoretical model which accounts for the main physical factors in these systems: (i) quantum confinement, through k·p theory, (ii) dielectric confinement, through image charge methods, (iii) electronic correlations, through a variational Quantum Monte Carlo method, and (iv) polaronic interactions, through Haken-like potentials.
We show that trions are formed by an exciton plus an excess carrier orbiting at longer distances, which reveals a significant ionic character of the bond. Biexcitons are formed by two excitons with strong intra-exciton interactions, and weaker inter-exciton ones. Polaronic interactions lead to large binding energies for excitons, trions and biexcitons. These are in good agreement with experimental measurements of nanoplatelets and Ruddlesden-Popper perovskites.[3-5] For nanocrystals, however, biexciton binding energies are systematically smaller than experimental values. This suggests excitons and biexcitons polarize the lattice differently.
1.3-O2
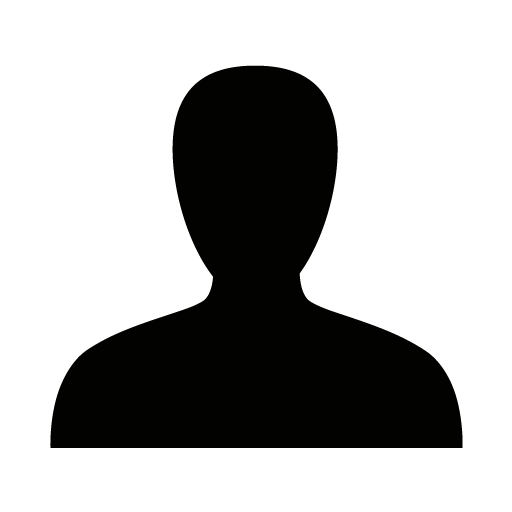
Perovskite nanocrystals exhibit exceptional optical properties, including ultrawide color tunability, high quantum efficiency and coherent single-photon emission. [1]
This renders them optimal candidates for optoelectronic devices, yet a comprehensive understanding of their optical characteristics is crucial.
Spectroscopy on single perovskite crystals helps to reveal their excitonic fine structure, which in thin films is concealed by inter-particle phenomena.
While photoluminescence (PL) studies have been conducted on single perovskite nanocubes, their wavelength-dependent absorption cross section has not been reported so far.
Since PL emission always originates from the energetically lowest state of the material, obtaining absorption data can contribute to a more complete picture of the energetic landscape.
The lack of these absorption measurements arises from the inherent difficulty in measuring the marginal absorption of such nanoscale samples.
To overcome this challenge we use an optical resonator, specifically a high-finesse microcavity [2], in which the light passes through the sample a thousand of times, thereby enhancing its absorption to a measurable amount.
This enables us to conduct ultra-sensitive and spectrally resolved measurements of the absorption cross section of perovskite nanocubes at room temperature.
The combination of this novel technique with PL and scanning electron microscopy (SEM) provides unique insight into the size- and morphology dependence of the energetic structure of perovskite nanocubes. Moreover, the degradation of the nanocrystals can be monitored by time-dependent changes in the spectral absorption signal.
An imminent replication of our measurements at cryogenic temperatures is expected to provide further understanding of the material’s optical properties.
The samples are provided by the groups of Prof. Urban and Prof. Kovalenko.
The findings subsume in a series of hyperspectral absorption measurements that were previously conducted on carbon nanotubes and defects in a two-dimensional material [3].
The progress towards routine measurements of samples on the nanoscale demonstrate that cavity-enhanced absorption spectroscopy has the potential to become a standard tool for the characterization of perovskite nanocrystals and other nanoscale samples, similar to the measurement of PL.
1.3-I1
Lead-based mixed-halide perovskites have a number of uses in solar and in light emitting applications. Unfortuantely, they exhibit ionic instabilities under illumination. Chief among them is photosegregation, a phenomenon first reported by McGehee in 2015. Since then, photosegregation has beceome well established as the light-driven segregation of iodine and bromine anions in mixed-halide lattices into iodine-rich and bromine-rich inclusions of the alloy. The phenomenon is reversible under darkness and segregated anions can remix.
Multiple models have been developed to explain photosegregation. Among them are thermodynamic, polaron, chemical, and trap-related models. While models explain photosegregation to varying extents only a band gap thermodynamic model has explained in a quantitative manner, observations of excitation intensity (Iexc)-dependent terminal halide stoichiometries and photoegregation Iexc thresholds.
One observation that has not been explained by any model though is the recent observation by Bach and co-workers of light-driven anion remixing in methylammonium lead iodide/bromide microcrystals. Hindering a deeper understanding of this photoresponse has been the fact that the Bach report is the only report of persistent photoremixing in a mixed-halide perovskite.
We have recently found that pulsed laser irradiation of photosegregated mixed-halide perovskite films can induce robust and reproducible, persistent photoremixing. This opens the door to furthering our understanding of mixed-halide photoinstabilities since it allows for the first time, concerted studies of photosegregation and photoremixing on the same system. More importantly, it provides an important test for any model seeking to self-consistently rationalize mixed-halide perovskite photoinstabilities.
1.3-O3
Matteo Zaffalon is a Postdoctoral researcher at the Department of Materials Science of the University of Milano-Bicocca (IT), where he earned his Ph.D. in Materials Science and Nanotechnology in 2022. In 2020 he collaborated with the Nanotechnology & Advanced Spectroscopy group at the Los Alamos National Laboratory (NM, USA) working on the spectroscopic investigation of solution grown functional nanostructures for application in photonic and optoelectronic devices. His research is now focused on the spectroscopic investigation and development of novel nanomaterials for the ultrafast detection and conversion of ionising radiation for energy and medical imaging applications.
Efficiency and emission rate are two traditionally conflicting parameters in radiation detection, and achieving their simultaneous maximization could significantly advance ultrafast time-of-flight (ToF) technologies. In this study, we demonstrate that this goal is attainable by harnessing the giant oscillator strength (GOS) inherent to weakly confined perovskite nanocrystals, which enables superradiant scintillation under mildly cryogenic conditions that align seamlessly with ToF technologies. Importantly, we show that the radiative acceleration due to GOS encompasses both single and multiple exciton dynamics arising from ionizing interactions, further enhanced by suppressed non-radiative losses and Auger recombination at 80 K. The outcome is ultrafast scintillation with 420 ps lifetime and light yield of ~10’000 photons/MeV for diluted NC solutions, all without non-radiative losses. Temperature-dependent light-guiding experiments on test-bed nanocomposite scintillators finally indicate that the light-transport capability remains unaffected by the accumulation of band-edge oscillator strength due to GOS. These findings suggest a promising pathway toward developing ultrafast nanotech scintillators with optimized light output and timing performance.
1.3-O4
Colloidal lead halide perovskite nanocrystals (NCs) are very promising materials for next-generation light-emitting devices due to their large gap tunability and high emission quantum yield. At ambient conditions, metal halide perovskites, whether in bulk or nanocrystalline form, exhibit a fairly linear increase in fundamental gap with increasing temperature, when crystallized in a tetragonal or cubic phase [1]. This also holds for CsPbBr3 NCs, although they presumably crystallize in an orthorhombic phase. Strikingly, the chlorine counterpart exhibits a sign reversal in the temperature slope of the fundamental gap [2]. We combined temperature and pressure-dependent photoluminescence (PL) measurements of colloidal CsPb(Br1-xClx)3 mixed-anion NCs to unravel the origin of such a reversal. The NC composition was varied continuously by ionic exchange in the primal colloidal solution. Careful analysis of the PL data allowed us to disentangle the effects of thermal expansion and electron-phonon interaction on the variation of the gap with temperature. We show that, concomitant with a transition into an orthorhombic phase, occurring for Cl contents exceeding ca. 40%, the electron-phonon interaction undergoes a sudden and radical change in sign and magnitude. In contrast, thermal expansion effects remain the same. Based on recent observations in mixed-cation CsxMA1-xPbI3 single crystals with low Cs content [3], we interpret such behavior as due to the activation of an anomalous electron-phonon coupling mechanism linked to lattice anharmonicities induced by coupling with Cs rattling modes. This takes place in the shrunken cage voids of the orthorhombic NC lattice for high Cl concentrations. In this way, we have clarified a puzzling result about metal halide perovskite NCs, providing valuable insights into the role of A-site cation dynamics in their optoelectronic properties.
1.3-O5
Lead halide perovskites nanocrystals (PNCs), prized for their solution processability, strong light-matter interaction, tunable and high radioluminescence within visible range, are emerging high-atomic number scintillating materials for next generation scintillator/photodetectors for ionizing radiation detection. However, the challenge of embedding NCs into optical-grade nanocomposites without degrading their optical properties continues to hinder their widespread adoption. Moreover, fundamental aspects of the scintillation mechanisms remain insufficiently understood, leaving the scientific community without well-defined fabrication protocols or rational design guidelines for fully harnessing their potential. Recently, innovative strategies for embedding PNCs in impermeable host matrices have been developed [1], effectively preserving their luminescence properties in harsh environments while preventing Pb dispersion, thereby enabling their safe use in biological applications. Furthermore, studies have identified key parameters in the design of scintillation detectors at the isolated NC level, providing critical insights for optimizing their performance [2]. We investigate the embedding of PNCs within mesoporous silica particles, enabling improved stability and controlled dispersion in non-scintillating solvents. Our findings suggest that localized PNC concentration can trigger cascading scintillation, where secondary excitation enhances emission efficiency. Furthermore, this approach mitigates lead dispersion while maintaining strong radioluminescence, addressing key concerns in high-loading nanocomposite systems. This study advances the understanding of nanoscale scintillation mechanisms and provides insights into designing next-generation PNC-based scintillation detectors.
2.1-I1
Sam Stranks is Professor of Energy Materials Optoelectronics in the Department of Chemical Engineering & Biotechnology and the Cavendish Laboratory, University of Cambridge. He obtained his DPhil (PhD) from the University of Oxford in 2012. From 2012-2014, he was a Junior Research Fellow at Worcester College Oxford and from 2014-2016 a Marie Curie Fellow at the Massachusetts Institute of Technology. He established his research group in 2017, with a focus on the optical and electronic properties of emerging semiconductors for low-cost electronics applications.
Sam received the 2016 IUPAP Young Scientist in Semiconductor Physics Prize, the 2017 Early Career Prize from the European Physical Society, the 2018 Henry Moseley Award and Medal from the Institute of Physics, the 2019 Marlow Award from the Royal Society of Chemistry, the 2021 IEEE Stuart Wenham Award and the 2021 Philip Leverhulme Prize in Physics. Sam is also a co-founder of Swift Solar, a startup developing lightweight perovskite PV panels, and an Associate Editor at Science Advances.
Halide perovskites are exciting materials for next-generation optoelectronic devices including solar cells, LEDs and X-ray detectors and they show intriguing photophysical behaviour. Here, I will outline our group's recent results on the photophysics of perovskites on different length scales. This work includes nanoscale quantum behaviour at low temperature in bulk thin films, recombination seen deep within, and at interfaces of, single crystals in X-ray detector devices, charge transfer pathways in 2D perovskites with electroactive molecules and charge and energy transfer at the interface between high quality 2D/3D heterojunctions. I will show how the multimodal toolboxes can shed important light on these processes by connecting the photophysical findings directly with structural, chemical and morphological properties. This information sheds light on sources of unwanted non-radiative recombination processes, and opportunities for further functionality tuning through different compositions, structures and heterojunctions. These findings are only possible when considering physically realistic models, and such models and appropriate fitting approaches are discussed in detail.
2.1-I2
Metal halide perovskites have attracted remarkable interest as promising materials for optoelectronic applications. A key factor underpinning their performance is the behavior of photogenerated excess charges. These highly polarizable materials exhibit strong charge-lattice interactions, which can result in diverse forms of charge localization, including polarons, bipolarons, and self-trapped excitons (STEs). Such localized states play an important role in defining the optoelectronic properties of perovskites, influencing charge transport, recombination, and light emission. In this talk, I will present a series of studies that investigate the formation, stability, and dynamics of these charge-localized states, employing advanced computational approaches such as non-empirical hybrid functionals and time-dependent density functional theory. I will discuss examples from single lead- and tin-based perovskites as well as double halide perovskites. By connecting computational predictions with experimental findings, I will illustrate how polarons, bipolarons, and STEs manifest in halide perovskites and their implications for optoelectronic and photonic properties.
2.1-O1
Marie Curie (MSCA) fellow @Hofkenslab, KU Leuven, Belgium (with Prof. Johan Hofkens) | Visiting researcher@Max-Planck Institute for Polymer research, Mainz, Germany | Visiting researcher @Tokyo Institute of Technology, Japan |
Former Post doc with Prof. Ivan Scheblykin,@LundUniversity, Sweden
Ph.D with Prof. Anunay Samanta, @School of Chemistry, University of Hyderabad, India
Current research: Microscopy based method development for nanoscale imaging of operando photovoltaic and optoelectronic devices;
Optical and dielectrophoretic control of colloidal particles and 3D imaging for the development of colloidal memory.
Unlike conventional semiconductor materials, metal halide perovskites (MHP) possess soft and ionic crystal structures leading to several unique features like facile ion migration, self-healing, elasticity, and memory. Within this dynamic system, external stimuli like high photon doses, electron beams, electrical bias, and mechanical stress induce structural changes and alter associated optoelectronic properties. Therefore, it is crucial to investigate the structure-photophysics relationship in these materials, especially in operational devices like solar cells, where traditional methods such as scanning electron microscopy fall short due to the layered structure. Moreover, electron and x-ray-based analytical techniques are often invasive altering the material properties.
To address these challenges, we developed Correlation Clustering Imaging (CLIM), a novel noninvasive method that utilizes photoluminescence fluctuations to reveal contrasts associated with defect dynamics in semiconductor materials. In films, CLIM images show the polycrystalline grain structure ideally correlating with electron microscopy images. Analysis of photoluminescence fluctuations suggests the presence of one type of metastable defect dominating the fluctuations. Although the relative amplitude of PL fluctuations is small in films, it is significantly larger in solar cells under short-circuit conditions. The correlated regions within devices are notably larger (up to 10 micrometers) than those in films (up to 2 micrometers). We propose that the regions resolved by CLIM in solar cells possess a common pool of charge extraction channels, which fluctuate and cause PL to vary. Since photoluminescence fluctuations report on the dynamics of non-radiative recombination processes, CLIM offers insights into structural and functional aspects related to carrier transport, ion migration, defect formation and annihilation, and recombination losses, which are crucial for the rational engineering of the next generation of devices.
CLIM provides imaging contrasts based on properties which were never used before for optical non-invasive imaging of luminescent materials and devices based on them in operando. The broad applicability of CLIM, requiring only a standard wide-field microscope and our user-friendly, open-source algorithm, positions it as an important new tool for material chemists, engineers, and device scientists.
2.1-O2
Charge carrier recombination models based on fluence dependent time resolved photoluminescence (TRPL) datasets can provide crucial insights into the optoelectronic properties of semiconducting materials. In the case of halide perovskites, the validity of the assumed recombination model is however often challenged [1], where an ongoing debate exists on the number and type of defect populations to consider, such that a multitude of defect populations need to be assumed to reproduce experimental data even of a canonical perovskite thin film [2]. In systems with enhanced complexity, such as perovskite quantum dot solids presenting pronounced diffusion processes [3], a modelling with common recombination models fails and an alternative approach as well as a validation protocol of the assumed models is required.
Herein, we present a novel TRPL data representation method that allows to (1) extract recombination constants from complex material systems beyond common recombination models and (2) simultaneously evaluate the validity of assumed processes in the different recombination models (such as the presence and type of defects) [4]. To do so, a method that processes time-resolved datasets to absolute carrier density-resolved datasets is developed. By analyzing different derivative representations, monomolecular and bimolecular recombination processes are graphically isolated, where a rigorous comparison of experimental and simulated datasets allows their clear association to different recombination processes as well as to equilibrium and out-of-equilibrium processes. This enables, among others, a clear distinction between shallow and deep trap contributions. The proposed analysis method is applied to different systems and the extracted recombination constants are validated through simultaneously acquired fluence dependent PLQY curves.
The proposed approach provides thus a broadly applicable analysis tool that allows to differentiate between different recombination and charge transfer processes and helps to identify the dominant contributions in a given semiconducting system, with largely varying defect populations and diffusion pathways.
[1] Kiligaridis, A., Frantsuzov, P. A., Yangui, A., Seth, S., Li, J., An, Q., ... & Scheblykin, I. G. (2021). Are Shockley-Read-Hall and ABC models valid for lead halide perovskites?. Nature communications, 12(1), 3329.
[2] Yuan, Y., Yan, G., Dreessen, C., Rudolph, T., Hülsbeck, M., Klingebiel, B., ... & Kirchartz, T. (2024). Shallow defects and variable photoluminescence decay times up to 280 µs in triple-cation perovskites. Nature Materials, 23(3), 391-397.
[3] Tiede, D. O., Romero-Pérez, C., Koch, K. A., Ucer, K. B., Calvo, M. E., Srimath Kandada, A. R., ... & Míguez, H. (2024). Effect of Connectivity on the Carrier Transport and Recombination Dynamics of Perovskite Quantum-Dot Networks. ACS nano, 18(3), 2325-2334.
[4] Tiede, D. O. et al – in preparation
2.2-O1
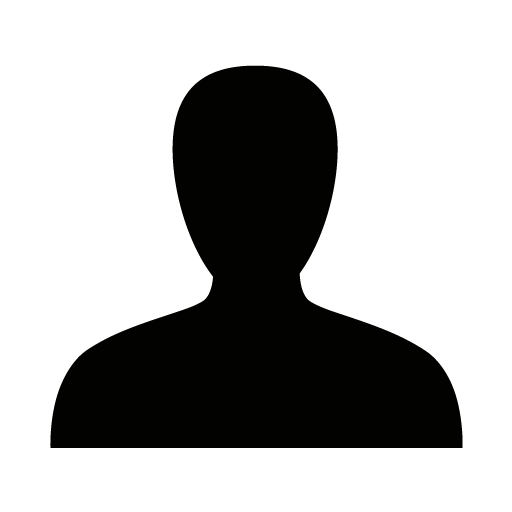
Metal-halide perovskite (MHP) semiconductors are highly relevant candidates for the fabrication of next generation solar cells thanks to their very good opto-electronic properties as well as ease of processability. That said, considerable challenges are still ahead for MHP solar cells: Indeed, under continuous external stressors, such as heat, moisture, bias and light, MHP materials suffer from various (irr)reversible chemical reactions [1, 2, 8]. These phenomena impede devices from attaining the technological readiness levels required for deployment. Therefore, it is crucial to understand the initial stages of perovskite material evolution under external stressors in order to mitigate long term degradation of devices.
As part of long-term degradation of solar cell devices, bulk absorber stability under continuous illumination is needed in order to conserve appropriate device function. Stable bulk properties would also avoid second hand reactions at interfaces with available decomposition products of the bulk [3].
Striking evidence for absorber instability under continuous irradiation has been previously described, where steady state photoluminescence (PL) either increases or decreases, depending on conditions of continuous illumination [4,5,6] or quasi-continuous illumination [7]. Additionally, these reports also highlight the crucial role of the sample environment on PL evolution under continuous illumination [8]. For all these works, the common and favored explanation for PL evolution under continuous illumination is the introduction and/or annihilation of new trapping sites which respectively causes a drop or increase in PL intensity. Though correlations to kinetic models or DFT calculations have been shown previously [7], experimental evidence for the effect of newly introduced trapping sites on the dominating recombination mechanisms at play is lacking. This is where time resolved photoluminescence (trPL) is a very useful tool: it can be used to identify what type of recombination mechanism is dominating from carrier-density dependent lifetimes, using so called differential lifetime plots [9].
In this presentation, we will focus on methylammonium-lead-iodide (MAPI) thin films and the (ir)reversible introduction of traps triggered by quasi-continuous illumination. For this, we develop a method that uses long pulses of light in combination with trPL counting schemes, calling it long pulsed trPL (LP-trPL). From the method, we observe the inclusion of long lived and non-deep trapping sites due to continuous illumination. The data also suggests a highly asymmetric mechanism of trap formation, where trap annihilation is much slower than observed formation. We conclude that previously described mechanisms of iodine outgassing [7] is compatible with the observed shallow nature of traps introduced as well as the asymmetric process of formation/annihilation.
2.2-I1
Lead halide perovskites, known for their excellent optoelectronic properties, hold significant industrial promise across applications such as solar cells, photocatalysis, and radiation detection. However, their instability and toxicity remain critical challenges. As a promising alternative, halide double perovskites, that substitute lead with elements like silver and bismuth, offer greater stability and reduced toxicity. Here, I will show how the optical, mechanical and toxicity characteristics of double perovskites can be tuned by compositional engineering. By employing a solvent-free, mechanochemical synthesis method, we circumvent common issues such as poor precursor solubility and the formation of unwanted side phases. Using synchrotron radiation, we tracked the formation mechanisms during the mechanochemical synthesis of Cs2Ag[BiM]Br6 (M = Sb, In, or Fe), identifying new intermediate phases and gaining valuable insights into the reaction kinetics.
We find that mechanochemical synthesis is a successful approach to make compounds that were not accessible via solution-based synthesis routes, such as Cs2AgBi0.5In0.5Br6, and Cs2AgBi1-xFexBr6. Hence, this solvent-free approach enables tuning of the absorption onset across the entire visible spectrum of light. In addition, high-pressure synchrotron-based X-ray diffraction (XRD) experiments revealed that the mechanical properties of these materials varies significantly with chemical composition. The improved understanding of the mechanochemical formation of alloyed-AgBi double perovskites opens new pathways for designing materials with tailored optical and mechanical properties, advancing their potential in sustainable energy applications.
2.2-I2
Non-equilibrium Bose-Einstein condensates of exciton-polaritons in metal halide perovskites present a versatile platform for fundamental and applied research. The transition to spontaneous coherence is widely believed to be driven by intricate many-body interactions between polaritons and the optically inaccessible reservoir of background excitons. To elucidate these interactions, we employ advanced optical spectroscopy techniques to probe the coherent dynamics of polaritons and identify the critical factors—ranging from material properties to multi-particle interactions—that determine the condensation threshold.
Focusing on two-dimensional Ruddlesden-Popper (RP) metal-halide semiconductors, we uncover key processes that hinder polariton condensation. Compared to the bare semiconductor, we find enhanced nonlinear exciton-exciton interactions in the microcavity environment. This is accompanied by ultrafast, parametric polariton scattering that prevents effective thermalization of sufficient population into the lowest-energy polariton state to enable condensation. Furthermore, we detect distinct spectral signatures of multi-particle correlations between polaritons and multiple excitonic transitions characteristic of RP perovskites.
Our findings suggest that the complex scattering landscape between the exciton reservoir and polaritons imposes significant limitations on polariton condensation in these materials. To address this, we propose strategies for reducing competing scattering pathways by systematically tuning the exciton fine structure via targeted organic cation substitutions. Finally, we discuss the broader implications of these results for highly polar materials, where lattice-mediated multi-particle correlations play a pivotal role in shaping many-body dynamics.
2.2-O2
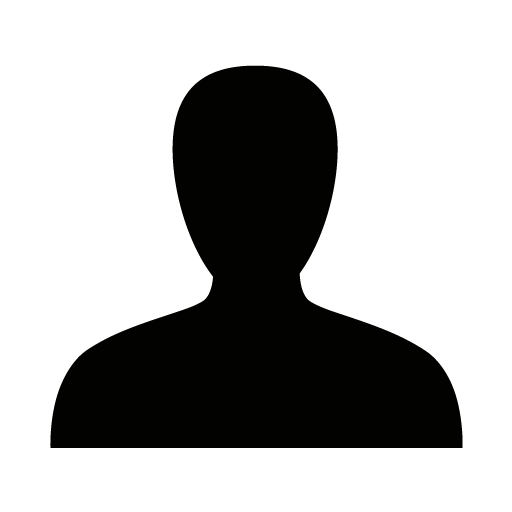
Current Position:
2020-Present – Senior Lecturer of Chemistry – Institute of Chemistry and The Center for Nanoscience and Nanotechnology, The Hebrew University of Jerusalem, Israel
Research Objectives:
Research and development of novel soft-semiconductors for light emission and X-ray detection
Research of novel soft-semiconductor materials and development of functional devices based upon them
Study fundamental processes and basic properties of functional materials – optical and electrical spectroscopy and microscopy
Development of composite semiconductors and devices based upon them
Education:
2016-2020 – Post-doctoral scholar – "Research and Development of Low-Cost and Air-Stable Solar Cells, Detectors and Light Emitting Devices" – Prof. Mercouri Kanatzidis Lab, Department of Chemistry, Northwestern University (Evanston, IL, USA)
2011–2016 – Ph.D. – Physical Chemistry – "Dimensionality Effects in Semiconductor Nanorods – Optical Study from Single Particles to Ensemble" – under the supervision of Prof. Uri Banin, The Hebrew University of Jerusalem (IL)
2010-2011 – M.Sc. (within the direct Ph.D. track) – Exact Science, The Hebrew University of Jerusalem (IL)
2006-2009 – B.Sc. – Exact Science (Physics and Chemistry), The Hebrew University of Jerusalem (IL)
Hybrid halide perovskites are a novel class of semiconductor materials with promising and versatile optoelectronic properties enabled by their chemically adjustable structures and dimensionality. The diversity in the metal ions, halide anions, and organic spacers enables a wide range of materials with highly tunable properties and variable dimensionalities. These materials are studied for various applications, such as photovoltaics, detectors, and light-emitting devices. The chemical and structural agility of halide perovskites, enabling the adjustment of the optical and electronic properties for a desired application, is significant. In our research, we seek to gain additional information regarding the correlation between structure and composition to optoelectronic properties in low-dimensionality halide perovskites.
Specifically, we study low-dimensionality hybrid halide perovskites that exhibit broad-spectrum, white-light emission at room temperature, associated with self-trapped excitons (STE). These compounds are ideal candidates for illumination applications. We study the correlation between structural and chemical motifs of low-dimensionality halide perovskites and their STE emission.
In this research, we have studied how exchanging the halide anions while maintaining the structure affects the STE properties. We have focused on a unique 1D perovskite structure based on edge-sharing dimers, exhibiting strong, broad emission with PLQY of approximately 40%. By changing the halide from I to Br and Cl, we observe an increase in the bandgap energy, as expected. However, the broad emission shows an anti-correlated behavior, resulting in red-shifted broad emission for the Cl sample, with a significantly larger stokes shift. We further study how mixing Br and Cl in a single structure affects the broad emission properties and how different synthetic approaches can be utilized to fabricate these compounds.
To gain additional information regarding the STE properties with different compositions we have studied the temperature-dependent photoluminescence of these compounds. We utilize combined spectrally and temporally resolved photoluminescence measurements, allowing us to study the transition from band-edge to STE emission upon excitation. We observed how this transition, along with additional properties of the STE emission, evolved with temperature and composition.
2.2-O3
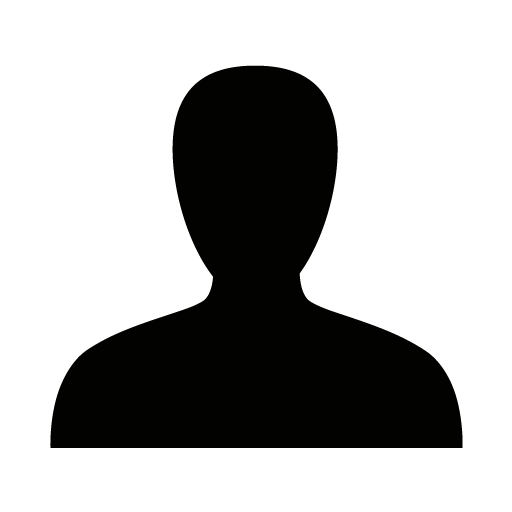
Two-dimensional (2D) perovskites are of great scientific interest as they have exceptional optoelectronic properties and are easily tunable in their bandgap, making them viable to be used for a variety of optoelectronic devices, such as LEDs, solar cells and photodetectors. 2D perovskites have a multi-quantum well structure, with alternating layers of insulating organic spacer molecules and inorganic perovskite layers, with varying thickness given by the integer n. As a result, they have narrow excitonic features and large exciton binding energies of up to several hundred meV. This begs the question of how the excitonic dissociation in 2D perovskites works, as they show efficient charge carrier separation, despite the large excitonic binding energy.
For n>1 2D perovskites, so called edge states have been observed along the edges of single crystal flakes and the grain boundaries of thin films. The excitons dissociate into these edge states resulting in an efficient charge carrier separation. They show a red-shifted photoluminescence (PL), which dominates the PL signal in perovskite thin films. In n=1 2D perovskites, these states have not been observed. However, previously we demonstrated that even in n=1 2D perovskites efficient charge carrier separation takes place, enabling a 9 % external quantum efficiency in a photoconducting photodetector. [1]
In this work we provide insights into the process of charge carrier separation in n=1 2D perovskites using spatially resolved photocurrent measurements. We correlate photocurrent and PL, while exciting locally with a focused laser beam with a resolution of less than 1 µm. We observed higher photocurrents along the grain boundaries compared to the inside of the grains of butylammonium lead iodide (BA2PbI4). At the same time, the PL is less bright at the grain boundaries with the peak maximum being shifted slightly red and the peak being broader. This supports the hypothesis that edge states are existent within n=1 2D perovskites, even though they are not as easily observable in PL as in n>1 2D perovskites, and that they are crucial for the charge carrier separation. With our contribution we hope to shed light on the exciton dissociation in n=1 2D perovskites with the aim to improve device efficiency for those materials.
2.3-I1
Paulina Plochocka, Directrice de recherché de 2e classe (DR2) in Laboratoire National des Champs Magnétiques Intenses (LNCMI), CNRS in Toulouse.
P. Plochocka obtained her PhD cum-laude in 2004 at the University of Warsaw working on the dynamics of many-body interactions between carriers in doped semi-magnetic quantum wells (QW). During her first post doc at Weizmann Institute of science, she started working on the electronic properties of a high mobility 2D electron gas in the fractional and integer quantum Hall Effect regime. She continued this topic during second post doc in LNCMI Grenoble, where she was holding individual Marie Curie scholarship. At the same time, she enlarged her interest of 2D materials towards graphene and other layered materials as TMDCs or black phosphorus. In 2012 she obtained permanent position in LNCMI Toulouse, where she created the Quantum Electronics group, which investigates the electronic and optical properties of emerging materials under extreme conditions of high magnetic field and low temperatures. Examples include semiconducting layer materials such as transition metal dichalcogenides, GaAs/AlAs core shell nanowires and organic inorganic hybrid perovskites.
High environmental stability and surprisingly high efficiency of solar cells based on 2D perovskites have renewed interest in these materials. These natural quantum wells consist of planes of metal-halide octahedra, separated by organic spacers. The unique synergy of soft lattice and opto-electronic properties are often invoked to explain superior characteristic of perovskites materials in applications. At the same time such unique synergy creates fascinating playground for exciton physics which challenges our understanding of this elementary excitation. I will demonstrate that even after decade of intense investigation the notation” unique” so often used in case of perovskites deserves serious scrutiny.
I will explore the excitonic landscape in 2D semiconductors. First, I will highlight the controversy surrounding the unexpectedly high light emission efficiency of this material and show that it can be explained by the interplay between phonons and the exciton fine structure. I will demonstrate that the soft lattice can suppress relaxation of excitons to dark state making 2D perovskites great light emitters. Moreover, I will discuss the exciton fine structure measured for multiple 2D layered perovskites characterized by a different lattice distortions imposed by organic spacers. Surprisingly, it has a non-trivial impact on the exchange interaction allowing the energy spacing between dark and bright excitons to be tuned. This tuning knob, not available in classic semiconductors, makes 2D perovskites a unique material system where the exciton manifold can be controlled via the steric effect. Finally, I will demonstrate the first experimental evidences of polaron formation in the optical spectra of these materials.
2.3-I2
Sascha is a Tenure-Track Assistant Professor in Physical Chemistry and Head of the Laboratory for Energy Materials at EPFL (Switzerland), while he is also maintaining strong ties with the Harvard community and in particular Winthrop House which he regularly visits as NRT and SCR member.
His team employs light-matter interactions to understand the next generation of soft semiconductors with the overarching goal of maximizing energy efficiency for a sustainable future by unlocking applications ranging from flexible light-weight solar cells & displays all the way to entirely new applications in quantum information processing.
Previously, he was a research group leader and Rowland Fellow at Harvard University. Before starting his lab at Harvard, Sascha studied Chemistry at Heidelberg University (Germany) and completed a PhD in Physics at the University of Cambridge (UK), where he subsequently worked as EPSRC Doctoral Prize Fellow.
Halide perovskites are fascinating semiconductors for light-emitting applications. Compared to conventional inorganic covalent semiconductors like silicon or GaAs, perovskites are structurally soft and often more disordered. Understanding the consequences of this remains a key challenge for commercialization but offers also opportunities for tailoring properties to target applications.
Here I will present our recent mechanistic insights from spectroscopy on the role of composition, doping and dimensionality to control light emission through localization effects in these materials.
I will talk about charge carrier accumulation in mixed-halide 3D systems, compare the photophysics of 2D Pb with that of new 2D germanium perovskites, and, time permitting, will introduce a new synthesis route to ambient doping of 0D nanocrystals giving for the first time access to strongly confined, transition-metal doped perovskite nanocrystals, with profound consequences for the light emitting properties of the resulting materials.
Overall I hope to highlight the promise the vast tunability of this material class holds for next-generation optoelectronic applications, once their working mechanisms are better understood.
2.3-O1
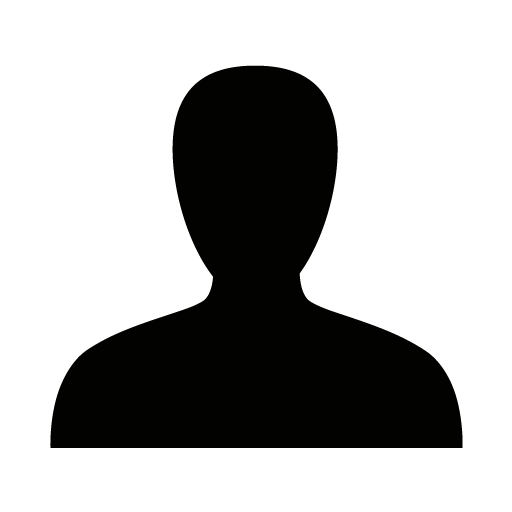
The interest in lead halide perovskite materials has increased over the last decade. Understanding the fundamental photo-physical and chemical nature of these materials is of great interest as they have great potential in the application in solar cells or light emitting devices (LEDs). Therefore, lead halide perovskite nanocrystals (NCs) will be the most relevant material. Their optical tunability by anion exchange, high photoluminescence quantum yield and their defect tolerance make them outstanding. However, there is still lack of understanding the chemical nature surrounding the inorganic NC. Therefore, it is necessary to investigate how the electronic structure of this type of NCs can be influenced by the organics and, ultimately, the devices can be improved.
In this work, we investigate cinnamic acid covered CsPbBr3 NCs to study their absolute band edge position by spectroelectrochemistry (SEC). Kroupa et al. have previously a shift of the valence band of PbS nanoparticles by introducing electron withdrawing or donating functional groups to cinnamic acids.[1] Here, we can also observe a shift of the valence band. In contrast to PbS, electron withdrawing ligands facilitate the oxidation of NCs compared to electron donating ligands. A shift of 0.34 V can be extracted from SEC in photoluminescence. Combining this result with theory, the earlier oxidation can be explained by a shift of the HOMO to the cinnamic acid derivative. Depending on the strength of the electronic properties of the aromatic ligand, the HOMO is more or less located on the ligand, whereas this is not the case for PbS.[1] This result (of the easier oxidation of CACF3 covered NCs) is supported by data from LEDs. In particular, focusing on the hole transport layer gives insight into the charge injection depending on the ligand surrounding the NCs.
1.3-I1
Prof. Dieter Neher studied physics at the University of Mainz. In 1990 he gained his PhD with Prof. G. Wegner. From 1990-1992 he was a research associate at the Optical Sciences Centre, Tucson, Arizona and at the Centre for Research in Electrooptics and Lasers, Orlando, Florida with Prof. G. Stegeman. 1992 he joined again Prof. G. Wegner at the MPI-P, heading the group Electrooptical Phenomena in Polymers. Following his habilitation in November 1998, he became Professor of Soft Matter Physics at the Institute for Physics and Astronomy at the University of Potsdam. Current research interests are electrical, optical and optoelectronic processes in conjugated materials.
Since the advent of low bandgap non-fullerene acceptors (NFAs), the performance of organic solar cells (OSCs) has improved significantly. A critical parameter is the offset between the relevant frontier orbitals at the DA heterojunction, which in most NFA-based blends is the difference in HOMO energies. Here we combine a wide range of methods, from femtosecond transient absorption to steady state photoluminescence and electroluminescence, spectroscopy to study the mechanisms and efficiency of free charge generation and recombination [1]. For a wide series of NFA-based OSCs, we find that the singlet exciton decay is the main competing pathway for free charge generation while reformation of singlet excitons from reformed CT states dominates the radiative recombination in EL. To explain our data as function of the HOMO offset, we set up a 5-state model which includes singlet and triplet excitons. Our results show that the optimal range of the energy offset for achieving optimal performance is quite narrow and that without additional means for efficient photon harvesting, the power conversion efficiency is limited to around 20 %.
1.3-O1
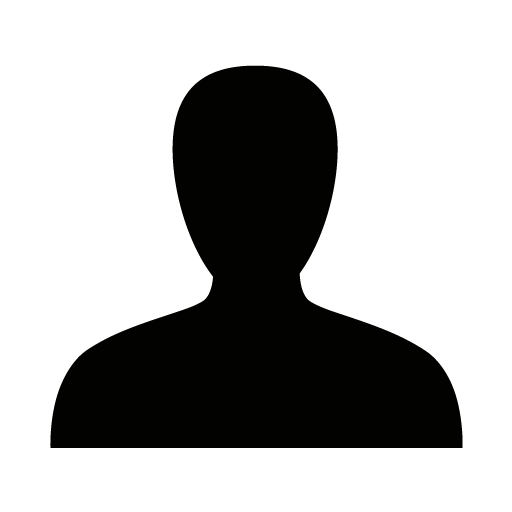
Recent progress, particularly with Y-series non-fullerene acceptors (NFAs), has propelled the power conversion efficiency (PCE) of single-junction organic photovoltaics (OPVs) beyond 19%.[1] This improvement pushes the need to design new donor polymers that effectively pair with NFAs. However, many high-performing donor polymers are expensive due to complex synthesis processes and costly raw materials. Thus, the design of low-cost donor polymers is crucial for large-scale production and commercial adoption. In this study, we explored the charge generation mechanism in new low-cost donor polymer FO6-T blends, which presents high crystallinity challenges and new potentials for efficient OPVs. When blended with the NFA L8BO, FO6-T achieves a notable PCE of 15%.[2] We identified two distinct polaron states in FO6-T: one associated with disordered domains and the other with crystalline domains, as evidenced by cyclic voltammetry (CV) and spectroelectrochemistry (SEC) measurements. These findings align with the reported FO6-T’s semi-crystalline morphology3. To gain deeper insights, transient absorption spectroscopy (TAS) was employed across timescales from femtoseconds (fs) to seconds (s) to investigate charge dynamics within these domains. Upon photoexcitation, FO6-T excitons rapidly converted into polaron states within a few picoseconds (ps). Polaron states in the disordered domains near the donor/acceptor (D/A) interface transferred to crystalline phases via an energy cascade within 20 ps. The crystalline phases played an important role in stabilizing the separated polarons, which exhibited lifetimes extending into the microsecond (μs) range, as shown by μs-TAS measurements. Then we studied the high-performing FO6-T:L8-BO. The two polaron states of FO6-T persist in the FO6-T/L8-BO blend, revealed by fs-TAS. Furthermore, Förster resonance energy transfer (FRET) was observed under donor excitation, supported by the TAS kinetic analysis. The highly overlapping emission spectrum of FO6-T and the absorption spectrum of L8-BO also support the FRET observation. To investigate the impact of findings discussed above on device performances, a series of D/A ratio studies was conducted. The FO6-T/L8-BO blend exhibited exceptional tolerance to D/A ratios ranging from 2:1 to 1:10. These findings underscore the potential of low-cost, simple donor polymers like FO6-T in achieving high-efficiency and semi-transparent OPVs, paving the way for broader commercialization and innovative applications.
1.3-I2
The inverse design of tailored organic molecules for specific optoelectronic devices of high complexity holds an enormous potential but has not yet been realized. Current models rely on large data sets that generally do not exist for specialized research fields. We demonstrate a closed-loop workflow that combines high-throughput synthesis of organic semiconductors to create large data sets and Bayesian optimization to discover new hole-transporting materials with tailored properties for solar cell applications. The predictive models were based on molecular descriptors that allowed us to link the structure of these materials to their performance. A series of high-performance molecules were identified from minimal suggestions and achieved up to 26.2% (certified 25.9%) power conversion efficiency in perovskite solar cells.
That milestone underlines the feasibility of developing autonomous research strategies that discover materials tailored for specific applications. That requires a highly interconnected workflow including synthesis, purification, characterization and device optimization. Such lines could specifically develop optimized interface materials for perovskite cells with various bandgaps, but also discover optimized interfaces for LEDs, photodetectors or X-Ray detectors. The outlook will summarize the advantages but also the limitations of data driven methods and will give further examples of such campaigns searching to find optimized materials for very different applications
1.3-O2
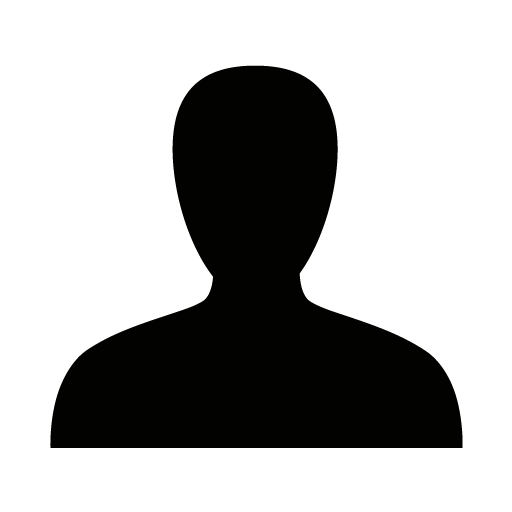
Whilst organic solar cells (OSCs) have now reached efficiencies necessary for commercialization, their current high cost is prohibitive for most applications. One exception to this is semi-transparent OSCs, where the tuneable absorption of the active layer can be uniquely exploited to create power generating, semi-transparent energy sources. These hold great potential for building integrated photovoltaics, and net-zero architecture. A common way to achieve this transparency is via reduced donor content, in so called ‘dilute donor’ cells.
In this work we explore printing such dilute donor systems, using donor PTQ10 and acceptor Y12, via blade coating; alongside structural analysis to understand the relationship between printing conditions and photovoltaic performance.
We show results from a custom, blade coater setup used to measure in-situ grazing incidence wide angle x-ray scattering (GIWAXS) at the Diamond Light Source synchrotron, under a range of conditions. We see differences in crystallization and drying dynamics depending on stage temperature and donor content, and relate these to device performance for blade coated OSCs. Grazing incidence small angle x-ray scattering (GISAXS) and a range of device based optoelectronic measurements are also shown to provide an overview of structure-function relationships. We believe this work provides an important insight into how blade-coating conditions impact film morphology, and therefore how printing conditions can be designed for optimum semi-transparent OSCs.
1.3-O3
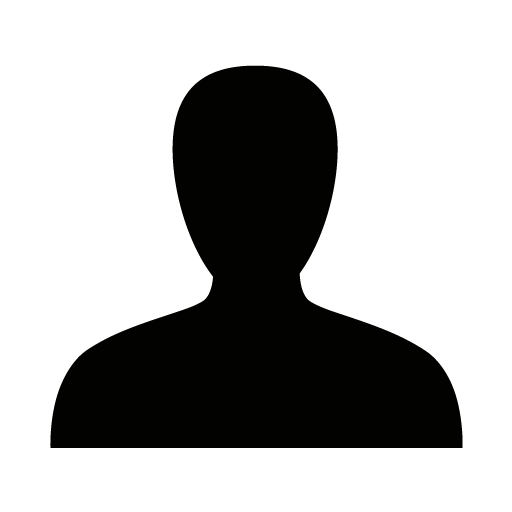
Organic solar cells (OSCs) have demonstrated significant improvements in power conversion efficiency, surpassing 20%, largely due to the development of non-fullerene acceptors (NFAs).[1] However, the limited operational device stability continues to present challenges for the organic photovoltaic community.[2,3,4,5] Here, we investigated the photodegradation of thin films of the donor PTQ10, the NFA Y6, and their blends under AM 1.5 illumination using a solar simulator in ambient conditions. We found that the pristine PTQ10 and Y6 exhibit relatively higher photochemical stability compared to the PTQ10:Y6 blend after 45 hours of degradation. When the blend PTQ10:Y6 is exposed to light through a 400 nm long-pass filter, the degradation rate significantly decreases, indicating the influence of UV light on the blends. To address this, we incorporated a third component, PC70BM into the active layer of the PTQ10:Y6 blend. The results show that the degradation rate in white light (AM 1.5) on the ternary blend (PTQ10:Y6:PC70BM) has the same rate as 400 nm long-pass filtered light on the binary blend (PTQ10:Y6), suggesting that the addition of PC70BM does not mitigate UV-induced degradation. These results are confirmed by UV-vis absorption spectra and IR spectra. The atomic force microscopy images show that after 45 hours of degradation, a slight increase in the roughness of the films is observed. The addition of a third component could be an effective way to improve the photostability of the active layer used in OSCs.
1.3-O4
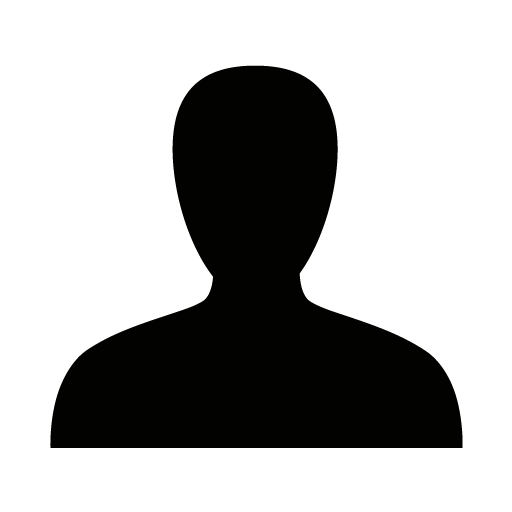
Semi-transparent organic photovoltaics (ST-PV) have gathered significant attention due to the unique ability to simultaneously convert sunlight into electricity while allowing visible light transmission, together with their ultra-light weight, flexibility, and process scalability. With this, potential applications such as agrovoltaics, photovoltaic windows or building faceds have been highlighted by researchers and industry.
Microalgae are photosynthetic microorganisms that are able to convert light and nutrients into biomass made of various valuable molecules such as lipids and proteins for the production of sustainable advanced fuels (SAF). In the context of COCPIT project (https://www.cocpit-horizon.eu/https://www.cocpit-horizon.eu/), microalgae biomass productivity could be maximized using thin film photobioreactor (PBR) in solar conditions such as the AlgoFilm technology. Optimization of the use of the sunlight collected at the culture system surface, by filtering the NIR and IR spectrum with a ST-PV, is considered to prevent PBR overheating, while enabling the dual valorisation of solar spectrum for both biomass and electricity production.
In this work, different active layer blends have been proposed to adapt and complement the ST-PV absorbance spectrum of Parachlorella kessleri (i.e. green microalga known for its interest in SAF production) absorbance spectra requirements. Lab-scale OPV devices were fabricated using a scalable deposition technique such as slot-die. Tests were first performed in terms of efficiency and transparency and further evaluated in combination with 6 lab-scale PBRs in parallel. The selection of the final active layer was based on a compromise between transparency, efficiency and microalgae productivity.
2.1-I1
Mariano Campoy Quiles´s research is devoted to the understanding and development of solution processed semiconductors for energy and optoelectronic applications. He and his team have built substantial research efforts in two application areas, solar photovoltaic (light to electric) and thermoelectric (heat to electric) energy conversion based on organic and hybrid materials. He studied physics at the Univesity of Santiago de Compostela, obtained his PhD in experimental physics from Imperial College London, and since 2008 he leads his team at the Institute of Materials Science of Barcelona.
Single-junction organic solar cells (OSC) nowadays have reached promising power conversion efficiencies around 20%. Besides new materials, going beyond the current efficiencies could, in principle, be achieved by multi-junction devices, which promise a reduction in thermalization losses [1]. For this promise to become a reality, two items should be addressed, namely, the multi-junction geometry and the screening of materials with very different gaps.
In this talk, we will present a multi-junction in-plane spectral splitting geometry that we call Rainbow solar cells [2]. In this geometry, a series of sub-cells are placed next to each other laterally, and illuminated through an optical component that splits the incoming white beam into its spectral components, thus matching local spectrum and absorption for each sub-cell. The fabricated n-terminal devices are capable of extracting the maximum power of each sub-cell without the need for current matching nor processing challenges. We demonstrate the concept for PM6:IO-4Cl and PTB7-Th:COTIC-4F blends, as high and low band-gap sub-cells, respectively. In agreement with simulations, we show an efficiency increase of around 30% of the Rainbow geometry with respect to our best single junction device [2]. Then, we use high throughput methods based on gradients on the parameters of interest and blade coating [3-5] to screen tens of materials exhibiting either wide band gap [4] or narrow bandgap [5], and thus push the efficiency of the Rainbow multi-junction further up. Finally, we evaluate the potential of ternary mixing to further improve the efficiency of rainbow solar cells. Material design rules for this type of device will then be revisited.
2.1-I2
Ji-Seon Kim is Professor of Solid State Physics and Director of the Plastic Electronics Centre for Doctoral Training (https://www.imperial.ac.uk/plastic-electronics/) at Imperial College London. She has previously taken up an EPSRC Advanced Research Fellowship at the University of Cambridge, obtained a PhD in Physics in 2000. Her research focuses on the basic science and technology of Nanoscale Functional Materials such as organics, organic/ inorganic hybrids, nanomaterials and their related applications, as well as developing novel Nanometrology for these functional materials (http://www.imperial.ac.uk/nanoanalysis-group).
Organic photovoltaics (OPVs) have rapidly improved in efficiency, with single-junction cells now exceeding 19% efficiency. These improvements have been driven by the adoption of new non-fullerene acceptors (NFAs) and the fine tuning of their molecular structures. Although OPVs are highly efficient, they often show extremely poor operational stability, primarily owing to the complex interplay between the morphological instability of the blended bulk heterojunction photoactive layers and the intrinsically poor photostability of the organic semiconductor materials themselves. To realize commercialization, it is vital to understand the degradation mechanisms of these organic materials to improve their stability [1]. Efficiency increases have, in part, been driven by the rational molecular design of materials. In this talk, I will discuss key molecular design parameters and show how each parameter impacts different degradation pathways with a particular focus on NFA molecular planarity, rigidity, and end groups [2-4] and polymers [5]. I will also discuss the impact of morphological and photochemical instabilities on OPV device stability. The fundamental understanding of the molecular origin of OPV stability is a key research theme for next-generation OPVs.
2.1-I3
Thuc-Quyen Nguyen is a professor in the Center for Polymers and Organic Solids and the Chemistry & Biochemistry Department at University of California, Santa Barbara (UCSB). She received her Ph.D. degree in physical chemistry from the University of California, Los Angeles, in 2001 under the supervision of Professor Benjamin Schwartz. Her thesis focused on photophysics of conducting polymers. She was a research associate in the Department of Chemistry and the Nanocenter at Columbia University working with Professors Louis Brus and Colin Nuckolls on molecular self-assembly, nanoscale characterization and molecular electronics. She also spent time at IBM Research Center at T. J. Watson (Yorktown Heights, NY) working with Richard Martel and Phaedon Avouris. Her current research interests are structure-function-property relationships in organic semiconductors, sustainable semiconductors, doping in organic semiconductors, interfaces in optoelectronic devices, bioelectronics, and device physics of OPVs, photodetectors, and electrochemical transistors. Recognition for her research includes 2005 Office of Naval Research Young Investigator Award, 2006 NSF CAREER Award, 2007 Harold Plous Award, 2008 Camille Dreyfus Teacher Scholar Award, the 2009 Alfred Sloan Research Fellows, 2010 National Science Foundation American Competitiveness and Innovation Fellows, 2015 Alexander von Humboldt Senior Research Award, 2016 Fellow of the Royal Society of Chemistry, 2015-2019 World’s Most InfluentialScientific Minds; Top 1% Highly Cited Researchers in Materials Science by Thomson Reuters and Clarivate Analytics, 2019 Fellow of the American Association for the Advancement of Science (AAAS), 2023 Wilhelm Exner Medal from Austria, 2023 Fellow of the US National Academy of Inventors, 2023 de Gennes Prize in Materials Chemistry from the Royal Society of Chemistry, 2023 Elected Member of the US National Academy of Engineering, 2024 Fellow of the European Academy of Sciences, and 2025 ACS Henry H. Storch Award in Energy Chemistry.
Bulk heterojunction organic solar cells (BHJ OSCs) potentially can offer low cost, large area, flexible, light-weight, clean, and quiet alternative energy sources for indoor and outdoor applications. OSCs using non-fullerene acceptors (NFAs) have garnered a lot of attention during the past few years and shown dramatic increases in the power conversion efficiency (PCE). PCEs higher than 20% for single-junction systems have been achieved, but the device lifetime is still too short for practical applications. Thus, understanding factors that affect the OSC long-term stability is crucial. In this talk, I will discuss the impact of different blend materials and device structures on the stability. A combination of characterization methods such as solid state Nuclear Magnetic Resonance (NMR), 4D TEM, GIWAXS, resonant soft X-ray scattering (RSoXS), AFM, X-ray photoelectron spectroscopy (XPS), Electron paramagnetic resonance (EPR) spectroscopy, and capacitance spectroscopy are employed to gain insight into the device stability. We propose strategies to improve the device stability.
2.2-I1
Morten Madsen, Professor wsr at the University of Southern Denmark, SDU NanoSYD.
My field of expertise is thin-film growth, integration and devices for energy conversion and storage applications. In 2010-2011, I worked with high performance transistors from III-V nanoscale membranes at the Javey research lab, UC Berkeley, California. In 2011, I established the OPV group at SDU NanoSYD, where we work on improving the performance and stability of organic and hybrid solar cells, including thin film synthesis, metal oxide interlayers and interfaces, organic and hybrid active layers as well as film and device degradation. Since 2016, we also have a focus on device up-scaling through Roll-to-Roll (R2R) printing technology at the SDU R2R facility. Vist out site for more details:
https://www.sdu.dk/en/om_sdu/institutter_centre/c_nanosyd/forskningsomrader/organic+solar+cells
The power conversion efficiency of organic photovoltaics (OPV) has recently crossed the 20% milestone, placing an even larger focus on degradation processes and device stability. Here, recent results on oxide-organic as well as 2D MXene-organic interfaces in scalable OPV will be presented. Transition metal oxides serve as efficient charge carrier selective interlayers for electron and hole extraction in organic photovoltaic devices. However, interlayer related instabilities have been reported as a degradation route for high performing non-fullerene acceptor OPV devices, making a thorough understanding of such interfaces important for the further development of OPV technology. Recent progress made within sputtered oxide charge extraction and transport interlayers for OPV devices will be presented. Supported by a variety of surface science characterization techniques, the effect of e.g. oxide composition, microstructure and defect states on the performance of sputtered oxide interlayers in organic photovoltaic devices will be discussed [1,2]. In addition, the use of 2D MXene to tune interfaces between active layers and transport layers in OPV will be presented [3], demonstrating how such 2D layers can be employed to passivate interface defect states, and from that improve efficiency and in particular device stability. Finally, routes for Roll-to-Roll (R2R) up-scaling and manufacturing of OPV will be presented. This includes development of the hybrid interfaces using industrial-compatible Sheet-to-Sheet (S2S) and R2R techniques, and the scale up from cells to modules using various device configurations. This part also includes examples on development of scalable transparent tandem solar modules reaching power conversion efficiencies of 12.3% for an average visible transmittance of 30%, developed in the EU project CITYSOLAR.
2.2-I2
Control of the molecular configuration at the interface of an organic heterojunction is key to the development of efficient optoelectronic devices. Due to the difficulty in characterizing these buried and (likely) disordered heterointerfaces the interfacial structure in most systems remains a mystery. Here, we demonstrate a novel synthetic strategy to design and control model interfaces, allowing for their detailed study in isolation from the bulk material. This is achieved by the synthesis of a polymer in which a non-fullerene acceptor moiety is covalently bonded to a donor polymer backbone using dual alkyl chain links, constraining the acceptor and donor units in a ‘through space’ co-facial arrangement. The constrained geometry of acceptor relative to electron rich and poor moieties in the polymer backbone can be tuned to control the kinetics of charge separation and the energy of the resultant charge-transfer state giving unprecedented insight into factors that govern charge generation at organic heterojunctions.
2.2-I3
Professor of Materials Physics at Karlstad University, Sweden, since 2011. Research interests: morphology of conjugated polymer thin films, photodegradation of OPV materials, energy level allignment in organic and perovskite multilayer structures. Employed at Karlstad university since 2000. Previously Research Scientist at Cambridge Display Technology in Cambridge,UK, and Research Assistant at University of Cambridge. Post-doc at EPFL Lausanne (1996-98) and TU Delft (1995-1996). PhD degree from the Weizmann Institute of Science in Rehovot, Israel.
The organic photovoltaics (OPV) field has seen a boost in performance with the introduction of small-molecule electron acceptors (SMA), such as Y5 and Y6, leading to power conversion efficiencies above 19%[1]. For these SMAs new challenges come about due to their tendency to self-aggregate. One strategy to limit aggregation and promote miscibility with the donor is the use of Y-based copolymers as electron acceptors, yielding high-efficiency all-polymer solar cells.[2] We have shown earlier [3] by temperature-dependent optical spectroscopy of solutions of PBDB-T and the polymer acceptor PF5-Y5 that donor and acceptor interact even at low concentrations.
Here we will first present results from morphological analysis of acceptor films by angle-resolved Near-Edge X-ray Absorption Fine Structure (NEXAFS) spectroscopy, with focus on the orientation of planar SMAs Y5 and Y6 in pristine spin-coated films. We found that the processing solvent is a key parameter to obtain preferentially face-on orientation. Face-on orientation was also found for films of the Y5-based copolymers PF5-Y5 and PYT. Due to the presence of nitrogen in the acceptor N K-edge NEXAFS spectroscopy could be used to probe the orientation of acceptor molecules in the bulk heterojunction films, where they are blended with the donor polymer PBDB-T, where nitrogen is absent. We find that the surface of these blend films is donor-rich and the polymer acceptors PF5-Y5 and PYT retain the face-on orientation that they have in neat films also in the blend films.[4]
Furthermore, we will present our findings on the photochemical stability of the acceptor materials. We studied the evolution of optical properties, composition, and energy levels, during one-sun (AM1.5) illumination in air of thin films of the small-molecule acceptor Y5 and its copolymer counterparts PF5-Y5 and PYT, along with their blends with PBDB-T.[5] We found that the copolymer PF5-Y5 undergoes rapid photooxidation, while the PYT film degrades much slower and the Y5 film properties remain almost intact, even after 30 hours of light exposure in air. For Y5 molecular packing and aggregation may protect the small molecule film from photodegradation. However, the significantly faster photodegradation of PF5-Y5 compared to PYT indicates that the BDT moiety in PF5-Y5 accelerates its photooxidation. These insights on the effects of intentional photodegradation on materials properties are expected to contribute to the design of stable acceptors for long-lived OPV devices.
2.2-O1
Squaraine (SQ) dyes have gained significant attention in the scientific community due to their versatility, tunable structures, photothermal stability, and exceptional photophysical properties, including their ability to exhibit fluorescence under specific conditions.[1] SQ dyes feature a donor-acceptor-donor (D-A-D) architecture, where the donor part is an electron-rich group, typically an aromatic or conjugated structure, that donates electrons upon excitation. The central core, typically a butanedione group, is electron-deficient and serves as the acceptor unit (A), enabling efficient electron transfer from the donor to the acceptor upon excitation.[2,3] To stabilize the D-A-D system, strong electron-donating groups, such as heterocycles or electron-rich aromatic units like aniline derivatives, are often incorporated into squaraine dyes. Symmetric SQ dyes have two equivalent donor units, while unsymmetrical squaraine (USQ) dyes feature two different donor units.[4]
In this study, several unsymmetrical squaraine (USQ) dyes were synthesized using a simple two-step method involving a condensation reaction between electron-rich aromatic amines and squaric acid, which minimizes byproducts and simplifies purification. The synthesized products were characterized using 1H-NMR, 13C-NMR and FT-IR to confirm their structures. The absorption and emission properties of the dyes were evaluated through UV-Vis and fluorescence spectroscopy.
Photovoltaic (PV) devices were fabricated by depositing the cell components using thermal vapor deposition (< 5.0x10-6 Torr) on pre-patterned indium tin oxide (ITO) glasses (c.a. 100 nm).[5] The structure of the assembled cell consist of 8 nm layer of MoO3 as hole-extracting layer (HEL), a 40 nm co-deposition of the SQ dye with fullerene (C60) as the active layer, a 8 nm layer of BPhen as the electron transport layer (ETL) and 8-hydroxyquinolinato lithium (Liq) as electron extracting layer (EEL) and a final 100 nm aluminum electrode. These devices exhibited homogeneous morphologies that reduced charge recombination. The resulting PV cell achieved an average power conversion efficiency (PCE) of 3.38%, attributed to the optimal alignment of HOMO/LUMO levels and the spectral synergy between SQ dyes and C60 fullerenes. This study provides essential guidelines for optimizing these materials to further enhance the performance of SQ-based photovoltaic cells.
2.2-O2
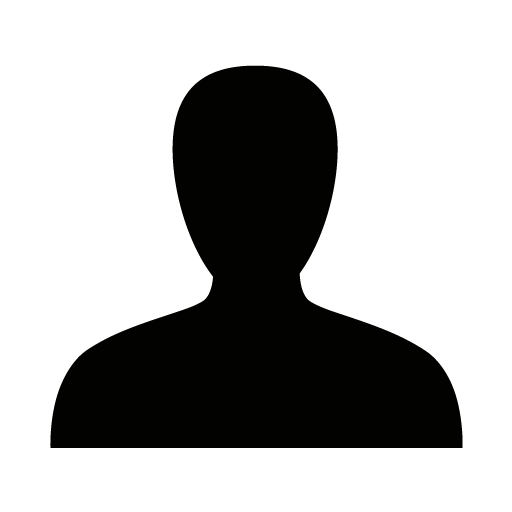
Despite having similar power conversion efficiency limits according to detailed balance, the performance of Y6 in organic photovoltaic blends still outshines that of its narrower bandgap derivatives, as shown in the TOC plot adapted from ref [1]. An understanding of the underlying mechanisms is needed to tap into the potential of low-bandgap non-fullerene acceptors (NFAs) for tandem applications and beyond. Thus motivated, we scrutinize two low-bandgap NFAs – BTPV-eF-eC9 and BTPV-4Cl-eC9 – in binary devices from the perspective of photocurrent generation, and resolve the losses incurred at each step between photon absorption and carrier extraction. With a combination of steady state and time-resolved optoelectronic techniques, we find that in these acceptors, the kinetic competition between charge-transfer state decay and its separation is a major limiting role in the overall photon harvesting capability of the devices. Despite having similar voltage losses as PM6:Y6, which we attribute to efficient charge-transfer formation at lower driving forces, the geminate loss pathway via the charge-transfer state curbs fill factor and photocurrent. This reinforces the notion that suppression of geminate loss pathways can vitally balance recombination losses with improved charge generation, and markedly move the overall internal quantum efficiency towards the detailed balance limit.
2.3-I1
Non-fullerene acceptors (NFAs) are exciting molecules allowing high efficiency in organic photovoltaic (OPV) blends with conjugated polymers. Interestingly, charges can also be generated by neat NFA films without additional donor. To understand the origins of exciton dissociation in neat NFAs, we have looked at the impact of aggregation, external electric field and non-linear effects. We used solvatochromism in order to gain insight on charge redistribution after excitation in isolated NFAs. We found that unaggregated NFAs feature a more dipolar excited state, revealing intramolecular charge transfer (ICT) character. This ICT character, however, is not enough to generate separated charges. Aggregation is the key to exciton dissociation in neat NFAs, which we observe with TA of solutions and films of several different NFAs. To explore the impact of an electric field on exciton dissociation in neat NFA devices, we used bias-dependent external quantum efficiency (EQE) and transient absorption (TA) spectroscopy. Electromodulated differential absorption (EDA) measurements then allowed us to observe charge transport under bias. Excitation correlation spectroscopy and fluence-dependent TA finally revealed how non-linear effects can increase the charge yield. Lastly, we comment on whether the neat domain charge generation significantly affects the photophysics of blends or not.
2.3-I2
Understanding and minimizing non-radiative recombination pathways is key to enhanced efficiencies in emerging solar cells, such as perovskite solar cells (PSC) and organic photovoltaics (OPV). Non-radiative recombination in any form, i.e. trap-assisted, or surface recombination of minority carriers at the (wrong) electrode will inevitably lead to lower efficiencies [1]. However, given the fast development of the efficiencies, the stability of OPVs is still not satisfactory.
In this talk, I will focus on the charge transport layers (CTL) and their properties in increasing efficiency and stability. I will focus on the passivation of metal-oxides in nip-solar cells for OPVs. The criterion that the CTL is conducting [2] will simultaneously lead to loss of selectivity [3]. We have identified and mitigated an important loss-factor caused by ZnO in OPVs. Surface defects in ZnO cause n-doping in a thin layer close to the cathode, causing a loss of Jsc. By inserting a thin layer of SiOxNy from solution processing, the recombination zone will be removed, with increased efficiency and lifetime as a result [4].
1.2-I1
Chalcogenides with stoichiometry ABCh3 (Ch = S, Se) form in competing, complex crystal structures and are challenging to synthesize. These aspects may be obstacles for solar cell applications, but are opportunities for fundamental materials science because the distinct structures have distinct (and possibly useful) properties, and because the kinetic barriers to synthesis may enable long-lived metastable phases. The most widely-studied chalcogenide perovskite BaZrS3, with band gap 1.9 eV, sits near the edge of phase stability. Alloying to reduce the band gap usually results in destabilizing the corner-sharing perovskite structure in favor of lower-dimensional structures with highly-anisotropic optoelectronic properties. Epitaxial film growth presents opportunities to stabilize structures that are thermodynamically unstable, and to explore the limits of phase stability and properties. I will present how we use methods of molecular beam epitaxy (MBE) to select between competing phases of BaZrSe3: the perovskite with band gap near 1.5 eV, and a face-sharing hexagonal structure (h-BaZrSe3) with large infrared birefringence. We can direct film growth towards one phase or the other by varying temperature, selenium potential, and/or the growth substrate. By choosing a substrate with rectangular symmetry in-plane, we demonstrate first-of-a-kind growth of fully-oriented h-BaZrSe3 thin films with giant birefringence that can be measured directly polarized transmission spectrophotometry.
Chalcogenide perovskites are also distinguished by their vibrational properties. Their polar optical phonon frequencies are low, and the ionic contribution to their dielectric polarizability is high - they are among the most polarizable of all dielectric semiconductors with comparable band gap. I will present measurements of dielectric response and Hall mobility that demonstrate effective screening of charge defects. However, the phonon frequencies are not so low as for the halide perovskites. I will present Raman and photoluminescence spectroscopy measurements that suggest how and why phonon-assisted, non-radiative recombination (i.e., Shockley-Read-Hall) is much faster in chalcogenide perovskites than in their halide counterparts.
The relative dearth of chalcogenides that are stable in the perovskite structure points to a basic underlying fact, that the perovskite structure is most often found in ionic compounds (e.g., oxides and halides), whereas transition metal chalcogenides have a strong component of covalent bonding. Chalcogenide perovskites can be thought of as covalent semiconductors trapped in ionic structures. I will present explorations of the consequences of this tension, including X-ray spectroscopic measurements of directional bonding, and scanning transmission electron microscopy (STEM) data that suggest substantial band gap fluctuations throughout thin film samples.
I will end by highlighting exciting directions for future research on complex chalcogenide semiconductors, including synthesis science, defect control, and advanced spectroscopy, that may enable future applications in photovoltaics and infrared photonics.
1.2-O1
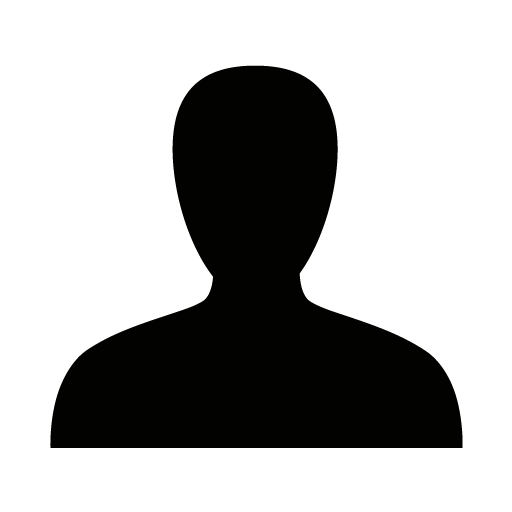
Chalcohalide are a family of inorganic semiconductors material that, thanks to a band gap generally between 0.7 eV and 2.2 eV, are emerging for photovoltaic application.[1–4] Chalcohalides have a the general formula MChX, in which M is one or more metal cations, Ch is a chalcogen (S2-, Se2-, Te2-) and X is an halide (Cl-, Br-, I-).[5] In this class of materials, the mixed metal chalcohalide Sn2SbS2I3 has shown promising potential for photovoltaic application due to its suitable band-gap and stability. Initial studies on its photovoltaic performance were conducted by Nie et al., who fabricated a device based on this material, achieving a power conversion efficiency (PCE) of 4.04%.[6,7] This material is generally synthesized through different methods including solid state synthesis, microwave-assisted processes, solvothermal method, and heat-up procedure. However, these methods leads to a poor control over size and morphology of the final products. Here, we report the synthesis of this mixed-metal chalcohalide Sn2SbS2I3 by hot injection method. Using this procedure for the chalcohalide, and exploiting the possibility to vary the parameter involved in the reaction, i.g. ligand-precursor chemistry, concentration variation of the reactive species, injection temparutre and annealing time,[8] we have achieved an accurate control of the size and the shape of the final crystals through. The particles showed a Cmcm crystal structure and rod-shaped morphology characterized by a lenght and width of 9,9 ± 3,0 µm and 0,9 ± 0,3 µm, respectively. The band gap is around 1.70 eV confirming the promising application for solar cell technologies. These results highlight the hot-injection approach as a promising synthetic method to synthesize Sn2SbS2I3.
1.2-I2
Colloidal metal halide perovskite nanocrystals have emerged as promising candidates for next-generation optoelectronic applications, including solar cells, light-emitting diodes (LEDs), and photodetectors. Their tunable optical and electronic properties, combined with facile solution-based synthesis, have brought lead and tin halide perovskites to the forefront of material research. However, due to environmental and health concerns associated with lead, tin-based perovskites have gained increasing attention, despite challenges posed by the instability of tin in its 2+ oxidation state.
In this work, we report recent advancements in the synthesis of tin halide perovskite nanocrystals, including CsSnI₃, CsSnBr₃, and FASnI₃, through precise control of precursor ratios and ligand chemistry. These developments enable the formation of highly stable, monodisperse nanocrystals with tunable optoelectronic properties. Notably, we observed the simultaneous formation of 2D Ruddlesden-Popper (RP) phases and 3D perovskite structures under similar synthetic conditions. Our studies highlight how the molecular ink plays a pivotal role in directing the growth and stability of these nanostructures.
We further demonstrate that by understanding the reaction mechanism, the precursor intermediate states, the selective formation of either 2D or 3D perovskite nanocrystals can be achieved, depending on the desired application. This control opens pathways for the development of tailored perovskite inks for optoelectronic devices, bridging the gap between fundamental colloidal chemistry and practical device implementation. Our findings provide critical insights into the growth mechanisms of tin halide perovskite nanocrystals, creating novel possibilities for their integration into future high-performance optoelectronic technologies.
1.3-I1
Bismuth-based perovskite-inspired materials (Bi-PIMs) have garnered significant attention as promising alternatives to lead (Pb)-based perovskites due to their lower toxicity and higher environmental stability. These semiconductors, which include compounds like Cu2AgBiI6 and A3Bi2I9, exhibit intriguing optoelectronic properties that make them suitable for photovoltaic applications. Bismuth (Bi)3+ shares similar electronic configurations with Pb2+, hinting to replicate the defect-tolerant electronic structure of lead-halide perovskites. This defect tolerance is crucial for reducing nonradiative recombination losses in Bi-based devices. Nevertheless, Bi-PIMs are also known to crystallize in disordered structures with a large number of surface defects/vacancies and grain boundaries compared to Pb-based perovskites, which explains their modest performance as photovoltaic absorbers. [1] This raises important questions: How can we mitigate the density and detrimental effect of defects in Bi-PIMs? Could the defect-driven structural characteristics of Bi-PIMs be utilized to expand the applications of these materials in photonics beyond photovoltaics?
In the first part of the talk, I will first summarize our key findings on selected Bi-PIMs for photovoltaic applications. In particular, I will present recent results on our compositional engineering efforts to improve morphology and charge carrier transport in Bi-Pims, leading to enhanced efficiency in both outdoor and indoor PV. [2,3]
In the second part of the talk, I will demonstrate second harmonic generation (SHG) for two low-toxicity Bi-PIMs, Cu2AgBiI6 (CABI) and AgBiI4 (ABI), using non-invasive nonlinear optical microscopy. The Bi-PIM with the largest number of cation vacancies, CABI, exhibits the most pronounced local inversion symmetry breaking, as assessed by the determination of the octahedral distortion angles. Consequently, CABI produces stronger SHG signals compared to ABI.
Our findings not only advance our understanding of Bi-PIMs but also open new avenues for their application in photonics.
1.3-I2
Lorenzo obtained his PhD in Chemistry in 2003 and since 2008 is Assistant Professor at the Chemistry Department of the University of Pavia. In 2021 he was appointed Full Professor in the same department. He was the recipient of the Young Scientist Award for outstanding work in the field of perovskites at the International Conference on Perovskites held in late 2005 in Zürich, of the “Alfredo di Braccio” Prize for Chemistry 2008 of Accademia Nazionale dei Lincei awarded to distinguished under 35-year-old chemists and contributed the Journal Materials Chemistry and Chemical Communications“Emerging Investigator” issues in 2010 and 2011. He is working in several areas of solid state chemistry with particular interest in the investigation of structure–properties correlation in different kinds of functional materials, in particular electrolyte materials for clean energy, hybrid organic-inorganic perovskites and catalysis materials. He is author of more than 200 papers on international peer-reviewed journals. Since 2018 he is member of Academic Senate and Vice-Director of the Chemistry Department. He is Director of the INSTM Reference Center “PREMIO” devoted to the synthesis of innovative materials and member of the Directive Board of INSTM. Since 2014 he is member of the Academic Board of the PhD in Chemistry of Pavia University. He is Editor of Journal of Physics and Chemistry of Solids.
The expansion of the discovered material space of MHPs has recently moved towards the investigation of lead-free systems in order to overcome the concerns related to Pb-toxicity. While effective alternatives for PV applications rely mostly on tin-based compositions, several other phases containing different metals such as Bi, Sb, Cu, and Ge have been discovered and investigated.In many cases, such perovskites result ill-suited for PV devices but possess very appealing optoelectronic properties, which can be exploited in other applications. Among these systems, bismuth- and antimony-based perovskite derivatives of general formula Cs3M2X9 (M = Bi, Sb; X = Br, I) have shown strong technological potential, in particular in the area of photocatalysis (both for solar fuel generation and organic synthesis) and photodetection. In this contribution we will present our recent results in the synthesis of powdered, nanocrystalline and thin film based on Bi and Sb metal halides employing sustainable and scalable approaches. The prepared phases, where properties modulation has been achieved by alloying Bi/Sb, the halide site, as well as the A-site cation, were employied in different applicative fields ranging from detection, photocatalysis and non-linear optics [1-3].
1.3-O1
Dr Vanira Trifiletti earned a degree in Material Science from the University of Milano Bicocca (2011). She worked as a research assistant until 2013, when she started the PhD. It was developed at IIT Research Centre for Bio-Molecular Nanotechnology and CNR-Nanotec, under the University of Salento's patronage. Then research fellow at the University of Milano Bicocca at the Materials Science Department until 2019. Until February 2021, she was a Marie Skłodowska Curie Action fellow at the School of Engineering and Materials Science, Queen Mary University of London. She is currently employed as a research assistant at the Materials Science Department of the University of Milano Bicocca. Scientific interests include nanostructured-materials for renewable energy applications, hybrid photovoltaics and thermoelectrics, device design and development, sol-gel synthesis, and chemical-physical analysis.
The introduction of nanoscale materials has enabled substantial technological advancements, with halide perovskites emerging as a promising class of materials due to their ability to self-assemble into structures, promoting charge confinement. These materials are particularly attractive because of their straightforward synthesis and low-cost production. Depending on the degree of isolation between metal halides achieved by organic or inorganic cations, halide perovskites can exhibit two-dimensional, one-dimensional, or quasi-zero-dimensional configurations. Among these, quasi-zero-dimensional perovskite derivatives have garnered attention for applications spanning photovoltaics, thermoelectrics, lasers, photodetectors, memristors, capacitors, and light-emitting diodes (LEDs).[1]
This work investigates the impact of sulphur doping on the thermal and electrical properties of bismuth-based perovskite derivatives. Sulphur doping was achieved by introducing bismuth tri-ethylxanthate into the precursor solution, with thin films fabricated using drop-casting or spin-coating techniques. Structural characterisation, employing X-ray diffraction, Raman spectroscopy, and grazing-incidence wide-angle X-ray scattering, confirmed the successful incorporation of sulphur into the crystal structure. Further insights into the material’s composition and morphology were obtained using X-ray photoelectron spectroscopy, CHNS elemental analysis, scanning electron microscopy, and energy-dispersive X-ray spectroscopy. An extensive study of UV-visible spectroscopy, photoluminescence, inverse photoemission spectroscopy, and ultraviolet photoelectron spectroscopy has provided a comprehensive understanding of the energy band gap. Results demonstrated that only the 1% sulphur doping reduced resistivity by two orders of magnitude. Furthermore, thermal voltage measurements revealed values exceeding 40 mV K⁻¹ at room temperature (~300 K) in both doped and undoped bismuth-based perovskite derivatives, highlighting the potential of these materials for thermoelectric applications.[2]
1.3-O2
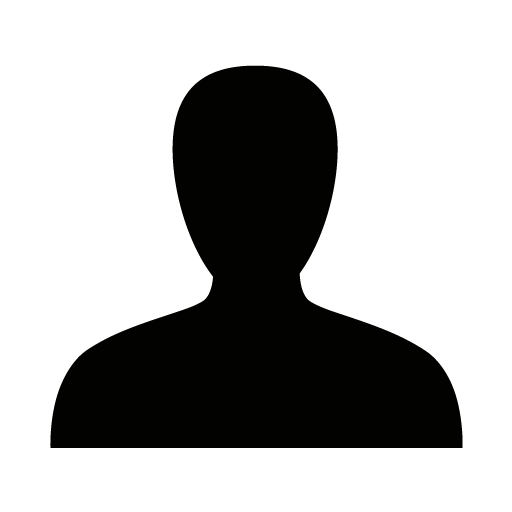
Tin-based perovskites have emerged as a promising semiconductor due to their unique bandgap tunability and potential in all-perovskite multijunction tandems. Despite this potential, they currently lag in terms of stability and efficiency compared to their lead-based counterparts, primarily due to high defect densities from rapid thin-film formation and Sn vacancies caused by oxidation. As Sn oxidation is detrimental to device performance and stability, slower and controlled crystallization to reduce uncoordinated Sn is hypothesized to enhance device performance and stability. Researchers have extensively employed additive, cation, and solvent engineering to control the crystallization of Sn-based perovskites, though understanding of the process remains limited.
We aimed to deepen our understanding of the crystallization of Sn-based perovskites by measuring the photoluminescence (PL) while casting films to quantify the rate of crystallization and additional intermediate phases that may form. Utilizing additive engineering and in situ PL, we demonstrate we are able to significantly decrease crystallization rate relative to FASnI3 and PEA0.15FA0.85SnI3. Additionally, we are able to preferentially orient FASnI3 along the [001] and [010] planes, indicative of more controlled crystal growth. Additionally, we are able to grow FASnI3 thin films completely at room temperature, minimizing the amount of added energy into the system to oxidize Sn(II). Upon exposure to ambient conditions, x-ray photoelectron spectroscopy (XPS) measurements have shown increased stability of the engineered FASnI3 with significantly less Sn(IV) content compared to pure FASnI3 and 10.48% power conversion efficiency when implemented into a p-i-n solar device.
2.1-I1
Pablo P. Boix, Ph.D. in Nanoscience, is a Research Scientist at Instituto de Tecnologia Química (CSIC). He led a pioneer perovskite research team at Nanyang Technological University (NTU), Singapore (2012-2016) with relevant contributions to materials and devices’ development (such as the first use of formamidinium cation in perovskite solar cells). His track record has more than 100 publications, which resulted in his selection as a Highly Cited Researcher in 2020 (Cross-Field) by Clarivate Web of Science, with an h index of 57. Dr. Boix is the co-inventor of 3 patents in the field of perovskite optoelectronics. Prior to his current position, he worked as a research group leader in a perovskite solar cell company (Dyesol Ltd, Switzerland), focusing on product R&D, and at Universitat de València. Currently, he is the PI of 2 research projects and the coPI of 3, including regional, national, and European funding.
Despite the potential of tin-based perovskites (Sn-PVKs), these materials face challenges such as defect formation and Sn²⁺ oxidation or non-uniform film formation, which limit their performance and stability when applied to optoelectronic devices. The integration of crystallization control and dimensionality modulation emerge as essential strategies to enhance the stability and performance in both material and devices. Here we present recent advancements in addressing Sn-PVKs’ challenges through these strategies, including the use of thiophene-2-ethylammonium halides (TEAX, where X = I, Br, Cl). The control on the synthetic conditions enables the dimensionality modulation of Sn-PVK microcrystals. In particular, using TEA+ as an organic cation, the formation of 2D-TEA₂SnBr₄ and highly luminescent 0D-TEA₄SnBr₆ microstructures was achieved, showcasing tailored optical properties for optoelectronic applications [1]. In parallel, the use of TEAX as an additive enhances FASnI₃ crystallization, suppresses Sn²⁺ oxidation, and boosts solar cell power conversion efficiency (PCE) from 6.6% to 12%. These additives also improve operational stability, with solar cells retaining over 95% of their initial PCE after 2000 hours of continuous operation under simulated sunlight [2]. The mechanisms of the unique FASnI₃-TEAX solar cells enhanced stability under ambient conditions will be highlighted. This includes insights into environmental factors such as humidity, applied voltage, and illumination, which influence the reversible decay and recovery of device performance. Our analysis provides a comprehensive approach to unlocking the full potential of Sn-based perovskites in next-generation photovoltaics.
2.1-O1
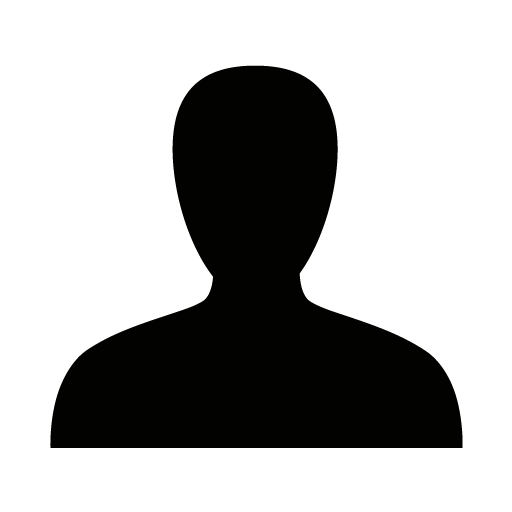
Tin-based perovskites (Sn-PVK) have emerged as one of the most promising lead-free alternatives for the development of efficient and environmentally friendly photovoltaic technologies. Despite their potential, the performance of Sn-PVK solar cells is severely limited by a high density of bulk and surface defects. These defects are primarily attributed to the rapid crystallization of the perovskite and the tendency of Sn²⁺ to oxidize into Sn⁴⁺, which creates lattice vacancies and significantly degrades device performance. The introduction of thiophene-2-ethylammonium halides (TEAX, where X = I, Br, and Cl) has been demonstrated as a strategy to improve the crystallization of FASnI₃, a common tin-based perovskite. When used in solar cells, these films present an improved operational stability maintaining >95% of the initial power conversion efficiency (PCE) after >2000 h in N2 in continuous operation under 1 sun illumination. However, the evolution of the FASnI3-TEAI solar cell parameters changes when carried out under ambient conditions.
In this work we present ongoing research to explore the mechanisms underlying the decay and subsequent recovery of the performance of FASnI₃-TEAI solar cells under ambient conditions. By analyzing the influence of environmental factors such as humidity, applied voltage, and illumination on device behavior, we aim to provide a better understanding of this unique phenomenon.
2.1-O2
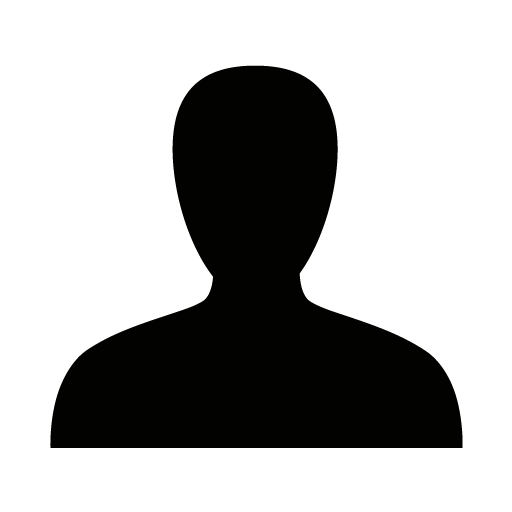
Tin halide perovskite solar cells (Sn-PSCs) are emerging as strong candidates to replace Pb-based perovskite solar cells (Pb-PSCs) due to their excellent optoelectronic properties and reduced toxicity. However, Sn-PSCs exhibit significantly lower efficiencies in comparison to Pb-PSCs due to, among other issues, the high voltage losses, which are nearly double the observed values in Pb-based PSCs.
On this basis, we designed and synthesized two novel fullerene derivatives, namely C60-1 and C60-2, functionalized with different fluorinated moieties, and incorporated them as interlayers between the perovskite and the C60 electron transport layer. The LUMO levels of C60-1 and C60-2 at -3.98 eV and -4.01 eV, respectively, exhibited better band alignment with the conduction band of the tin perovskite layer (-3.92 eV) compared to C60 (-4.05 eV). This enhanced alignment minimized the energy level mismatch, significantly improving the overall device performance. Additionally, the fluorinated functionalization conferred an extra degree of hydrophobicity enhancing the operational stability of the devices without any encapsulation in ambient atmosphere conditions. Consequently, the efficiency of the devices increased from 9.3% for the reference device to 10.5% and 11.0% for the devices containing the C60-1 and C60-2 interlayers, respectively. These results highlight the potential of functionalized fullerenes to mitigate voltage losses and improve the performance and stability of Sn-PSCs, paving the way for future advancements in their design and development.
2.1-I2
Pnictogen-based semiconductors have gained increasing attention as potential nontoxic alternatives to lead-halide perovskites [1]. This is because of their ability to replicate key features of the electronic structure of lead-halide perovskites, which is believed to be conducive towards achieving defect tolerance, whilst overcoming the toxicity and stability limitations. This talk discusses one such material, BiOI, for applications in X-ray detection.
The composition of heavy elements Bi and I leads to stronger X-ray attenuation than commercial Cd-Zn-Te and amorphous-Se, as well as lead-halide perovskites. We show that the high stopping power, as well as its large mobility lifetime products and low dark currents enable these devices to have a limit of detection >250x improved over commercial amorphous-Se and Cd-Zn-Te detectors. This can improve the safety of medical imaging, and we examine the fundamental reasons behind the properties of BiOI enabling this strong performance [2]. Finally, we discuss the key challenges to bring this technology to market [3].
2.2-O1
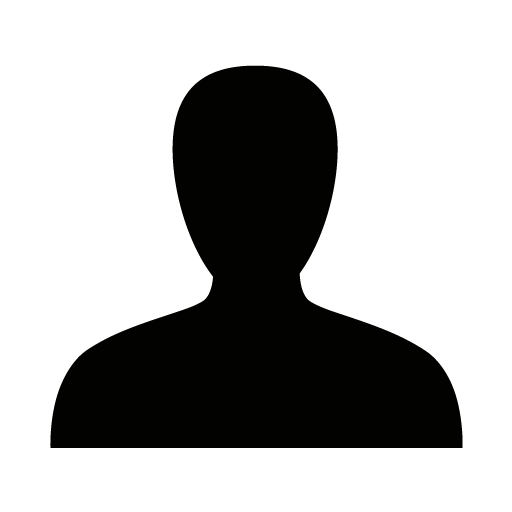
Luminescent zero-dimensional (0D) antimony halide (Sb–X) hybrids showcase emissive properties (emission peak position; photoluminescence quantum yield (PLQY)) that are heavily influenced by the local metal halide geometry/site asymmetry. However, achieving control over this local geometry has proven synthetically challenging due to the variety of coordination geometries that the Sb–X units can adopt. As a result, establishing a clear structure–luminescence relationship in 0D Sb–X hybrids has been difficult. This study is an attempt to draw a structure–luminescence relationship by controlling the Sb–X geometry utilizing 2D cadmium halide hybrids as the host that serves as a framework for incorporating emissive Sb3+ dopants. By selecting different organic cations, the local metal halide geometry/distortion within the host hybrids can be tuned, which in turn modulates the luminescent properties of the Sb3+ dopants. A distinct structure–luminescence relationship is observed: as the local metal halide distortion increases, the emission peaks shift to longer wavelengths (red-shift), and the PLQY improves. DFT calculations of the doped compounds, which explore the structural and electronic properties in both the ground and excited states, help clarify the luminescence mechanism and the reasons for varying luminescence efficiency (PLQY). This study provides deeper insight into the luminescence mechanisms, emphasizing the significance of structural distortions in both the ground and excited states of Sb3+-doped 2D cadmium halide hybrids. The experimental and computational findings are valuable for developing a clearer structure–luminescence relationship and for guiding the rational synthesis of 0D Sb halide hybrids.
2.2-I1
Dr. Galian received her Ph.D in Chemistry at the National University of Cordoba, Argentina in 2001. Then, she was a postdoc researcher at the Polythecnic University of Valencia, University of Valencia and University of Ottawa. During those years, she has studied photosensibilization processes by aromatic ketones using laser flash photolysis techniques and was involved in photonic crystal fiber/semiconductor nanocrystal interaction projects. In 2007, Dr. Galian came back to Spain with a Ramon y Cajal contract to study the surface chemistry of quantum dots and since 2017 she has a permanent position as Scientist Researcher at the University of Valencia. Her main interest is the design, synthesis and characterization of photoactive nanoparticles and multifunctional nanosystems for sensing, electroluminescent applications and photocatalysis.
Perovskite nanocrystals (NCs) are well known for their exceptional tuneable optical and electronic properties. They can be engineered at the nanoscale to optimize their interaction with light, improving the efficiency of the absorption, transfer, and conversion of energy.
Lead-free metal halide materials, such as Bi- and Sb-based NCs, having an A3B2X9 (A = CH3NH3+, Cs+; B = Sb3+ or Bi3+, X = Cl–, Br–, I–) stoichiometry, have been proposed as good candidates for heterogeneous photocatalysis thanks to their higher chemical stability and better performance than those of Pb-based perovskite NCs. In this context, the ability of colloidal layered structures Cs3Sb2Br9 NCs for the photoreduction of p-substituted benzyl bromide substrate to produce C-C coupling products and the key role of the surface chemistry in facilitating the interaction between the NC and the substrate will be discussed. Moreover, they showed promising activity towards the photocatalytic degradation of organic dyes with good recyclability, preserving their performance and structural properties.
Recently, we have explored the (photo)catalytic potential of a family of perovskite oxides CaxSr01-xTiO3 (x=0-1) doped with iron and cobalt the sublattices. The pollutant degradation (organic dyes) and clorates reduction activity will be also discussed for water remediation.
2.2-O2
Double perovskite (DP) nanocrystals (NCs) are promising lead-free materials for applications in photovoltaics, light-emitting devices, and plastic scintillators. The state-of-the-art DP NCs, Cs1.92Ag0.2Na0.75K0.07InCl6 doped with 0.5% Bi (CANKBIC), achieve a photoluminescence (PL) quantum yield (QY) of ~70% [1]. However, their excited-state fine structure remains unexplored. In this work, we present temperature- and magnetic field-dependent PL dynamics of CANKBIC NCs, providing evidence for a luminescent state split into three distinct levels with lifetimes ranging from a few ns to ms.
Synthesized CANKBIC NCs exhibit PL centered at 2.0 eV and a multiexponential decay with an average PL lifetime (τavg) of 4.7 µs at room temperature. The PL originates from self-trapped excitons involving BiCl6 and AgCl6 octahedra, though the fine structure of the emissive state remains unexplored. As the temperature drops below 150 K, the τavg triples—a phenomenon previously attributed to the suppression of non-radiative decay, suggesting near-unity QY below this temperature [2]. At temperatures below 15 K, the τavg further increases reaching values of ~ms at 6 K. We interpret these results as a fingerprint of a fine structure in the emissive state, with a lower lying forbidden (dark) and a higher lying allowed (bright) state. Crucially, the analysis of the temperature dependent PL lifetimes and the decay amplitudes, reveals that the dark state is further split into two states exhibiting very different transition probabilities. At 6 K, applying a magnetic field shortens the PL lifetime, consistent with field-induced mixing between the dark states. We attribute the fine structure to a combined effect of electron-hole exchange interaction and spin-oribit coupling in a low symmetry environment, similar to what is observed in e.g. Cu(I) molecular complexes [3].
This study unveils the previously unobserved excited-state fine structure of DP NCs, contributing new insights into their photophysical behavior.
2.2-I2
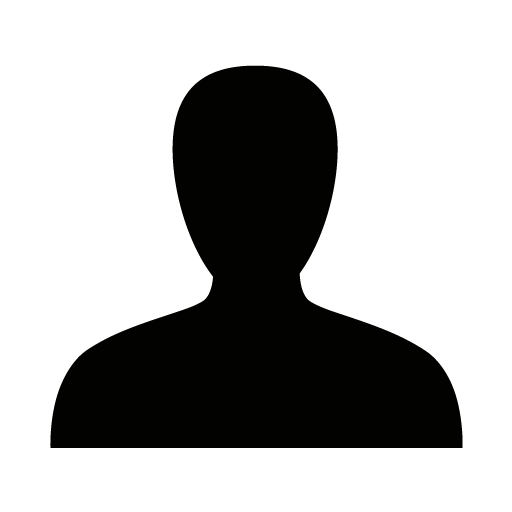
As a new class of semiconducting materials with excellent optoelectronic properties, metal halide perovskites have been applied in various fields such as solar cells, photodetectors, and electroluminescence. However, the toxicity of the lead element contained in these materials limits their application scenarios. Tin, a low-toxic element in the same main group as lead, is considered the most suitable alternative to lead. However, the performance of tin perovskite materials is currently far inferior to lead-containing materials, especially in terms of the short carrier lives and low open-circuit voltages of photovoltaic devices. Current research community generally attribute these phenomena to the material defects of tin perovskites, but the nature and regulation of these defects remain obscure. Under the guidance of theory, we have systematically studied this issue for tin perovskites from both aspects of bulk and surfaces, and prepared a series of high-quality single crystal and thin film samples. We directly characterized the types of defects, their concentrations and influence on semiconductor properties, revealing that the dominant defects in tin perovskites are not directly caused by oxidation of Sn2+. We found that substituted thiourea molecules as Lewis-base ligands can deactivate the Sn2+ 5s electron pair and create an appropriate intermediate phase structure, thereby slowing down the crystallization rate, inhibiting surface and bulk defects of the crystal, and obtaining high-quality thin films. On the other hand, by decorating the thin-film surfaces with molecular dipoles but not changing the bandgap of perovskite layer, we elevated its conduction band minimum (CBM) to better match the energy level of the electron transport material (ETM). The above measures have rendered thin films of tin perovskite with charge carrier lifetimes longer than 0.5 μs and diffusion lengths on the micrometer scale, and boosted the open-circuit voltage of solar cell devices to 1.0 V (only ~0.1 V loss), approaching the level of lead-containing materials. The energy conversion efficiency reached 16%, setting a new performance record for lead-free perovskite solar cells. Their stabilities are also significantly enhanced, demonstrating a promising application prospect.
2.2-O3
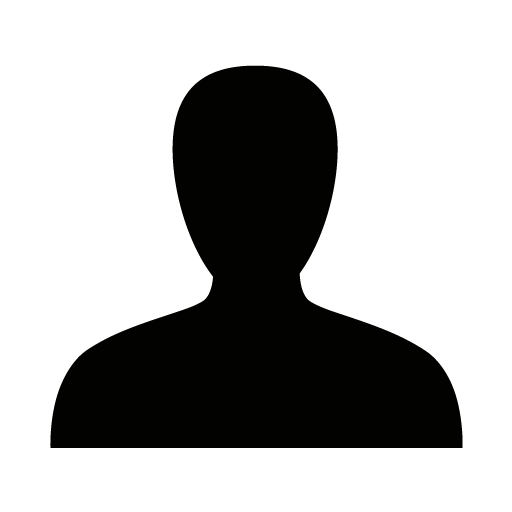
In recent years, substantial efforts have been made, dedicated to identifying lead-free perovskites that retain the remarkable optoelectronic properties of lead-based perovskites while enhancing stability and reducing toxicity. Numerous substitution strategies have been explored, resulting in promising new materials, like for example halide double salts. This class of materials, have demonstrated high potential, with compounds like AgBiI4 and Ag3BiI6 showing some of the highest power conversion efficiencies among lead-free photovoltaic materials[1–4]. Yet, these materials have been a challenge to model due to the existence of vacancies, and partially occupied Ag- and Bi-sites in their crystal lattice. Recently, we developed a symmetry-based approach to create atomistic models that allow the accurately description of their electronic structure and optical properties[5]. Here, we employ this approach to investigate atomic substitutional engineering in order to explore the phase space of Ag-In halide double salts and design a direct band-gap material in analogy with the case of Cs2AgInCl6 double perovskite[6]. By substituting Bi3+ with In3+ in a reduced-symmetry model of AgBiI4, our first-principles calculations for AgInI4 show a direct bandgap within the visible range (1.72 eV), close to the optimal range for indoor photovoltaic applications. This value is comparable with the indirect bandgap (1.68 eV) of the Bi-based ternary compound, suggesting a potential advantage for the In-based material in photovoltaic efficiency under specific conditions. To confirm this, we evaluate the spectroscopic maximum limited efficiency of AgInI4 compound under AM-1.5G solar irradiance and LED irradiance using the LED-B4 standard. Our findings indicate that the In-based compound under-perform with respect to Bi-based for thin-film thickness of around 500 nm, though slightly improved performance is observed at thicknesses of above 1.5 μm. Based on these predictions, we set to synthesize the hypothetical In-based double salt and the resulting thin film exhibited transparency at room temperature, not matching our initial hypothesis. This prompt us to explore the possibility that more stable phases exist within the complete Ag-In-I phase space. By employing a systematic materials screening process within the Materials Project database, [7] we identify new phases that are more stable than the AgInI4 phase designed starting from AgBiI4. These phases exhibit direct bandgaps that are larger than 3.0 eV. While these hitherto unknown phases may not be suitable for photovoltaic applications, their transparency, dispersive electronic bands and well-defined band edges position them as promising candidates for other applications that require wide-bandgap semiconductors. Overall, our exploration of the Ag-In-I phase space broadens the opportunities for the development of environmentally friendly materials for optoelectronic applications where wide bandgap semiconductors are required.
1.1-I1
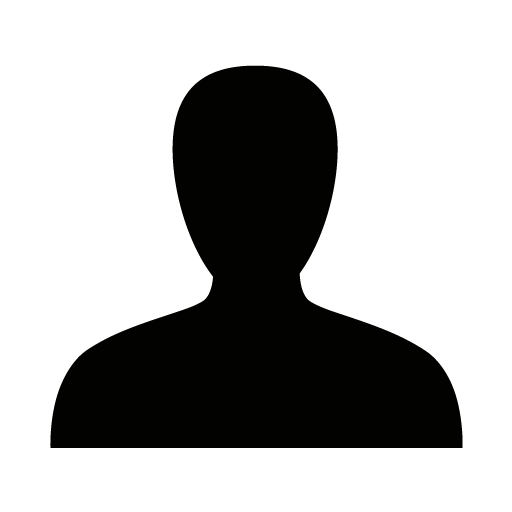
Large scale light source facilities such as synchrotrons and X-ray free electron lasers (XFELs) are used across the breadth of scientific applications from helping to develop new energy storage materials to understanding viruses and aiding the development of vaccines. The demands of making faster measurements with high fluxes of X-rays has driven the development of new readout electronics and supported the adoption of direct detection materials. Driven by the upgrade to “3rd generation” synchrotrons, Si based photon counting detectors have been used on synchrotrons since the mid-2000s [1] with high-Z semiconductor options, such as CdTe, following over the next decade. In parallel, similar technologies were in development for medical-CT, but the certification requirements and barriers to entry in the medial field meant it was not until the 2020s that the first photon-counting detector CT systems reached the clinic [2].
Whereas the requirement of medical CT detectors to measure hard X-rays up to a flux of 109 photon/mm2/s at a resolution of 0.3-0.5mm is unlike to change, the synchrotron detector technology is being pushed further. Spatial resolutions less than 0.1mm are already commonplace and the advent of 4th generation synchrotrons is pushing the flux requirements up to 1012 photons/mm2/s. In parallel, XFELs which deliver very intense femto-second X-ray pulse are moving to continuous repetition rates in the MHz range which will require X-ray detectors to operate at similarly high fluxes and small pixel sizes.
Currently, the best candidate detector material for these applications is CdZnTe. It has the benefit of high X-ray stopping power, low leakage current, good electron charge transport properties, is available with fine pixelation and is compatible with some standard interconnection techniques [3]. However, CdZnTe is melt grown in 3-inch boules that limit the single die size and has limited hole transport properties that may ultimately limit the operating flux. The rapid development of Perovskite and similar detector materials have demonstrated the potential of these materials as hard X-ray detectors [4]. The challenges and opportunities that these new materials may bring to the scientific X-ray detector community will be discussed.
Whilst the ~70 light source facilities around the world may not represent the largest volume detector market, they have the benefit of being research facilities that can support the development and advancement of new detectors materials and readout technology to push the boundaries of X-ray detection.
1.1-O1
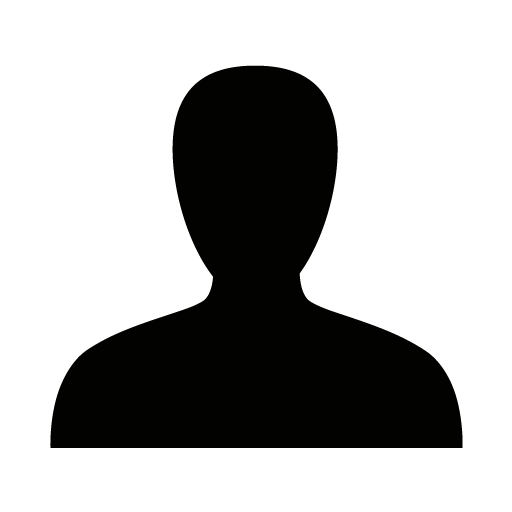
Currently, there is a significant need to increase the spatial resolution of medical X-ray imaging while maintaining high detection efficiency to provide high-quality images at low radiation doses [1]. This pushes research for the development of novel detector materials and architectures. Besides diverse state-of-the-art detector materials such as Si a-Se, and CdTe, recently, a novel class of semiconductors - metal halide perovskites - emerged as promising materials for radiography and computed tomography applications. However, existing studies lack persuasive examples of fully integrated perovskite detectors that would combine a high spatial resolution of multi-pixel detectors together with high detection efficiency (DE) [2], for which direct integration of single crystals on the readout substrates would be required. Here we show a method of fabricating high-quality, thick large-grain polycrystalline CsPbBr3 films by melt growth directly on pixelated glass interposers. The obtained detector arrays show a remarkable 20 lp mm-1 spatial resolution with a DE of 75.4% and low noise equivalent dose (NED) of ca. 46 photons for 22 keV X-rays under a low reverse bias voltage. The combination of these characteristics results in unprecedently, for charge-integrating mode, high values of 20% for detective quantum efficiency (DQE) at the Nyquist frequency. Single-pixel devices demonstrate single-photon counting performance for γ-radiation with an energy-resolved peak of 241Am. Melt-grown CsPbBr3 films, featuring a unique combination of detection efficiency, scalable fabrication, and cost-effectiveness, are promising for the development of high spatial resolution, low-dose X-ray imaging systems.
1.1-O2
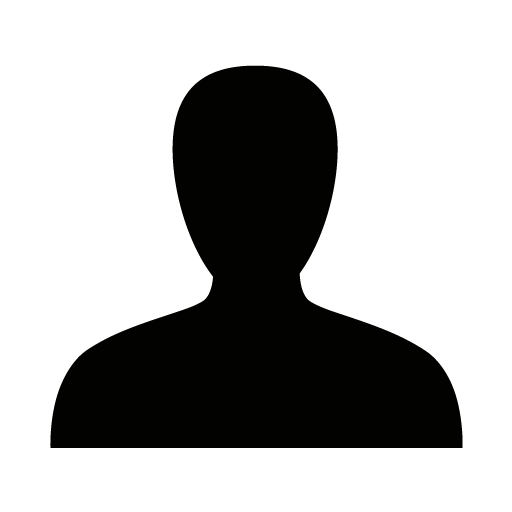
Hybrid metal halide perovskites have emerged as promising materials for X-ray detection due to their scalable, cost-effective, and robust solution growth, combined with their ability to detect single gamma-photons under high applied bias voltages. Despite these advantages, their rapid degradation under high electric fields, a result of mixed electronic-ionic conduction, has hindered the development of stable and efficient perovskite-based X-ray detectors.
To address this limitation, we previously demonstrated a photovoltaic mode of operation at zero-voltage bias, using thick methylammonium lead iodide (MAPbI3) single-crystal films (up to 300 µm) grown directly on hole-transporting electrodes via the space-confined inverse temperature crystallization (ITC) method [1]. These devices exhibited near-to-ideal performance, long-term stability, 88% detection efficiency, and a noise equivalent dose of 90 pGyair with 18 keV X-rays. However, we observed experimentally that the performance of MAPbI3 devices degrades significantly beyond thicknesses of 200 µm, presenting a challenge for their application in scenarios requiring thicker crystals for higher sensitivity.
Building upon this foundation, we now present [2] advances in compositional engineering that enable up to 100% charge extraction in perovskite single crystals with thicknesses reaching 900 µm. These high-quality crystals exhibit extended charge carrier lifetimes, enabling efficient absorption of X-rays at energies of both 18 keV and 45 keV and complete charge collection. This marks a significant step forward in perovskite detector technology, as the thicker crystals allow for higher detection sensitivity while maintaining excellent material stability. Uniquely, these devices operate with no external bias, a breakthrough in X-ray detection technology. Unlike other semiconductor direct X-ray detectors, which require extremely high electric fields generated by hundreds of volts to achieve efficient charge collection, our perovskite detectors achieve 100% charge extraction under zero-voltage bias. This sets a new standard for X-ray detectors, combining superior performance with simplicity in operation.
In conclusion, this study introduces a novel approach to perovskite X-ray detectors by combining compositional engineering with advanced solution growth techniques. This results in devices with enhanced charge extraction efficiency, superior carrier lifetimes, and robust long-term stability, paving the way for cost-effective, high-performance X-ray imaging technologies.
[1] Sakhatskyi, K.† Turedi, B.†, Bakr, O. M, Kovalenko, M. V. et al. Nature Photonics 2023, 17(6), 510-517.
†equal first-authors
[2] unpublished
1.1-I2
From 2019, A.M. serves as Associate Professor in Condensed Matter Physics at Department of Materials Science. His research is focused on the development of advanced hybrid functional nanomaterials for applications in photonics and theranostics in collaboration with several national and international universities and research institutes. He started his research by working on hybrid organic/inorganic light NIR emitters based on lanthanides ions and photonic crystals for lighting and telecom, in the framework of several national and international project and networks. The topic of the current research is the design and study of advanced materials and nanostructured materials for photon managing and scintillation applications. The experimental activity is centered on CW and ultrafast TRPL photoluminescence spectroscopy, transient absorption spectroscopy, confocal imaging, IR and FT-IR spectroscopy to tackle both fundamental and applicative aspects aimed at the development of materials to implemented real-world technologies.
Fast and highly emissive plastic scintillators, usually made of a polymeric scintillating matrix that host a fluorescent dye, are requested for many advanced applications where high signal-to-noise ratio is required in a short time window. For example, to detect high rate events avoiding pile up in high energy physics experiments at the energy and intensity frontiers to face the challenges of unprecedented event rate and severe radiation environment, or to quickly acquire high quality image at low dose in medical applications as in the time-of-flight positron emission tomography (TOF-PET) imaging technique, where coincidence time resolution (CTR) of tents of picoseconds time is desired. Unfortunately, their low density results into a low stopping power of the high energy radiation and their scintillation light yield 𝜙𝐿𝑌, defined as the ratio between the number of emitted photons and the energy deposited in the system, is lower than the one of best inorganic scintillators. This detrimentally reduces the emitted light output and consequently the detector sensitivity.
A common strategy to improve the 𝜙𝐿𝑌 is the loading of polymeric scintillators with high Z elements or dense nanoparticles (NPs) to enhance the stopping power of liquid and polymeric conjugated scintillators [1-3]. Otherwise, to still keep the good emissive properties of conjugated systems, a possible solution is to use scintillating metal-Organic Framework (MOF) crystalline s hybrid system composed of conjugated ligands connected by heavy-element containing linking nodes [4-7].
I will present the most recent results on the development of these system highlighting the main peculiar photophysical of the scintillation process in these materials and the perspectives for their future uses.
1.2-I1
Proton therapy is a cutting-edge cancer treatment that uses high-energy proton beams to target tumors with remarkable precision. Unlike conventional radiation therapy based on high energy photons, proton therapy allows for a more localized deposition of energy, delivering a high dose to the tumor while minimizing damage to surrounding healthy tissues. This precision is especially crucial in treating cancers located near critical organs or in pediatric patients, where sparing healthy tissue is essential to reducing long-term side effects.
The success of proton therapy relies heavily on accurate dose delivery. Even small deviations in the proton beam’s range or energy due to misalignment issues can lead to under-dosing the tumor or over-dosing healthy tissues. Therefore, during proton therapy is even more important the monitoring in real-time of the dose delivered to the patients to ensure treatment safety and effectiveness. In fact, real-time and in-situ dose monitoring systems can detect discrepancies during treatment, enabling immediate corrections and improving patient outcomes.
Organic semiconductors and lead halide perovskites recently demonstrated their potentiality for the detection and spatial mapping of MeV protons1–4. These two classes of materials in the form of thin films fully benefit from solution-based fabrication processes that allow fast and easy large-area coverage over flexible thin plastic substrates with low-cost procedures.
Here, we report about the employment of organic and perovskite thin films as the active layer of proton direct and indirect detectors for the real-time and in-situ monitoring of the dose delivered to the patients during proton therapy treatments.
1. Fratelli, I. et al. Real-Time Radiation Beam Monitoring by Flexible Perovskite Thin Film Arrays. Advanced Science (2024).
2. Fratelli, I. et al. Direct detection of 5-MeV protons by flexible organic thin-film devices. Sci Adv 7, eabf4462 (2021).
3. Basiricò, L. et al. Mixed 3D – 2D Perovskite Flexible Films for the Direct Detection of 5 MeV Protons. Advanced Science 2204815, 1–8 (2022).
4. Calvi, S. et al. Flexible fully organic indirect detector for megaelectronvolts proton beams. npj Flexible Electronics 1–11 (2023).
1.2-O1
Recently, near-infrared (NIR) photodetectors are gaining increasing attention due to the significant development of automotive vehicles, smart phones, machine vision, augmented reality, and so on. Particularly, some high-throughput applications such as optical communication and computed axial tomography also require NIR photodetectors to response fast. In the wavelength region between 850 and 1100 nm, where LiDAR (light detection and ranging) technology is operated, silicon photodetectors dominate the current market owing to their low cost and easy integration with fabrication procedures in industry. However, silicon photodetectors are usually bulky and require high-temperature purification processes, making them less attractive for wearable devices. In this respect, emerging absorbers including organic materials, lead-halide perovskites, and chalcogenide quantum dots are perfect alternatives to silicon because they can be synthesized using low-temperature solution-processing methods. Nevertheless, these emerging absorbers are also facing different challenges. For instance, organic materials have poor carrier transport, and lead-halide perovskites are not stable in air. Moreover, almost all the efficient NIR photodetectors based on lead-halide perovskites and chalcogenide quantum dots contain Pb or Cd, which raises great concerns on toxicity as well as environmental pollutions. It is also worth noting that only a handful of NIR photodetectors based on organic materials or PbS have reported a cut-off frequency exceeding 300 kHz, indicating that a fast photo-response is still challenging to achieve in these solution-processed photodetectors, especially in the NIR region.
In this work, we aim to develop fast NIR photodetector using a perovskite-inspired material – AgBiS2, which has an ideal bandgap (~1.2 eV) along with strong absorption coefficients (> 105 cm-1 in the visible and UV region), and is only composed of RoHS-compliant (low toxicity) elements. We found that AgBiS2 photodetectors could exhibit high cut-off frequencies either under white light (> 1 MHz) and NIR light (approaching 500 kHz) illumination, which correspond to rise/fall times of only a few microseconds. Such fast photo-response is attributed to the short transit distances of photo-excited charge-carriers in the ultrathin AgBiS2 layer, where drift transport could dominate. Temperature-dependent transient current measurements also revealed ion migration to play a crucial role in limiting the photo-response speed and increasing the dark currents of AgBiS2 photodetectors, while its detrimental impacts can be effectively suppressed by carefully tuning the thickness of the AgBiS2 layer. These outstanding characteristics enable an air-stable, real-time, and solution-processable heartbeat sensor to be realized based on our AgBiS2 NIR photodetector, demonstrating the great potential of AgBiS2 devices in high-throughput systems.
1.2-O2
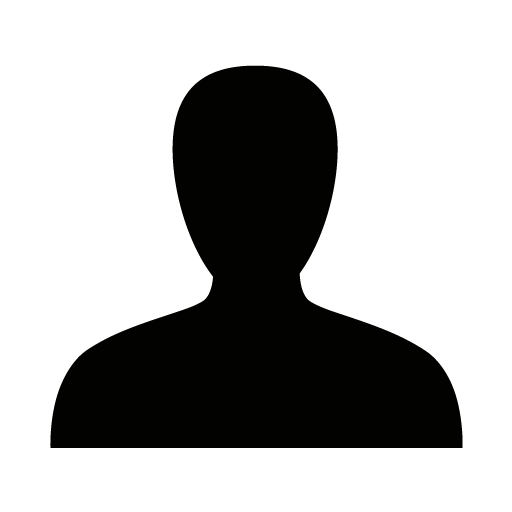
Title: Project MULANS: Multi-layered Nano Scintillators
Author(s), Abigail Seddon, Guillaume Bertrand, Ludovic Tortech
Affiliation, Commissariat à l’énergie atomique et aux énergies alternatives, Saclay, France
E-mail: abigail.seddon@cea.fr
Scintillators are materials that emit light when bombarded with high-energy particles (β, γ) or X-rays; they convert incoming X-ray radiation into visible light that can then be captured using film or photosensors. For each application wihtin radiodetection, there is an appropriate organic or inorganic scintillator exhibiting the requirements of the desired measurements. However, for all scintillators two phenomena are an ongoing challenge to master. (1) The scintillation light emission is isotropic, hindering efficient light collection. (2) Ultra-fast decay cannot be achieved without a drastic loss of light production. Herein, a multi-layered scintillation device is reported, exhibiting high light collection and ultra-fast decay (low afterglow) achievable via the Purcell effect. The Purcell effect is the enhancement of a quantum system's spontaneous emission rate by its environment.2 By using a substrate of either a layer of metal or alternating layers of metal and dielectric material, it was found that the light conversion efficiency can be increased by 250 percent for a scintillation device (Figure 1) compared to a conventional homogeneous scintillator.1
A proof-of-concept of a novel bilayer Purcell device is reported, consisting of an organic scintillation molecule, such as POPOP or 1,9-diphenylanthracene, with an inorganic dielectric such as CuBr. The devices are fully characterised strcuturally, including by SEM-EDX, XPS, and AFM. The difference in optical properties between each layer enables the angular enhancement observed by photophysics and radiphysics. Moving forwards, devices may provide better light yield and reduced decay times, inhibiting afterglow with adequate spectral shaping via the Purcell effect. Real-world results include enabling faster and better resolution scans. This is the first report of a device of this kind.
1.2-O3
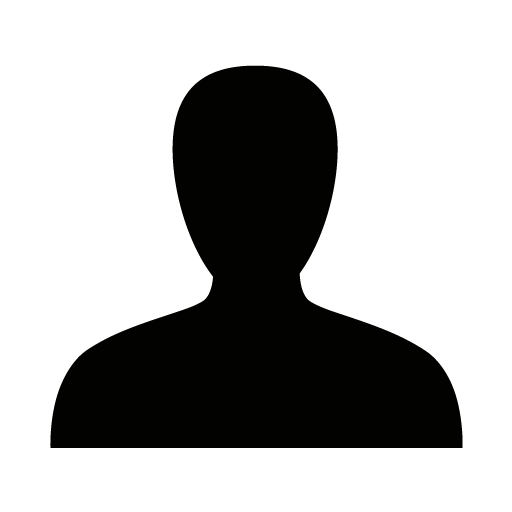
A multilayer luminescent design concept is presented to develop energy sensitive radiation-beam monitors on the basis of colorimetric analysis. Each luminescent layer within the stack consists of rare-earth-doped transparent oxide of optical quality and a characteristic luminescent emission under excitation with electron or ion radiation. For a given type of particle beam (electron, protons, α particles, etc.), the penetration depth and the energy loss at a particular buried layer within the multilayer stack vary with the initial energy of the beam. The intensity of the luminescent response of each layer depends on the energy deposited by the radiation beam within the layer, so characteristic colour emission will be achieved if different phosphors are included in the layers of the luminescent stack. Phosphor doping, emission efficiency, layer thickness, and multilayer design are key parameters relevant to achieving a broad colorimetric response. Case examples are designed and fabricated to illustrate the capabilities of these new type of detector to evaluate the kinetic energy of either e- beams of a few keV or α particles of a few MeVs.
1.2-I2
Chemically synthesised semiconductor nanocrystals are gaining momentum as ultrafast nanoscintillators for radiation detection. In particular, quantum-confined lead halide perovsite (LHP) nanocrystals in polymeric matrices combine the high density of Pb-based scintillators with high production scalability, extreme radiation hardness, and unique scintillation properties governed by a multiexciton generation and decay process. This results in competitive efficiencies with Pb-enriched commercial plastic scintillators, improved stability to radiation damage and ultrafast emission lifetimes, largely below 0.5 ns, which promise important advances in fast timing applications from medical tomography to high energy physics. In this talk, I will review the fundamental mechanisms of nanoscale scintillators, highlighting the impact of multi-exciton generation processes and showing how carrier dynamics optical experiments can effectively anticipate particle size effects on both scintillation timing and efficiency. The potential of single nanocrystals is further extended by demonstrating collective scintillation processes made possible by the unique electronic structure of LHP nanocrystals. In addition to isolated nanocrystals, innovative ways to improve performance in high-density plastic matrices and supermolecular nanocrystal architectures will be presented and discussed.
1.3-I1
Silvia Colella is a researcher at the National research council, CNR-NANOTEC, in Bari, Italy. She received her PhD in “Nanoscience” at National Nanotechnology Laboratory in Lecce (Italy), in 2010. She has been visiting student in the group of professor Luisa De Cola at the Westfälische Wilhelms-Universität of Münster (Germany), where she dealt with the synthesis and photophysical characterization of electroluminescent metal complexes. In 2010 she joined BASF – The Chemical Company (Strasbourg) with a Marie Curie fellowship as experienced researcher in the frame of the EU project ITN SUPERIOR, working on Dye Sensitized Solar Cells. She continued as post-doc researcher at the Institut de science et ingénierie supramoléculaires (ISIS) in Strasbourg, France. In 2012 she started her independent research in Lecce (Italy) at the University of Salento in collaboration with CNR-NANOTEC, the team focused on the conception and optoelectronic characterization of innovative optoelectronic devices based on hybrid halide perovskites. Many high impact publication were produced in this time interval, among them one of the first report in halide perovskite for PV exploitation (Colella et al, Chemistry of Materials, 2013 25, 4613-4618).
Silvia Colella is author of >70 peer-reviewed publications in renowned international journals (including Energy and Environmental Science, Advanced Materials, ACS Energy Letters).
Her scientific production led to >3000 total citations and a h-index of 28 (https://scholar.google.it/citations?user=S2TZd_4AAAAJ&hl=it; https://www.scopus.com/authid/detail.uri?authorId=24170650100).
Lead-Halide hybrid Perovskites are recently emerging as promising materials for high energy radiation detection thanks to the combination of high absorption coefficient, excellent transport properties, even in polycrystalline films, and their solution processability. Here we present Perovskite based X-ray detectors employing a perovskite-polymer composite thin film as active layer. The use of polymeric template for the perovskite growth allows a precise control of the active layer thickness and compactness, and confers to the device unconventional resistance to moisture and performances.[1] The performances of the active perovskite layer, inserted in a photodiode architecture, were studied under 40kV X-ray radiation. Remarkably, a top sensitivity of 5.5±0.2 μC Gy-1 cm-2 was measured for the device operating in passive mode (0V), coupled with exceptional stability. The samples were stored in air for 629 days tracking the performance degradation retaining 97% of the initial sensitivity. [2] A general overview of the potential of polymeric perovskite-based composites for x-ray detection in given.[3,4]
References
[1] A Giuri, S Masi, A Listorti, G Gigli, S Colella, CE Corcione, A Rizzo, 'Polymeric rheology modifier allows single-step coating of perovskite ink for highly efficient and stable solar cells', 2018, Nano Energy 54, 400-408
[2] M. Verdi, A. Giuri, A. Ciavatti, A. Rizzo, L. Basiricò, S. Colella, B. Fraboni, 'Record stability for perovskite-based X-ray detectors through the use of polymeric template', under submission, 2023, Advanced Materials Interfaces 10 (18), 2300044.
[3] Incorporation of Functional Polymers into Metal Halide Perovskites thin-films: from interactions in solution to crystallization, 2023 Materials Advances.
[4] Implication of polymeric template agent on the formation process of hybrid halide perovskite films, Antonella Giuri, Rahim Munir, Andrea Listorti, Carola Esposito Corcione, Giuseppe Gigli, Aurora Rizzo, Aram Amassian, Silvia Colella, 2021, Nanotechnology.
1.3-O1
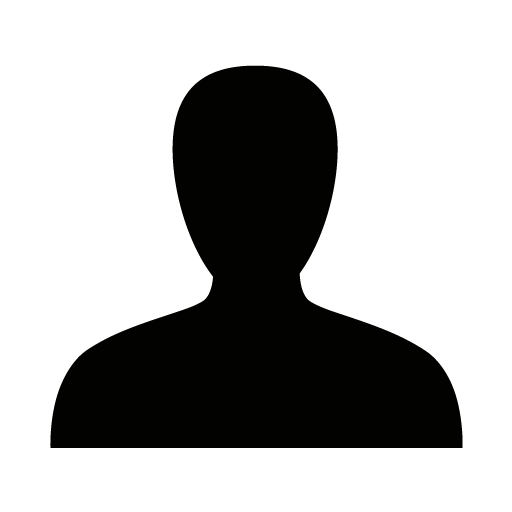
Metal halide perovskites (MHPs) are emerging as promising materials for next-generation radiation detectors due to their high atomic number, efficient X-ray absorption, low exciton binding energy, and unique electronic structure, which enhances charge carrier mobility and collection efficiency [1-3]. Furthermore, their solution processability and mechanical flexibility make them ideal for integration into lightweight, flexible X-ray detector designs. In this work, we report the fabrication and characterization of lightweight perovskite-embedded membranes (PEMs) by spin-coating triple-cation perovskite onto hydrophilic membranes. The detectors were tested under continuous X-ray irradiation using a laboratory X-ray source and pulsed X-ray conditions at the KMC-3 XPP beamline of BESSY II, enabling comprehensive performance evaluation in diverse radiation environments. Pulsed X-ray testing was conducted with energies ranging from 7 keV to 16 keV and at different voltages.
With this approach, a sensitivity of 0.97 × 10⁵ μC/Gyair.cm² under continuous 8 keV irradiation at a dose rate of 0.094 µGyair/s at 100 V was achieved. Additionally, we investigated the impact of polymer treatment on the X-ray detection performance. By adding polymers, we further increased the sensitivity to 2.38 × 10⁵ μC/Gyair.cm² for the polymer-treated devices under the same conditions.
Our results show that polymer treatment improves the photoluminescence quantum yield (PLQY) and increases the crystallinity of the perovskite at the 100 planes, indicating enhanced material quality. These improvements correlate with a notable increase in X-ray sensitivity in the polymer-treated devices.
Notably, the detectors demonstrated X-ray response even at 0 V, with polymer-treated device showing a sensitivity of 0.93 × 10² μC/Gyair.cm², while the control devices showed a sensitivity of 0.42 × 10² μC/Gyair.cm² under continuous 8 keV irradiation at a dose rate of 184.7 µGyair/s. Under both continuous and pulsed X-ray conditions, polymer-treated devices consistently outperformed untreated controls, underscoring their superior performance and robustness.
The polymer additives act as passivation agents, reducing defect states and improving charge transport properties, which contribute to the enhanced X-ray detection performance. These findings position polymer treatment as a key strategy for optimizing the performance of flexible perovskite X-ray detectors.
In conclusion, this study demonstrates the feasibility of developing high-performance, flexible perovskite X-ray detectors with enhanced sensitivity. These advancements open the path towards lightweight, portable, and wearable radiation monitoring systems, creating new opportunities for advanced radiation detection.
1.2-I1
The convergence of population growth and climate change threatens food security on a worldwide scale. Current trends in population growth suggest that global food production is unlikely to satisfy future demand as predicted. In order to accomplish these significant objectives, the use of agrochemicals (fertilizers, pesticides, plant hormones, etc.) becomes inevitable to ensure quality and high yields. However, their intensive application has resulted in the deterioration of ecosystems. making necessary to develop more efficient and less toxic methods against pests and infections, while improving crops productivity. Importantly, large quantities of agrochemicals do not reach their intended objectives due to application practices (between 10-75% do not reach their target) and their widespread use, contributing to the deterioration of ecosystem quality, adversely affecting the health of living beings, water and soil.
Among the novel technologies considered, Metal-Organic Frameworks (MOFs) appeared as innovative and promising materials for environmental applications.[1] In this work, we take a further step and use organic agrochemicals as linkers in the preparation of MOFs, what we have called AgroMOFs. Through this original strategy, we aim to achieve a controlled agrochemical delivery and enhance crop production and quality, while reducing contamination.[2] All these studies evidenced the potential of MOFs in agriculture without damaging our environment.
1.2-I2
Overcoming biological barriers is essential for nanomedicines to reach their intended targets, requiring the exploration of "smarter" delivery nanosystems that can discern when and where to release specific compounds, thereby avoiding off-target effects. However, translating these concepts into clinical practice demands a deeper understanding of how synthetic nanomaterials interact with the complex biological environment.
Inspired by the natural interactions of cells, viruses, and exosomes within the body, biomimetic nanocarriers replicate the structure and functionality of cellular membranes. These carriers, designed using cellular components such as those from cancer cells, platelets, and macrophages, exhibit enhanced pharmacokinetics and tissue-specific targeting capabilities (Adv. Biosys, 2020, 4 (3), 1900260; J. Nanobiotechnol. 2022, 20, 538; J .Nanobiotechnol., 2024, 22 (1), 10).). Such nanocarriers can be programmed for a variety of specialized functions, including immune evasion, homotypic targeting, and direct cytosolic delivery via membrane fusion, bypassing traditional endo-lysosomal pathways. (J. Colloid Interface Sci. 2023, 648, 488-496; J. Colloid Interface Sci, 2024). The combination of engineered biomimetic coatings with nanosized metal-organic frameworks (nanoMOFs) represents a novel approach that integrates the homotypic targeting and fusogenic capabilities of cell-derived membranes with the high payload capacity of MOFs. This innovative system demonstrates efficient cellular internalization of therapeutic agents, reduced toxicity, and enhanced cytotoxic effects in both 2D cultures and 3D spheroid models. These findings highlight the system’s potential for precise drug delivery in cancer therapy and suggest opportunities to adapt this approach for various tumor types and therapeutic agents, advancing the field of precision nanomedicine.
1.2-O1
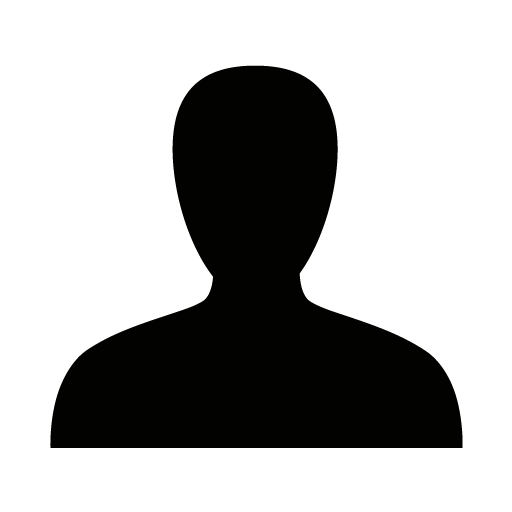
When cells internalized nanoparticles via endocytosis this involves also proteins bound to the surface of the nanoparticles. After internalization, the original protein corona may be partly exchanged. In case the proteins bear labels providing contrast for imaging, the in vivo distribution of the originally bound proteins as well as the one of the nanoparticles can be determined. This can be done for example with fluorescence or X-ray fluorescence based method. Colocalization analysis then provides information about the degree in which the original protein corona is retained.
Most studies about the interaction of nanoparticles (NPs) with cells are focused on how the physicochemical properties of NPs will influence their uptake by cells. However, much less is known about their potential excretion from cells. In order to control and manipulate the number of NPs in a cell however both, cellular uptake and excretion need to be studied quantitatively. Monitoring the intracellular and extracellular amount of NPs over time (after residual non-internalized NPs have been removed), enables to disentangle the influence of cell proliferation and exocytosis, which are the major pathways for the reduction of NPs per cell. Proliferation depends on the type of cells, and exocytosis depends in addition to the type of cells also on the properties of the NPs, such as their size. Examples are given on the role of these two different processes for different cells and NPs.
1.2-O2
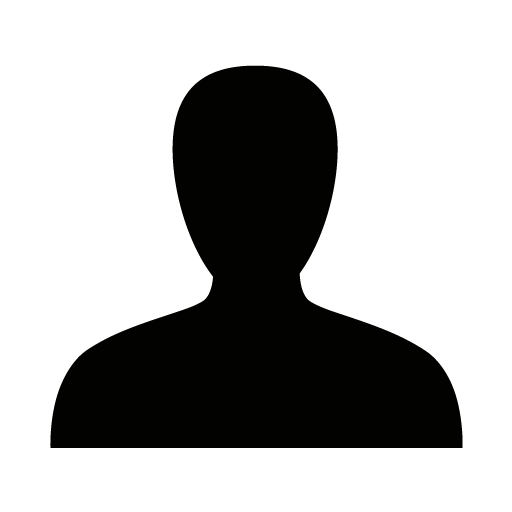
We present a green and high-yield method for the synthesis of core-shell nanocomposites (NCs), where magnetic nanoparticles (MNPs) are coated with UiO-66 or UiO-66-NH₂ metal-organic framework (MOF) shells, using Zr or Hf clusters. Notably, the presented method avoids the use of hazardous dimethylformamide (DMF), relying on ethanol as a solvent, making it highly suitable for biomedical applications, such as targeted drug delivery or hyperthermia. The process allows for the control of the shell thickness from 5 to tens of nm and thus to further adapt the resulting composites for the specific applications. The shell thickness is shown to directly affect the heating capabilities of the NC. The MOF shell serves as an effective insulating layer which can confine the produced heat to close vicinity of the NC. As a proof of concept and to demonstrate the versatility of our approach, we also coated Au and Pd NPs of various shapes and sizes. In summary, this work provides a facile, efficient, and reproducible methodology for the preparation of NCs with a controlled size while opening new avenues in the field of MNP@MOF nanocomposites.
1.3-I1
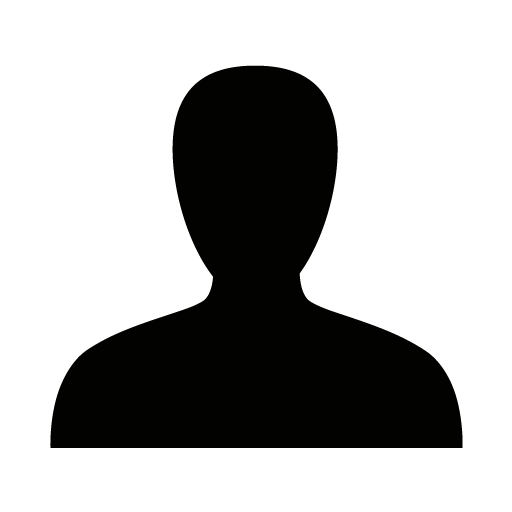
The high demand of food of the ever increasing global population involves the extensive use of agrochemicals to increase crop production. However, this goal also poses real threats to human health (110,000 deaths/year and 5 million pesticide related illnesses), aquatic ecosystems, and the environment at large. One of the most common classes of pesticides, organophosphates (OPs), are highly toxic to humans and ecosystems as a consequence of their acetylcholinesterase (AChE) inhibitory activity. In this communication, I report the ability of different zinc zeolitic imidazolate frameworks (ZIFs) to behave as potential antidotes against OP poisoning. The Zn–L coordination bond (L = purine, benzimidazole, imidazole, or 2-methylimidazole) is sensitive to the G-type nerve agent model compounds diisopropylfluorophosphate (DIFP) and diisopropylchlorophosphate, leading to P–X (X = F or Cl) bond breakdown into nontoxic diisopropylphosphate. P–X hydrolysis is accompanied by ZIF structural degradation (Zn–imidazolate bond hydrolysis), with the concomitant release of the imidazolate linkers and zinc ions representing up to 95% of ZIF particle dissolution. The delivered imidazolate nucleophilic attack on the OP@AChE adduct gives rise to the recovery of AChE enzymatic activity, thereby reversing organophosphate poisoning[1,2].
1.3-I2
I earned my PhD in Chemistry from the Tokyo University of Agriculture and Technology in 2000, focusing on the activation of carbon-oxygen and carbon-sulfur bonds by low-valent transition metal complexes. Following this, I joined the Université Pierre et Marie Curie as a postdoctoral researcher, where I worked on the design and synthesis of organometallic complexes with non-linear optical (NLO) properties. In 2001, I was honored with a prestigious Humboldt Fellowship, which provided me the opportunity to collaborate with Prof. J. A. Gladysz at the University of Erlangen-Nuremberg in Germany. There, I conducted research in organometallic chemistry and homogeneous catalysis. In 2003, I transitioned to the Institute of Materials Science of Barcelona (ICMAB), where I collaborated with Profs. F. Teixidor and C. Viñas as part of the I3P Postdoctoral Program (2003-2006) on various projects on the synthesis of functional boron cluster based molecular materials. This was followed by a Ramón y Cajal Fellowship (2007-2008), culminating in my appointment as a tenured researcher in 2008.
Since 2024, I have co-led the Inorganic Materials and Catalysis Laboratory (LMI) at ICMAB, where I coordinate research activities centered on boron-cluster-based molecular and polymeric materials, including metal-organic frameworks (MOFs), and their diverse applications. My current work focuses on exploring the limits of boron clusters based polymeric materials in the fields of optics, magnetism, (electro/photo)catalysis and biomedicine, thus bridging various research areas, including chemistry, physics, material science and biomedicine.
Carboranes, polyhedral boron clusters, have emerged as a fascinating class of 3D ligands for the construction of metal-organic frameworks (MOFs) due to their unique properties, such as thermal stability, chemical inertness, and tunable electronic and steric characteristics. Carborane-based MOFs have gained significant attention in recent years as promising materials for various applications [1]. The versatility of carboranes allows for the design and synthesis of MOFs with diverse structures and properties, offering exciting opportunities for fundamental research and practical applications.
We are currently exploring the integration of lanthanide and/or transition metal ions with carborane ligands to create a range of innovative multifunctional materials. Our recent work demonstrated that the bulkiness and acidity of carborane linkers enables the synthesis of multivariate MOFs incorporating flexible combinations of multiple lanthanides. This strategy has produced Tb/Eu MOFs for anticounterfeiting [2], GdLn MOFs with magnetocaloric and luminescent properties [3], and the first-ever MOF containing eight different lanthanides [4]. Leveraging the multivariate approach, we are currently synthesizing carborane-based MOFs with strategically selected lanthanide combinations and exploring our approach to transition metals. This strategy allows us to explore the properties of "complex multifunctional materials" and develop novel materials with tailored functionalities.
1.3-O1
When cells internalized nanoparticles via endocytosis this involves also proteins bound to the surface of the nanoparticles. After internalization, the original protein corona may be partly exchanged. In case the proteins bear labels providing contrast for imaging, the in vivo distribution of the originally bound proteins as well as the one of the nanoparticles can be determined. This can be done for example with fluorescence or X-ray fluorescence based method. Colocalization analysis then provides information about the degree in which the original protein corona is retained.
2.1-I1
2.1-I2
Manuel Souto Salom (Valencia, 1988) is an Oportunius Research Professor and Principal Investigator at CIQUS (University of Santiago de Compostela). He is also a Guest/Visiting Professor at the University of Aveiro. He holds a double degree in Chemistry and Chemical Engineering from the University of Valencia (Spain) and from the École de Chimie, Polymères et Matériaux (ECPM) de Strasbourg (France), respectively, doing a research internship at PLAPIQUI (Argentina). He also earned a Master’s degree in Molecular and Supramolecular Chemistry (2011) from the University of Strasbourg conducting his Master thesis at Instituto Superior Técnico (IST, Lisbon). He obtained his PhD in Materials Science at Institut de Ciència de Materials de Barcelona (ICMAB-CSIC) with Prof. Jaume Veciana in 2016 conducting two research stays at the National University of Singapore (NUS) and at the University of Antwerp. In 2017, he started to work as a postdoctoral researcher at the Institute of Molecular Science (ICMol-UV) with a Juan de la Cierva fellowship. In 2019, he started his independent research career as an Assistant Professor at the Chemistry Department of the University of Aveiro and CICECO-Aveiro Institute of Materials. In 2022 he was promoted to Principal Researcher (tenure, Permanent Researcher/Assoc. Prof.) at the same institution. His research interests encompass molecular electronics, electroactive polymers and organic batteries. His main current research interest is the design and synthesis of new functional electroactive porous frameworks (e.g., COFs & MOFs) based on redox-active organic building blocks for energy storage applications. In 2021, he was awarded an ERC Starting Grant with the project ELECTROCOFS, which aims to design new redox-active COF-based electrodes for rechargeable batteries. He received, among other distinctions, the NanoMatMol PhD award, the PhD Extraordinary award, and the European Award on Molecular Magnetism Doctoral Thesis. He is member of the RSEQ (GENAM) and SPQ chemical societies and Fellow of the Young Academy of Europe.
Improving the efficiency of the renewable energy conversion and storage devices is one of the main challenges in order to reduce the energy consumption and mitigate climate
change. Redox-active metal-organic (MOFs) and covalent organic frameworks (COFs) have emerged in recent years as auspicious electrode materials towards energy storage applications due to their high stability, porosity to facilitate ion diffusion, and huge chemical and structural versatility [1,2] Besides their inherent porosity, MOFs and COFs may also incorporate electronic functionalities, such as electrical conductivity, becoming attractive for their implementation as integral components in electronic devices [3]. Such porous materials present other advantages, including the easy modulation of physical properties by post-synthetic modifications, and the fine-tuning of their electronic properties is easily accomplished. In this sense, the design of mixed ionic-electronic conductors is highly desirable for energy storage applications. In the first part of the talk, I will present a proton-electron dual-conductive MOF based on tetrathiafulvalene(TTF)-phosphonate linkers and lanthanum ions. The formation of regular, partially oxidized TTF stacks with short S···S interactions facilitate electron transport via a hopping mechanism. Additionally, the material exhibits a proton conductivity of 4.9 x 10-5 S cm–1 at 95% relative humidity conditions due to the presence of free -POH groups, enabling efficient proton transport pathways [under review]. In the second part of the talk, I will present an approach to improve the electrochemical performance of an anthraquinone-based COF (DAAQ-TFP-COF) cathode material in metal anode (Li, Mg) based batteries [4]. Finally, the synthesis and electrochemical properties of a series of redox-active TTF-based COFs that were explored as high-voltage organic cathodes for lithium batteries will be discussed [5]
2.1-O1
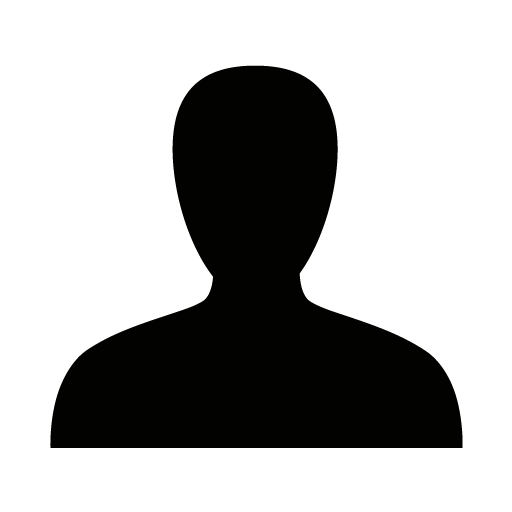
Zeolitic imidazolate frameworks (ZIFs) which are a subtype of metal organic frameworks (MOFs) have been extensively used to prepare catalyst materials for a variety of electrochemical reactions for energy conversion and storage applications. Most notable examples of ZIFs used for that purpose include ZIF-8 and ZIF-67 etc. Particularly ZIF-8 with its high surface area, defined pore structure and tunable particle size is widely utilized as a platform material to prepare so-called metal- and nitrogen-doped carbon (M-N-C) catalysts with M= Co, Fe, Ni, Zn etc., which are an emerging class of catalyst materials [1], [2]. The active sites in M-N-Cs ideally have M-N4 coordination resembling to metal centres in macromolecules such as porphyrins and phthalocyanines [3]. Most representative examples of M-N-Cs include Fe-N-Cs, Co-N-Cs and Ni-N-Cs etc. which are showing promising activities for a variety of electrochemical reactions e.g. oxygen reduction reaction (ORR), carbon dioxide reduction reaction (CO2RR) and hydrogen evolution reaction (HER). The structures of M-N-C catalysts are quite complex and require a fine balance between morphological, electronic, and chemical properties to reach optimal electrocatalytic activities. In this talk, I will present our activities on (i) the preparation of phase-pure M-N-C catalysts derived from ZIF-8 via active-site imprinting [4],[5] and highlight the benefits of our strategy to achieve high density of active sites and enhanced electrochemical performance levels [6] and (ii) feasibility of using gas physisorption techniques as a new approach to quantify active sites in M-N-Cs. The challenges of maximizing active site utilization and eliminating unfavourable mass-transport characteristics faced by ZIF-8 derived M-N-Cs in electrochemical energy devices e.g. fuel cells will also be briefly discussed.
2.1-O2
I was born in Kottayam, Kerala (India) in 1997. I completed my BSMS dual degree from the Indian Institute of Science Education and Research (IISER), Tirupati with a major in Chemistry. I was awarded INSPIRE fellowship for the entire program. During my master thesis, I worked on “Tuning of upconversion luminescence in lanthanide-based upconversion nanophosphors” and "Mandelic acid appended chiral gels as efficient templates for multicolour circularly polarized luminescence" under the supervision of Dr. Jatish Kumar (IISER Tirupati). Then I moved to the University of Basel for doing PhD in Chemistry. My PhD studies focuses on the synthesis of metal-oxo clusters and their application in catalysis and formation of porous frameworks. My hobbies include traveling and watching movies.
Metal-organic frameworks (MOFs) have been one of the most advancing classes of materials in the past few years, with immense potential in the fields of catalysis,[1] gas storage,[2] drug delivery,[3] water purification,[4] etc. However, the fundamental aspect of determining the precise chemical composition of the synthesised MOFs is lacking sufficient attention, although it is crucial for many advanced applications, such as catalysis.
Here, we developed a simple yet robust methodology to derive the minimal formula of the synthesised MOF material.[5] To achieve this, we combine Nuclear Magnetic Resonance (NMR) spectroscopy, Thermogravimetric analysis (TGA), and UV-Vis spectroscopy. We investigated the previously used methodologies that solely rely on TGA and demonstrated why the assumptions that were made in this technique are not justified. We further dive deep into the use of NMR to quantify the different organic molecules present in the framework. We have also shown the crucial influence of digestion methods and relaxation time on the accurate determination of the minimal formula. Finally, we highlighted the importance of determining the amount of chloride ions in the MOF structure and showcased that chloride is present in significant quantities when a MOF is synthesized from chloride-based precursors such as ZrCl4 of ZrOCl2.
We also introduce the concept of “room temperature molar mass” as this is more significant in terms of applications of MOFs and has arrived at a fully charge-balanced and chemically feasible minimal formula. We used our methodology to derive the room temperature minimal formula of MOF-808 and UiO-66 to show the generality of our technique. This work thus lays the foundation for a more rigorous reporting of MOF compositions.
2.2-I1
Dr. Carolina Carrillo Carrión is a Tenured Scientist of the Spanish National Research Council (CSIC) since 2022, working at the Institute for Chemical Research (IIQ, CSIC-University of Seville). In 2023 she started her own group “NanoChemistry with Metal-Organic Frameworks (NanoChemMOFs)” at the IIQ.
Short CV: degree in Chemistry (2006), M.Sc. in Fine Chemistry (2007) and European PhD degree (2011) in the Analytical Chemistry Department of the University of Córdoba (UCO); responsible of the analytical department and R&D projects at Biomedal company (2012-2013); Humboldt postdoc Fellow (2013-2015) in @Parak Biophotonics group (Philipps-University of Marburg); Juan de la Cierva-Incorporación contract (2015-2017) at @CIC biomaGUNE; Marie Curie postdoc Fellow (2018-2020) at the CiQUS@USC; JIN/RETOS-project as PI at the University of Córdoba (09/2020-03/2021); Ramon y Cajal contract (04/2021-12/2021, University of Seville); Tenured Scientist of CSIC at IIQ (since 2022).
Research interests: Her scientific career has been focused at the interface between (bio)analytical chemistry, nanotechnology and material science, and biomedicine. She has expertise in the design, synthesis, and characterization of functional hybrid nanomaterials for (bio)applications. Since 2018 she focused her research on metal-organic frameworks (MOFs), including two main research lines: i) the study of the unique characteristic of MOFs, not found in other porous materials, such as the structural changes/transformations upon an external stimulus (e.g., light irradiation, heat treatment, high-pressure, gas/water adsorption), including thermal diffusion, gate-opening phenomena, phase-to-phase transitions; and ii) the development of MOF-based nanoplatforms for specific target applications in different fields. In the biomedical field, the most relevant examples focus on designing MOFs for light-triggered intracellular release of bioactive compounds, bioorthogonal catalysis in living cells, renal therapies (MOF-modified kidney scaffolds or enhanced adsorbents for hemodialysis), and Glyco-MOFs for improved therapies. In other research fields, MOF-based systems have been designed for biomass valorisation, sensing, and energy-related applications.
Publications: https://scholar.google.es/citations?hl=es&user=VCVQqlQAAAAJ&view_op=list_works&sortby=pubdate
The application of Metal-organic frameworks (MOFs), especially nanoscale MOFs, in biomedicine has become a rapidly developing hot research topic in the last years. This interest arises from their unique properties, mainly their high chemical and structural diversity, tunability, and potential multifunctionality. Since the structure of the MOF will directly influence its properties, function, and biological performance, each MOF must be designed specifically for each target application. In this direction, the engineering of MOFs involves the incorporation of functional units to endow them with new properties and functions, with the ultimate aim of developing novel MOF-based nanoplatforms with improved performances for some specific applications. There is a wide variety of interesting functional units that can be smartly combined with MOFs, ranging from metallic nanoparticles, to polymers, fluorinated agents or therapeutic biomolecules, and the appropriate synthetic and/or functionalization strategy must be optimized depending on the nature and characteristics of the functional unit and the type of MOFs. To illustrate the potential and bright future of MOFs in the field of biomedicine, this talk will show an overview of different possibilities through a series of concrete examples focused on different application areas, including MOF-nanoplatforms for the generation of drugs inside cells, phototherapy, renal therapy or hemodialysis, and immune-mediated therapies.
2.2-O2
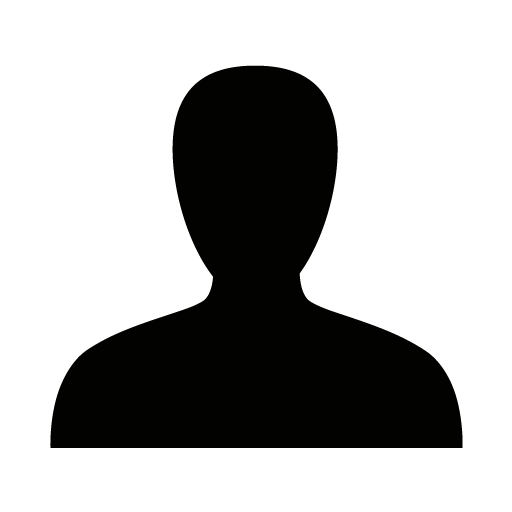
Metal-Organic Frameworks (MOFs) are crystalline, porous materials composed of metal or metal clusters bonded by polytopic organic ligands.1 These materials possess unique physicochemical properties, making them attractive for various applications such as gas storage/separation, catalysis, drug delivery, chemical sensing, and water treatment.2 On the other hand, plasmonic nanoparticles exhibit unique optical properties, including Localized Surface Plasmon Resonance (LSPR), which make them suitable for applications such as catalysis, sensing, and heating.3,4
Combining these two distinct materials presents challenges due to the functionalization of the nanoparticle core, which must fulfill two roles: adapting the nanoparticles to the high-temperature and high-pressure conditions typically required for MOF synthesis, and promoting the controlled growth of MOFs onto the cores.
However, there are limited reports in the literature regarding the growth of Zr-based MOFs, such as the UiO family, on plasmonic nanoparticles. This is primarily due to the propensity of high temperatures and long reaction times to cause reshaping or complete dissolution of the nanoparticles, resulting in either etching or undesirable changes in optical properties.
In this study, we developed synthetic methodologies aimed at preventing reshaping and etching during the synthesis of nanocomposites comprising Zr-based MOFs and Au nanoparticles functionalized with polyethylene glycol. These nanocomposites exhibit absorption around 800 nm and maintain their infrared absorption properties even at lower synthesis temperatures, thereby increasing reaction yields. Additionally, the nanocomposites demonstrate colloidal stability and have been extensively characterized.
Our findings not only provide insights into overcoming challenges associated with MOF-plasmonic nanoparticle composites but also offer a foundation for the development of stable and functional nanomaterials suitable for a wide range of applications.5
2.2-O3
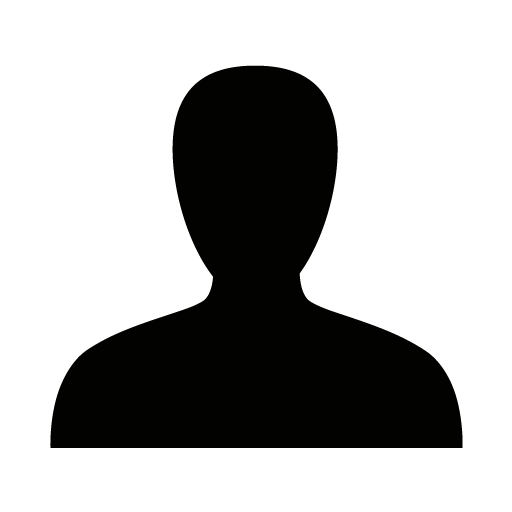
Group IV metal-organic frameworks (MOFs) with phosphonate linkers offer enhanced stability and potential applicability compared to their carboxylate-based counterparts. However, synthetic strategies for accessing such frameworks remain underdeveloped. The inorganic nodes of MOFs - group IV metal oxo clusters, are considered the smallest conceivable nanocrystal prototypes. Their structurally similar inorganic core, capped with an organic ligand shell, allows them to be treated as model systems for colloidally stable nanocrystals of the same composition.[2]
The effectiveness of nanocrystals in many applications depends on their surface chemistry. Here, we leverage the atomically precise nature of zirconium and hafnium oxo clusters to gain fundamental insights into the thermodynamics of ligand binding. Using a combination of theoretical calculations and experimental spectroscopic techniques, we investigate the interactions between the M6O88+ (M = Zr, Hf) cluster surface and various ligands: carboxylates, phosphonates, dialkylphosphinates, and monosubstituted phosphinates. We refute the common assumption that the adsorption energy of an adsorbate is unaffected by the surrounding adsorbates. Through ligand exchange from the carboxylate-capped clusters, we find that dialkylphosphinic acids possess too much sterical hindrance, preventing complete exchange. Monoalkyl or monoaryl phosphinic acids drive off carboxylates quantitatively and we obtained the crystal structure of M6O4(OH)4(O2P(H)Ph)12 (M = Zr, Hf), first fully phosphinate-capped clusters. Phosphonic acids, however, cause a structural reorganization into amorphous metal phosphonate as indicated by Pair Distribution Function analysis.
These findings rationalize the absence of phosphonate-capped M6O8 clusters and underscore the challenges in preparing group IV phosphonate MOFs. Our results further reinforce the notion that monoalkylphosphinates, as carboxylate analogs with superior binding affinities, are promising alternative linkers for MOFs. We infer that while metal oxo clusters serve as minimalistic prototypes for oxide nanocrystals, their surface chemistries exhibit significant diversity due to variations in surface curvature. The development of a versatile toolkit for precise manipulation of cluster surfaces could unlock new opportunities in designing advanced cluster-based materials.
2.2-O1
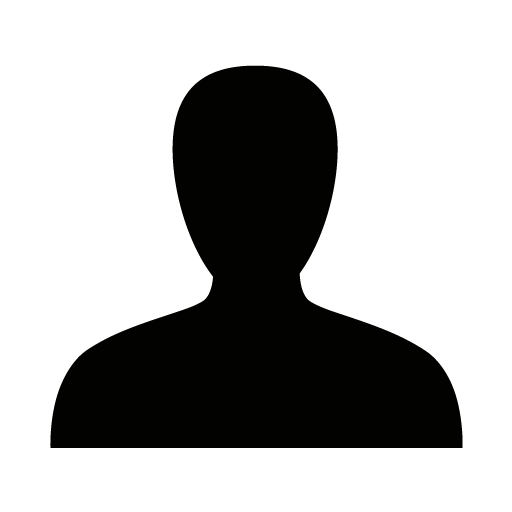
In recent years, metal−organic frameworks (MOFs) have been extensively investigated for diverse heterogeneous catalysis due to their diversity of structures and outstanding physical and chemical properties.[1] Currently, most related work focuses on employing MOFs as porous substrate materials to fabricate confined nanoparticle or heteroatom doped electrocatalysts.[2] However, they must typically be annealed at high temperature before application.
Herein, a simple room-temperature process is used to synthesize a series of bi-, tri-, tetra- or penta-metallic MOFs directly on a nickel or steel substrate. The as-prepared MOFs are applied directly as highly efficient oxygen evolution reaction (OER) electrocatalysts with no post-annealing treatment. Highest OER activity was found for the tetra-metallic Ni2Co2MnZnFex-BTC MOF outperforming those of single metal MOFs and commercial precious RuO2 catalysts significantly. With this MOF as the catalyst, OER current densities of 10 and 100 mA cm-2 can be achieved with overpotentials of only 222 and 250 mV, respectively. Meanwhile, a small Tafel slope of 30 mV dec-1 was obtained.
It was found important that some iron ions from the steel substrate are incorporated in the MOF structure due to local partial corrosion; if a nickel substrate is used some few iron ions must be added to obtain electrocatalytic activity. The presence of BTC linkers is important as well; without them no active material is deposited on the substrates.
Moreover, the catalysts show high electrochemical stability in strong basic solution as used in alkaline electrolysis. This work demonstrates that bi- and multi-metallic MOFs prepared by easy and energy-efficient deposition procedures are promising as advanced catalysts for electrochemical energy conversion.
2.3-I1
Carlos Martí-Gastaldo was initially trained in Coordination Chemistry and Molecular Magnetism in E. Coronado´s group at the ICMol-University of Valencia (PhD 2009), before shifting focus to apply his training to the design of Metal-Organic Frameworks during my postdoctoral stage as a Marie Curie Fellow in M. J. Rosseinsky's group at the University of Liverpool (2010-2012). He began his independent career in 2013 in Liverpool, with the award of a Royal Society University Research Fellowship. In 2014, he returned to the ICMol with a Ramón y Cajal Fellowship to lead the design of highly stable MOFs, one of the strategic research lines of the 1st ‘María de Maeztu’ Excellence program awarded to the center. With the award of an ERC Starting Grant in 2016, he established his own research group at the ICMol. The Functional Inorganic Materials team (FuniMat; www.icmol.es/funimat) is focused on the design and processing of porous inorganic materials for biological and environmental-related applications. He has founded the start-ups ‘Porous Materials for Advanced Applications’ S. L. (2018) and ‘Porous Materials in Action’ S. L. (2021) (www.porousinaction.com) to accelerate the transfer of research results into socially useful products and services. He received an ERC Consolidator Grant in 2021 and is one of the guarantor investigators of the 2nd ‘María de Maeztu’ Excellence program of ICMol (2021-2024), and main responsible of the implementation of a new research line for the Molecular Design of Biomaterials in the center.
Since the beginning of his independent career, he has built an international reputation for world leading research recognised with awards, Spanish/European fellowships, invited presentations, talented young scientists attracted/supervised and a sustained competitive funding record as PI near to 8 M€.
In addition to their high surface area, variability in pore size and connectivity, and compositional versatility, the ability to be modified by post-synthetic strategies has helped to tailor the properties of many families of Metal-Organic Frameworks (MOFs) to specific applications by using relatively simple methods. In this contribution, we will discuss how the use of heterobimetallic titanium clusters can enable alternative post-synthetic strategies suitable for directing structural transformations into new ultraporous architectures amenable to isoreticular design, or for integrating additional components with long-range periodicity into a pre-assembled MOF.
In 2018 we introduced a bimetallic titanium SBU, [Ti2Ca2(O)2(RCO2)8(H2O)4] (Ti2Ca2), that combines hard (Ti+4) and soft metal sites (Ca2+).[1] In addition to being compatible with the systematic design of frameworks for controllable pore dimensions and topologies,[2] the dynamic nature of the Ca-O bonds allows integrating compositional and structural changes in the crystal whilst maintaining a periodic structure thanks to the robustness imposed by the Ti+4 nodes. This allows the preparation of crystals of MUV101 and MUV-102, heterometallic titanium MOFs isostructural to archetypical frameworks as MIL-100 and HKUST, by reaction of MUV-10(Ca) with transition metals.[3] This metal-induced topological transformation provides control over the formation of hierarchical micro-/mesopore structures at different reaction times and enables the formation of heterometallic titanium MOFs that are inaccessible under solvothermal conditions at high temperature, thus opening the door for the isolation of additional titanium heterometallic phases not linked exclusively bound to trimesate linkers.[4] We will also illustrate how this combination of hard and soft coordination bonds can facilitate the translocation and ordered positioning of small molecules in these porous architectures.[5] This is triggered by an adaptive response of the solid through a cooperative interplay between the conformational changes of the guest molecule and the reversible reconfiguration of the pore windows through the breaking/formation of coordination bonds with the Ca2+ sites in the framework.
2.3-I2
1.2-I1
Kaifeng Wu obtained his B.S. degree in materials physics from University of Science and Technology of China (2010) and his PhD degree in physical chemistry from Emory University (2015). After his postdoc training at Los Alamos National Laboratory, he moved to China to start his independent research in 2017. His current work focuses on the ultrafast spectroscopy of carrier and spin dynamics in low-dimensional optoelectronic materials, as well as relevant applications in quantum information and energy conversion technologies. He is the winner of the 2022 Distinguished Lectureship Award by the Chemical Society of Japan, 2021 Future of Chemical Physics Lectureship Award by the American Physical Society, 2020 Chinese Chemical Society Prize for Young Scientists, 2019 Robin Hochstrasser Young Investigator Award by the Chemical Physics journal, and 2018 Victor K. LaMer Award by the American Chemical Society. He also serves as the Editorial Advisory Board of J. Phys. Chem. Lett.
Coherent manipulation of solid-state spins is important for quantum information processing. Current solid-state spin systems either operate at very low temperatures or are difficult to scale-up. Colloidal quantum dots (QDs), by contrast, can be synthesized in large quantity in solution at low cost, yet with high finesse in size and shape control. Further, they are usually strongly quantum-confined, thus their carriers well isolated from the phonon bath, which could enable long-lived spin coherence at room temperature. We studied coherent spin dynamics in solution-grown lead halide perovskite QDs using transient magneto-optical spectroscopy. We observed ensemble-level quantum beats resulting from an exciton fine-structure gap and quantitatively controlled the gap energy using temperature-programmable lattice distortion. This unique mechanism has important implications for the application of perovskite QDs in quantum light-sources and coherent exciton control. Further, by dissociating excitons using ultrafast interfacial electron transfer, we achieved room-temperature all-optical initialization, manipulation and readout of hole spins in CsPbBr3 QDs. This represents a milestone towards a scalable and sustainable future of spin-based quantum information processing.
1.2-I2
This talk will review our investigations of the quantum optical properties of single lead-halide perovskite nanocrystals.
Lead halide perovskites indeed exhibit outstanding optical and electronic properties for a wide range of applications in optoelectronics and for light-emitting devices. The physics of their band-edge exciton, whose recombination is at the origin of the photoluminescence, is particularly rich and at the heart of current research. In particular, the long-lived ground exciton of lead halide perovskite nanocrystals plays a major role in the quantum properties of the emitted light, since it promotes the formation of biexcitons and thus the emission of correlated photon pairs.
On the other hand, the production of single indistinguishable photons with quantum emitters is fundamental to many applications in quantum optics, such as linear optical quantum computing, quantum teleportation and quantum key distribution. Our current investigations thus aim at reducing the dephasing rate and spectral diffusion in these materials and improve the indistinguishability character of the emitted photons, which is investigated via two-photon interference (i.e. the Hong-Ou-Mandel experiment).
1.2-O1
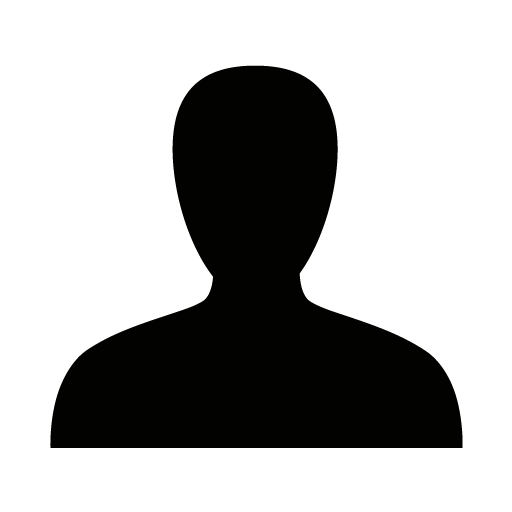
Title: Exciton in Halide Perovskite Nanoplatelets: Finite Confinement and Dielectric Effect in Effective Mass Approximation
Kaouther Tlili,†,‡ (Presenting author) Maria Chamarro,‡ Kais Boujdaria,† and Christophe Testelin ‡
†Université de Carthage, Faculté des Sciences de Bizerte, LR01ES15 Laboratoire de Physique des Matériaux : Structure et Propriétés, 7021 Zarzouna, Bizerte, Tunisia.
‡Sorbonne Université, CNRS, Institut des NanoSciences de Paris, F-75005, Paris, France.
Two-dimensional (2D) lead halide perovskite (LHP) nanoplatelets (NPLs) have garnered significant interest due to their exceptional optoelectronic properties, such as high exciton binding energy, narrow emission lines, and robust room-temperature excitonic stability. These features make them promising candidates for advanced photonic and optoelectronic applications [1], including light-emitting diodes [2], solar cells [3], and photodetectors [4]. Predicting and controlling excitonic properties in these systems is critical for their integration into practical devices.
In this study, we investigate the excitonic properties of CsPbBr3 and CsPbI3 NPLs using an advanced effective mass approximation (EMA) framework. The model incorporates quantum and dielectric confinements, finite potential barriers, and thickness-dependent carrier masses. Additionally, we explore the dependence of Bloch functions on the NPL width, enabling a detailed understanding of how lattice distortions and confinement modulate the electronic states near the band edges. This refined approach addresses the limitations of infinite-confinement models [5,6,7,8] and provides a realistic description of the excitonic behaviour in nanoscale systems.
Our results reveal a strong influence of dielectric contrast and quantum confinement on excitonic energy and binding energy. For thin NPLs, we achieve good agreement with experimental data [9,10], particularly when finite potential barriers and variable effective masses are included. The model demonstrates a significant enhancement in exciton binding energies due to dielectric effects and quantum confinement. Incorporating Bloch function dependence on NPL width further refines the description of excitonic fine structures, revealing critical interactions between carrier delocalization, dielectric mismatches, and finite potential offsets at interfaces.
This work establishes a robust theoretical framework for understanding and predicting the excitonic properties of LHP NPLs. The findings underscore the influence of the ligand environment and its importance on dielectric and finite confinement effects in achieving precise control over excitonic behaviour in quasi-two-dimensional materials.
References:
[1] Li, H. et al. (2021). Energy & environmental materials, 4(1), 46-64.
[2] Gan, X et al. (2017). 2D. Solar Energy Materials and Solar Cells, 162, 93-102.
[3] Cui, J et al. (2021). Science Advances, 7(41), eabg8458.
[4] Liu, X et al. (2017). Small, 13(25), 1700364.
[5] Ghribi, A. et al. (2021).. Nanomaterials, 11(11), 3054.
[6] Rajadell et al. (2017). Physical Review B, 96(3), 035307.
[7] Movilla et al. (2023). Nanoscale Advances, 5(22), 6093-6101.
[8] Gramlich, M et al. (2022). Advanced Science, 9(5), 2103013.
[9] Bohn, B. J et al. (2018). Nano letters, 18(8), 5231-5238.
[10] Wang, S et al. (2022).
1.3-I1
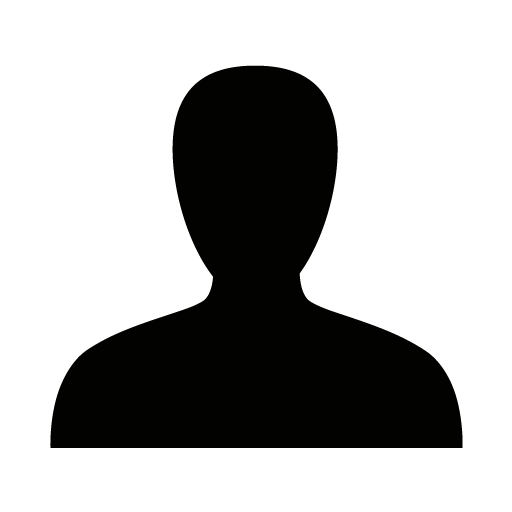
At the center of quantum technology lies the light-matter interactions. Chiral quantum optics is a rising field of research in which the momentum- and spin-dependent asymmetric light-matter interaction offers novel utilization of photonic and electronic degrees of freedom. However, it remained challenging to generate and understand the chiral photon emission in a controlled manner since the physical or structural chirality does not always translate into optical chirality or spin angular momentum (SAM). Here, we report an efficient generation of chiral quantum light in single perovskite quantum dots (PQDs), which are one of the brightest quantum emitters known so far but at the same time are intrinsically achiral.[1] By coupling the PQD with chiral plasmonic particles (Au helicoid),[2] we prompted the emission of single photons with more than an order of magnitude increased degree of circular polarization (DOCP) and Purcell factor of 2-3. Optical helicity of localized plasmon was responsible for the chirality transfer, which was largely dictated by the 3-dimensional structure-defined surface current flow of Au particle. Critical prerequisites for chiral light generation were to finely tune the PQD emitting energy to resonate with optical helicity and at the same time detune from the localized surface plasmon resonance (LSPR) band of the particle. Further, the emission handedness was retained constant under varying excitation conditions, while the photon flux of chiral emission was controlled by the excitation energy near and far above the band edge, which flipped the sign of excitation dissymmetry factor. These results shed light on the largely unexplored mechanism of chiral light-matter interaction and promises the deterministic utilization of chiral quantum light source.
1.3-I2
We are a multidisciplinary and collaborative research team with the overarching goal to establish structure-function relationships by understanding and advancing the fundamental knowledge rooted in the physics, chemistry and engineering of next generation materials for optoelectronics, sustainable, energy conversion, quantum computing, sensing and environmental preservation. Our philosophy is to develop creative and out-of-the-box approaches to solve fundamental scientific problems and apply this knowledge to demonstrate technologically relevant performance in devices.
Colloidal perovskite quantum dots (PQDs) are an exciting platform for on-demand quantum, and classical optoelectronic and photonic devices. However, their potential success is limited by the extreme sensitivity and low stability arising from their weak intrinsic lattice bond energy and complex surface chemistry. Here we report a novel platform of buried perovskite quantum dots (b-PQDs) in a three-dimensional perovskite thin-film, which overcomes surface related instabilities in colloidal perovskite dots. The b-PQDs demonstrate ultrabright and stable single-dot emission, with resolution-limited linewidths below 130 μeV, photon-antibunching (g²(0)=0.1), no blinking, suppressed spectral diffusion, and high photon count rates of 10⁴/s, consistent with unity quantum yield. The ultrasharp linewidth resolves exciton fine-structures (dark and triplet excitons) and their dynamics under a magnetic field. Additionally, b-PQDs can be electrically driven to emit single photons with 1 meV linewidth and photon-antibunching (g²(0)=0.4). These results pave the way for on-chip, low-cost single-photon sources for next generation quantum optical communication and sensing.
1.3-I3
Sascha is a Tenure-Track Assistant Professor in Physical Chemistry and Head of the Laboratory for Energy Materials at EPFL (Switzerland), while he is also maintaining strong ties with the Harvard community and in particular Winthrop House which he regularly visits as NRT and SCR member.
His team employs light-matter interactions to understand the next generation of soft semiconductors with the overarching goal of maximizing energy efficiency for a sustainable future by unlocking applications ranging from flexible light-weight solar cells & displays all the way to entirely new applications in quantum information processing.
Previously, he was a research group leader and Rowland Fellow at Harvard University. Before starting his lab at Harvard, Sascha studied Chemistry at Heidelberg University (Germany) and completed a PhD in Physics at the University of Cambridge (UK), where he subsequently worked as EPSRC Doctoral Prize Fellow.
Solution-processable semiconductors like halide perovskites and certain molecules are promising for next-generation spin-optoelectronic applications [1]. Yet, we don’t fully understand what governs spin and light polarization in these materials, and even less how these are affected by chirality.[2]
In this talk, I will give an overview of our recent efforts to understand the spin-optoelectronic performance of these materials through time-, space- and polarization-resolved spectroscopy and microscopy.
For investigating halide perovskite films, we pushed broadband circular dichroism to diffraction-limited spatial and 15 fs time resolution for creating a spin cinematography technique to witness the ultrafast formation of spin domains due to local symmetry breaking and spin-momentum locking [3].
I will then briefly explain the fundamentals and artefacts involved in measuring circularly polarized luminescence reliably and introduce an open-access methodology and code to do so [4]. Finally, I will show our most recent development of a transient sensitive broadband full Stokes-vector spectroscopy with unprecedented time- and polarization resolution to track the emergence of chiral light emission [5].
[1] Nature Reviews Materials 8, 365 (2023)
[2] Nature Reviews Chemistry, in press (2024/25)
[3] Nature Materials 22, 977 (2023)
[4] Advanced Materials 35, 2302279 (2023)
[5] unpublished (2024/25).
2.1-I1
Jacky Even was born in Rennes, France, in 1964. He received the Ph.D. degree from the University of Paris VI, Paris, France, in 1992. He was a Research and Teaching Assistant with the University of Rennes I, Rennes, from 1992 to 1999. He has been a Full Professor of optoelectronics with the Institut National des Sciences Appliquées, Rennes,since 1999. He was the head of the Materials and Nanotechnology from 2006 to 2009, and Director of Education of Insa Rennes from 2010 to 2012. He created the FOTON Laboratory Simulation Group in 1999. His main field of activity is the theoretical study of the electronic, optical, and nonlinear properties of semiconductor QW and QD structures, hybrid perovskite materials, and the simulation of optoelectronic and photovoltaic devices. He is a senior member of Institut Universitaire de France (IUF).
Perovskite quantum dots are attractive semiconductor nano-objects for quantum light emission. Although initial electronic and excitonic fine structure calculations were reasonably predictive for quantum confinement and magnetic field effects, questions related to the coupling of the quantum confined excitonic states to the lattice are still open. This presentation will give some insights on electron coupling to the lattice, excitonic polarons and lattice polymorphism in the 3D halide perovskites used as active materials in perovskite quantum dots. New results on the empirical modelling of Fröhlich excitonic polarons in the context of coupling to multiple phonons will be given. The refinement of empirical excitonic polaron models necessary to fully account for the variation of the exciton barycenter and reduced masses as a function of the coupling parameters will be described, The crossover from the weak coupling regime to the medium coupling regime for excitonic polarons will be quantified by the variations of the virtual phonon populations, effective dielectric constants, barycenter and reduced masses and Huang-Rhys factors. The connection with empirical modelling of free polarons will be proposed using Lee-low and Pines theory to define exciton binding energies and to stress the interplay between populations of virtual phonons and electron-hole correlations.
2.1-I2
Prof. Z. Hens received his PhD in applied physics from Ghent University in 2000, worked as a postdoctoral fellow at Utrecht University and was appointed professor at the Ghent University department of inorganic and physical chemistry in 2002. His research concerns the synthesis, processing and characterization of colloidal nanocrystals.
Semiconducting perovskites hold great promise for demanding applications involving light emission, such as lasers. In this respect, fully inorganic colloidal CsPbBr3 nanoplateltes (NPLs) could be of interest since somewhat similar colloidal CdSe NPLs showed exceptionally high material gain related to stimulated emission through the biexciton-exciton transition[1].
Here, we report on a spectroscopic study of CsPbBr3 2D NPLs, geared towards understanding the photo-excited states and evaluating the performance metrics related to optical gain. In line with literature reports, we show that photo-excitation of 2D CsPbBr3 NPLs leads to the formation of strongly bound excitons. Importantly, the pronounced difference between the oscillator strength of the ground-state-to-exciton transition in absorption and emission points towards considerable exciton localization, for which we estimate a coherence area of a mere 1-2 nm2. Furthermore, we show that this localization comes with the formation of an exciton-polaron, which shifts the exciton emission to the red of the exciton absorption.
Through pump-probe spectroscopy using co-polarized and cross-polarized pump and probe beams, we demonstrate that light absorption in the presence of excitons leads to bound biexcitons. These biexcitons have singlet character, and the biexciton emission exhibits an additional Stokes shift with respect to the biexciton absorption. Furthermore, the oscillator strength of this transition indicates that the biexciton area is more than 5 times smaller than the cross-sectional area of the exciton. Both elements suggest that like excitons, biexcitons couple with the CsPbBr3 lattice to form strongly localized biexciton polarons.
After fs optical pumping, CsPbBr3 exhibit a strongly redshifted, weak and short-lived band of net stimulated emission. Using the exciton and biexciton characteristics, we can account for this transient optical gain by assuming stimulated emission across the biexciton-exciton transition. We relate the limited gain performance – as compared to, for example, CdSe NPLs – to the pronounced localization of the biexciton or biexciton polaron.
2.1-O1
Colloidal semiconductor nanocrystals are a candidate source of time-correlated and entangled photons through the cascaded radiative relaxation of multiexcitonic states (multiple excitons within the same nanocrystal). However, the efficient nonradiative Auger-Meitner decay of multiexcitons renders them mostly nonemissive. This limits not only potential uses but also their investigation, particularly their spectroscopy. I will present the heralded spectroscopy of three-photon cascades from triexcitons in giant CsPbBr3 nanocrystals at room temperature.[1] Heralded spectroscopy, realized previously in our group,[2] is a single-particle technique using a single-photon sensitive, 180-picosecond time-resolved spectrometer based on a single-photon avalanche diode (SPAD) array. By post-selecting events of triple photon detections following a single laser pulse, we isolate the triexciton relaxation cascades. This allows us to resolve the weak binding energies associated with the triexciton and the biexciton emissions in the cascade (1.13 ± 0.27 and 0.46 ± 0.28 meV, respectively). Simultaneously, we measure the lifetime of each relaxation step individually, despite their high similarity (triexciton: 0.51 ± 0.08 ns, biexciton: 0.82 ± 0.11 ns, and exciton: 2.21 ± 0.17 ns). These nanocrystals also exhibit near unity values of the second- and third-order correlation functions, g(2)(0) = 0.97 ± 0.01 and g(3)(0,0) = 0.94 ± 0.02. Those weak exciton–exciton interactions are in accordance with the nanocrystals’ diameter of 26 nm, more than four times the exciton Bohr diameter in CsPbBr3 of 7 nm,[3] whereas stronger interaction and binding were previously found in smaller-size (6 nm edge) CsPbBr3 nanocrystals by our group, studying biexcitons using the same technique.[4] We also combine fluorescence lifetime analysis, photon statistics, and spectroscopy, to verify emission from a single emitter despite the high emission quantum yields of multiply excited states and the comparable emission lifetimes and energies of singly and multiply excited states. I will also compare the biexciton and exciton emission properties during fluctuations in the nanocrystal’s emissivity (“blinking” between on, gray, and off states). Such blinking is typically attributed to Auger-Meitner recombination with excess charges or to surface-trap recombination. I will present indications that blinking can change the multiexciton-to-exciton emission rate ratio, which could be a potential pathway toward control of the photon number statistics of multiexcitonic emission cascades. Finally, I will discuss how this effect changes in nanocrystals of different sizes.
2.1-O2
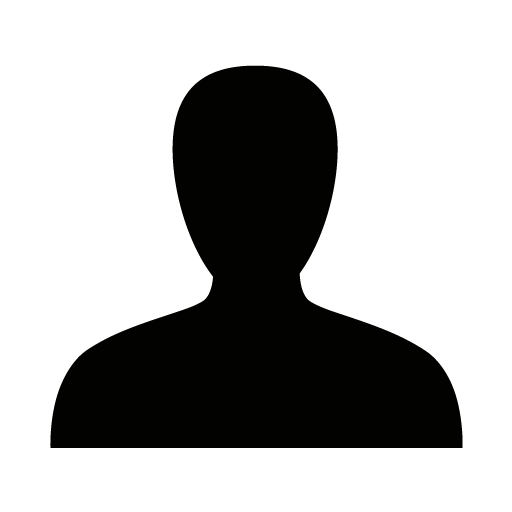
Colloidal perovskite nanocrystals have emerged as promising candidates for next-generation quantum light sources based on their excellent optical properties. In this context, the method to optically prepare the emitting states is a critical step. While previous works on epitaxial quantum dots have stablished that resonant excitation can improve the performance of quantum emitter when compared to above-bandgap excitation by avoid the dephasing processes involved in hot carrier cooling, low-background resonant excitation of a single colloidal perovskite nanocrystal has not yet been achieved. Here, we demonstrate resonant three-photon excitation (3PE) of a single perovskite nanorod at both room temperature and cryogenic temperature. Third-harmonic generation, arising as a background created from the substrate interface is observed and further eliminated using polarization by a factor of 105, resulting in a signal to background ratio larger than 100. The giant three-photon absorption cross-section at room temperature is measured to be around 10-76 cm6·s2·photon-2 at resonance, which is two orders of magnitude larger than those of traditional CdSe-based nanocrystals. Additionally, similar fluorescence and blinking properties between upper-bandgap 1PE and resonant 3PE are observed in room temperature, highlighting the sample’s photostability under high power NIR beams. This low-background resonant three-photon excitation method has great potential to improve the optical coherence properties of perovskite nanocrystal.
2.2-I2
Metal halide perovskites possess unique optical and electronic properties, including high light absorption, high mobility, tuneable bandgaps and high defect tolerance, and these properties make them very promising for achieving high performance optoelectronic devices. Here we demonstrate several examples of using perovskites as emitters for applications in multifunctional displays, visible light communications (VLCs) and quantum random number generators.
Firstly, we develop multifunctional displays using highly photo-responsive metal halide perovskite LEDs (PeLEDs) as pixels. With efficient defects passivation of perovskite layers, the red emissive PeLEDs shows an external quantum efficiency (EQE) of around 10% when working at LED model and a power conversion efficiency (PCE) of 5.34% at photovoltaic model. Due to the strong photo response of the PeLED pixels, the display can be simultaneously used as touch screen, fingerprint sensor, ambient light sensor, and image sensor without integrating any additional sensors. In addition, decent light-to-electricity conversion efficiency of the pixels also enables the display to act as a photovoltaic device which can charge the equipment.[1] The multiple-functions of our PeLED pixels can not only simplify the display module structure and realize ultra-thin and light-weight display, but also significantly enhance the user experience by these advanced new applications, and this is a feature hardly possible for conventional LED technologies.[2]
Additionally, we further demonstrate an all perovskite based visible light communication (VLCs) system with PeLEDs and PePVs, and quantum number random generator based on PeLEDs.[3] These demonstrations show that perovskite based optoelectronics will have special advantages in the future for high performance and low-cost telecommunication devices.
2.2-O1
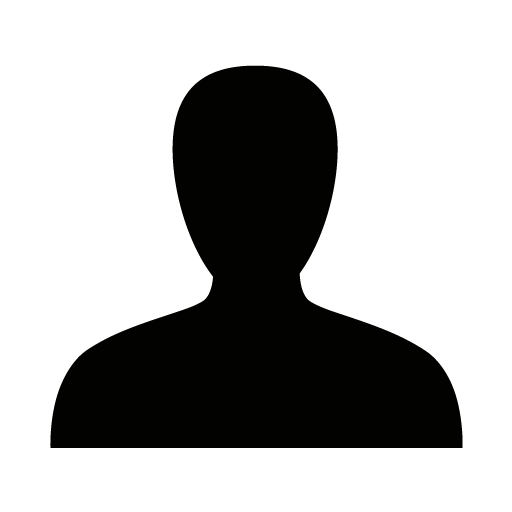
The ability to control the emission of individual nanocrystals through external electric fields has garnered significant interest in the field of quantum technology. Among the most promising materials for quantum emitters are colloidal lead halide perovskite nanocrystals due to near unity quantum yields and their flexibility in size, shape, and composition engineering. An external electric field induces the quantum-confined Stark Effect (QCSE), which is expected to result in a reduced overlap of the electron and hole wavefunction and an energy shift of the bandgap. This allows to extract polarizability and permanent dipole moment, the latter affecting, e.g., the Rashba effect in lead halide perovskite nanocrystals. Moreover, the field-induced tuning of the excitonic fine structure[1] in single nanocrystals may pave the path for single or entangled photon emitters.
Here, we present an approach of polarization-resolved photoluminescence (PL) spectroscopy on highly anisotropic, single CsPbBr3 nanorods (NRs) for exploring the QCSE under a well-defined angle between crystal axes and electric field[2]. A polarizability of 23 meV/(MV/cm)2 and a permanent dipole of 6.4*10-30 Cm could be extracted, e.g., for a single CsPbBr3 NR aligned at 65° relative to an applied electric field of up to ± 333 kV/cm. We observe a clear correlation between energy shift, spectral line width, and PL intensity under the influence of the QCSE. A detailed analysis on a statistically relevant number of NRs revealed an interesting correlation between PL intensity and polarizability: The higher the PL intensity, the lower the polarizability. Comparing aged NRs with low PL intensities with freshly synthesized ones shows that 44% of the aged NRs reveal a polarizability larger than our error bar (i.e., > 3 meV/(MV/cm)2, whereas only 7% of the fresh NRs show a similar finding. Our work thus highlights new insights and challenges in terms of modulating single lead halide perovskite nanocrystals in directional electric fields.
2.2-I1
Generating and manipulating non-classical light in the form of a stream of single photons is central to a broad range of emerging quantum-light applications, from quantum computing to quantum sensing and quantum imaging. In this talk, I will present recent advances in using colloidal lead-halide perovskite quantum dots (QDs) towards this end, with advantageous attributes such as spectral tunability, solution-processability, and scalability.
Sharing results from single-particle spectroscopy and ab-initio molecular-dynamics simulations, I will argue that the pronounced exciton-phonon coupling in perovskite QDs[1] is key to understanding and manipulating (multi)excitons in these materials. To illustrate this point, I will elaborate on the strikingly different photophysics in individual perovskite QDs at cryogenic and room temperature. At cryogenic temperature, perovskite QDs behave as textbox semiconductors, capable of cavity-free coherent quantum-light emission and hosting phenomena such as single-photon superradiance[2] and superabsorption.[3] The latter two concepts have both been proposed theoretically early on but evaded experimental demonstration until very recently. At room temperature, on the other hand, coupling of the exciton to large-amplitude lattice vibrations in the QD core and at the QD surface leads to pronounced emission broadening[4] and localization of the exciton wavefunction.[5] While the latter inevitably accelerates thermally activated decoherence processes, it can auspiciously be leveraged to increase single-photon purity, up to 98% for cavity-free, nonresonantly excited single perovskite QDs at room temperature.[6]
References:
[1] C. Zhu, et al., Adv. Optical Mater. 2024, 12, 2301534.
[2] C. Zhu, et al., Nature 2024, 626, 535–541.
[3] S.C. Boehme, et al., under review.
[4] G. Rainò, et al., Nat. Commun. 2022, 13, 2587.
[5] L.G. Feld, at al., DOI: 10.48550/arXiv.2404.15920.
[6] C. Zhu, et al., Nano Lett. 2022, 22, 3751–3760.
2.2-O2
Lead halide perovskite quantum dots (PQDs) have emerged as promising materials for advanced optoelectronic applications due to their tunable excitonic properties.[1] However, achieving strong light-matter coupling in PQD films has been challenging due to issues with film quality, weak oscillator strengths and spectral diffusion.[2] Leveraging advances in PQD synthesis and film preparation,[3] we present the successful formation of uniform, thick, scattering-free films with well-defined excitonic transitions. By coupling these highly transparent cesium lead bromide (CsPbBr3) PQD solids with resonant modes of metallic resonators, multiple cavity exciton-polaritons at room temperature are obtained, evidenced by significant alterations in the absorption and emission spectra.[4]
Unlike traditional PQD systems, the dynamics observed by transient absorption spectroscopy (TAS) is dominated by the interplay of polaritonic states with dark-state reservoirs, while effects such as polaron formation are seemingly absent. The study also reports a substantial reduction in photoemission linewidth and ultrafast modulation of optical absorption properties on the picosecond timescale. These insights establish the groundwork for developing polaritonic devices with tunable photophysical properties and lay the foundation for pursuing phenomena like Bose-Einstein condensation in solid-state systems.[4]
1.1-I1
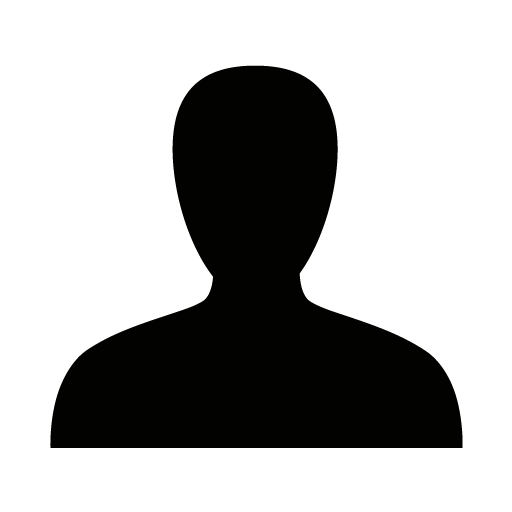
Efficiencies of lead halide perovskite photovoltaics (LHP PVs) have increased to over 26%, and over 30% for tandems, and potential for low manufacturing costs puts them on track for near-future commercialization. While most efforts to date have focused on stability and scalability of LHP PVs, the environmental impact of their manufacturing should be considered, and concern regarding the toxicity of lead (Pb) remains a major challenge to large-scale commercialization. This invited talk will describe two recent studies related to (1) life cycle assessment of cation precursors, and (2) fate of toxic Pb leachate from hypothetical breakage of fielded module arrays.
Devices exhibiting the best combination of high efficiency and long operational lifetimes have used mixed cation perovskite absorber layers such as cesium / methylammonium / formamidinium lead iodide (CsxMAyFA1-x-yPbI3). However, the associated environmental burdens of the supply chains of perovskite precursors should also be considered when selecting compositions for commercialization. Prior literature based on laboratory-scale data reported a particularly high environmental burden for FA and warned against using these highest-performing film compositions. Here we used updated data sources, process scale-up concepts, and sensitivity analysis to build commercial-scale life cycle inventory (LCI) models for perovskite precursors. Our life cycle assessment results indicate that the environmental burdens of CsI, MAI, and FAI are similar to each other. This conclusion reveals that the composition can be selected based on PV efficiency and operational stability, without additional constraints of environmental impact. The current cesium supply appears sufficient for near-future perovskite deployment. Our commercial-scale LCI models for perovskite films aid in more transparent and robust environmental analysis that can contribute to industrial manufacturing choices.
Second, we will present a screening-level, EPA-compliant model of fate and transport of Pb leachate in groundwater, soil, and air following hypothetical catastrophic breakage of LHP PV modules in conceptual utility-scale sites. We estimated exposure point concentrations of Pb in each medium and found that most of the Pb is sequestered in soil. Exposure point concentrations of Pb from the perovskite film fell well below US EPA maximum permissible limits in groundwater and air even upon catastrophic release from PV modules at large scales. Background Pb levels in soil can influence soil regulatory compliance, but the highest observed concentrations of perovskite-derived Pb would not exceed EPA limits under our assumptions. Nonetheless, regulatory limits are not definitive thresholds of safety, and the potential for increased bioavailability of perovskite-derived Pb may warrant additional toxicity assessment to further characterize public health risks.
1.1-I2
The shift to renewable energy sources is essential for achieving global sustainability and reducing carbon emissions. Solar energy, alongside wind power, plays a central role in this transition, with annual photovoltaic production projected to exceed 1 terawatt peak (TWP) by 2025. However, the exponential growth in solar panel deployment brings critical challenges regarding end-of-life management. Sustainable recycling strategies are urgently needed to minimize environmental impact and ensure the efficient recovery of valuable materials. Embracing a circular recycling model not only mitigates the risks of landfilling but also supports resource conservation and the long-term sustainability of solar technologies.
Perovskite solar cells (PSCs), a next-generation photovoltaic technology, offer tremendous potential due to their high efficiency, low material costs, and scalability. Yet, the sustainability of PSCs depends on overcoming significant recycling and environmental hurdles, particularly the safe recovery and reuse of lead halides. Our research addresses these challenges by focusing on the circular recovery of materials, developing scalable processes that minimize waste and enable the reintegration of critical resources into the production cycle.
In the context of closed-loop recycling, we demonstrated a novel solvent-based layer-by-layer extraction process that enables the recovery of MAPbI₃ perovskite solar cells with a 99.97% mass recovery rate. Essential components such as ITO glass, SnO₂, MAPbI₃, and spiro-OMeTAD were successfully purified and reused. Solar devices fabricated using recycled materials retained performance comparable to those produced with virgin components, achieving efficiencies of 19%. A techno-economic analysis revealed that adopting this recycling strategy could reduce material costs by 63.7% at the laboratory scale, highlighting its cost-effectiveness and potential for broader adoption.
To address the environmental concerns associated with lead waste, we developed an innovative recycling process to convert contaminated lead into high-purity lead iodide (PbI₂). This method achieves near-complete conversion with a Faradaic efficiency close to unity. Single-crystal growth purification resulted in PbI₂ of 35% purity, with residual materials fed back into the recycling loop. The process demonstrated a scalable output of approximately 1g per hour, with clear pathways for industrial upscaling. Fabricating perovskite solar cells from the recovered PbI₂ resulted in efficiencies exceeding 20%, confirming the viability of repurposing lead waste for sustainable photovoltaic production.
By transforming legacy lead waste from industrial and household sources into high-value materials for new solar cells, our work provides a scalable and environmentally responsible solution to lead recycling. This approach not only eliminates ecological hazards but also advances a circular economy, where resource efficiency and waste minimization drive the sustainability of perovskite solar technologies.
1.1-O1
Abhinandan Patra
Abhinandan Patra was born and brought up in Puri, the spiritual capital of Odisha, India, where he finished his education upto M.Sc. in Physics. He was awarded with a PhD degree in Physics from CNMS, Jain University, Bangalore, India. During the tenure of his PhD, he was awarded a prestigious fellowship from the Ministry of Foreign Affairs and International Cooperation (MAECI) under the Italian government and awarded as the best researcher scholar and gold medalist among 100 PhD scholars. Lately, he has been working on the integration of solar cell with supercapacitor for photosupercapacitor application at CHOSE, University of Rome Tor Vergata, Rome, Italy as a post doc fellow.
Tackling the global energy crisis demands the seamless integration of energy harvesting, conversion, and storage systems into a unified module. Such systems mitigate the inconsistencies of renewable energy sources, making them ideal for portable optoelectronic devices operating in both outdoor and indoor lighting conditions. [1-2] In this study, we present an innovative approach by integrating a carbon perovskite solar cell (CPSC) with an MXene-based in-plane microsupercapacitor (MSC) on a single substrate. The three-terminal device, constructed on a glass/indium tin oxide (glass/ITO) substrate, features a shared terminal between the MSC and the solar cell, alongside two dedicated terminals for individual components. The MXene-based MSC was developed using blade-coating of water-processed Ti₃C₂Tₓ MXene ink onto the substrate, followed by laser scribing to form an interdigitated in-plane structure. This MSC achieved a capacitance of 16 mF/cm² at a current density of 0.08 mA/cm² and exhibited impressive durability, retaining 86% of its capacitance and delivering a coulombic efficiency of 96% after 6,000 charge-discharge cycles. The photovoltaic component demonstrated a conversion efficiency of 7.11% under standard sunlight conditions (1 sun) and 22.6% under indoor lighting at 1000 lx. The integrated device showcased exceptional performance across varying light environments, achieving an overall efficiency of 3.8% and a storage efficiency of 59.1%. This breakthrough photosupercapacitor represents a significant step forward for portable optoelectronic devices, paving the way for advanced solutions in energy storage and conversion technologies.
1.1-O2
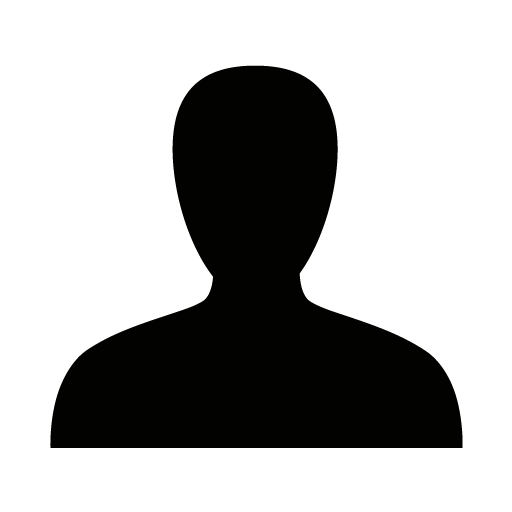
Halide perovskites have gained a lot of attention in energy storage applications due to their high electrical and ionic conductivity, large diffusion coefficients and ease of structural dimensions. However, the use of toxic solvents and lead in the synthesis processes has limited their potential for commercial development [1]. To overcome these challenges, we developed the mechanosynthesis of lead-free 2D and 3D perovskite powders for supercapacitor applications. Using this scalable and solvent-free ball-milling technique, we have synthesized methylammonium copper bromide (MA2CuBr4), cesium bismuth chloride (Cs2Bi3Cl9) perovskites, and MA2CuBr4-graphite composite powders which were composed of aggregates of nanograins. After mechanosynthesis, MA2CuBr4 powders were washed and centrifuged in methyl acetate several times to obtain the orthorhombic crystal structure (with Pbca space group) and confirmed by the x-ray diffraction analysis [2]. MA2CuBr4 was first studied as an electrode material for supercapacitor and we have tested two electrolytes/working electrode systems. In the optimized LiPF6 electrolyte/Ti electrode system, MA2CuBr4 showed stable and high average specific capacitance of 205 Fards/gram (Fg-1) for 20 cycles as a working electrode thanks to the 2D layered morphology suitable for lithium intercalation. To the best of our knowledge, the performance of MA2CuBr4 perovskite was not studied for supercapacitor electrode application but is comparable to the recently reported lead-free bismuth-based perovskite (Cs3Bi2I9) supercapacitor with a specific capacitance of ~242 Fg-1 [3]. A maximum current of up to 20 miliamperes (mA) was observed at voltage of 1.72 V during the large operating voltage range of 0.3 V to 3.2 V. Witnessing the high performance of MA2CuBr4 as a supercapacitor, perovskite-graphite composites were synthesized using one-pot and two-pot schemes. In graphite-based composite powders, the graphite few layers were observed to distribute homogeneously within powders, indicating strong graphite-perovskite interaction. The effect of graphite addition was also studied for improving the electrochemical performance. These results demonstrated at first the great potential of mechanosynthesis, which is a green and easy-scalable powder synthesis technique to develop electrode materials for supercapacitors. This was confirmed by further experiments with another mechanosynthesized perovskite, Cs2Bi3Cl9. The cyclovoltametric and charge/discharge experiments with Cs2Bi3Cl9, MA2CuBr4, and MA2CuBr4-graphite composite have allowed for better evidencing the interest of such layered materials. Thus, mechanosynthesis shows a promise for creating eco-friendly, high-efficiency lead-free hybrid perovskite devices for sustainable energy storage applications.
Keywords : lead-free perovskites, green chemistry, solvent-free mechanosynthesis, electrode materials, supercapacitors, energy storage
1.2-I1
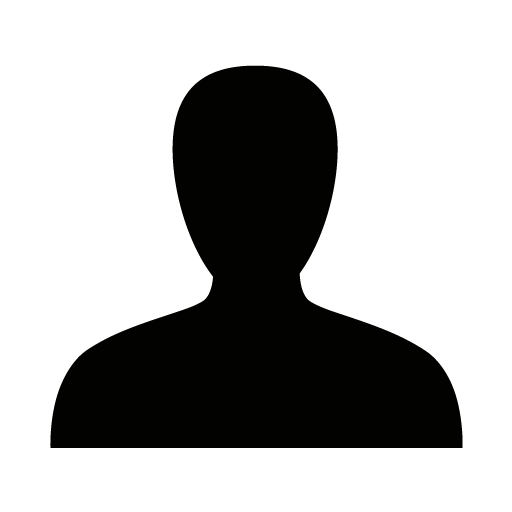
Halide perovskite solar cells (PSCs) have rapidly approached the efficiency levels of traditional silicon photovoltaics, marking a significant breakthrough in solar technology. However, their commercial viability is compromised by their limited long-term stability. This presentation focuses on the recent strides made in enhancing the stability of PSCs through innovative interface engineering. By examining the primary factors influencing PSC stability and the mechanisms of device degradation, we have explorde how interface manipulation serves both as a corrective measure for surface defects and a defensive mechanism against environmental degradation. Special emphasis will be placed on the latest advancements in interface modification agents, interfacial regulation strategies, and the design of novel charge transport and capping layers. We aim to provide a comprehensive overview of the current challenges, recent technological advances, and future directions in the sustainability of halide perovskite photovoltaics, emphasizing the pivotal role of interface engineering in the evolution of this promising technology.
1.2-I2
Thanks to their high absorption coefficient and ideal band-gap [1], lead halide perovskite materials are strong candidates for the next generation of solar cells, achieving certified power conversion efficiencies of over 26%. However, the development of perovskite-based solar cells is impeded by obstacles such as degradation of the perovskite layer by light, oxygen, and moisture. Addressing these challenges while advancing sustainability is critical for their widespread adoption.
Photoluminescence (PL) is a valuable tool for studying photoexcited carrier processes in solar cells. However, measuring the steady-state and time-resolved PL of perovskite thin films reveals the complex and sometimes surprising behaviors of these materials [2–4]. Here, we discuss the use of PL studies to understand the stability and performance of perovskite materials, particularly in evaluating alterations to manufacture and materials to improve sustainability. This includes the use of sustainable solvent systems and material substitutions, such as carbon electrodes as alternatives to gold. These studies are complemented by XRD and SEM analyses to investigate the effects of material and process changes on morphology, uniformity, and photovoltaic device performance.
Here, we discuss the work of the Applied Photochemistry Group at the SPECIFIC Innovation and Knowledge Centre, Swansea University, towards advancing the understanding of photostability and photochemistry of perovskite solar cells. By addressing sustainability challenges, particularly through solvent and material innovations, this research supports the transition from lab-scale to production-scale manufacture of environmentally responsible perovskite PV.
1.2-O1
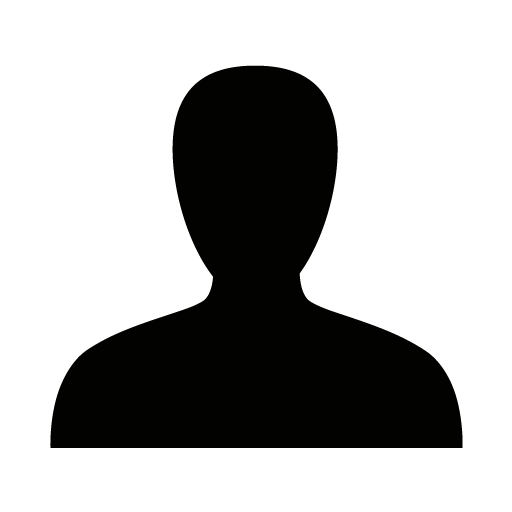
The huge expansion of nanotechnology calls for searching processes that can reduce the environmental impact of the syntheses employed to obtain nanocrystals (NCs).
In this contribution, we present the preparation of lead halide perovskites (CsPbX3, X = Cl, Br, I) in limonene, a molecule extracted from natural sources and considered a green solvent. [1] The synthesized NCs resemble the same structural, optical and morphological properties of the homologues prepared in the common solvents, i.e. 1-octadecene. In particular, the NCs resemble cubic morphologies and possess a considerable photoluminescence quantum yield (> 80% for CsPbBr3). Exploiting the relatively high volatility of the limonene, we verified that it can be quantitatively removed from the NCs just by vacuum pumping and demonstrated the possibility of recovering from the waste of the reactions the pure solvent that can be reused for subsequent syntheses. In addition, the substitution of 1-octadecene with limonene as the reaction solvent was analyzed by examining the consequences of the environmental impact of the entire synthesis process through a Life Cycle Assessment (LCA).
1.3-I1
Dr. Annalisa Bruno is an Associate Professor Nanyang Technological University (ERI@N), coordinating a team working on perovskite solar cells and modules by thermal evaporation. Annalisa is also a tenured Scientist at the Italian National Agency for New Technologies, Energy, and Sustainable Economic Development (ENEA). Previously, Annalisa was a Post-Doctoral Research Associate at Imperial College London. Annalisa received her B.S., M.S., and Ph.D. Degrees in Physics from the University of Naples Federico II. Her research interests include perovskite light-harvesting and charge generation properties and their implementation in solar cells and optoelectronic devices.
Metal-halide perovskites (MHP) are highly promising optoelectronic materials due to their exceptional properties and versatile fabrication methods [1]. These materials are crucial for solar cells and optoelectronics devices to quantum emitters.
Thermal evaporation has emerged as a pivotal method to enable precise control over film thickness, composition tuning, stress-free deposition, surface modification capabilities, and large-area [2] devices—key factors in the advancement of perovskite optoelectronics.
In this talk I will present how overcoming the challenges of scalability and reproducibility in perovskite solar cell (PSC) production, critical for their sustanaible viability. We have demonstrated the possibility of achieving a sixfold increase in speed while maintaining film quality and high-power conversion efficiencies. This accelerated co-evaporation process demonstrates the potential for large-scale, cost-effective PSC production without needing post-annealing, further simplifying the manufacturing process [3].
Moreover, leveraging on the possibility of fabricating high-quality films with high thickness control by thermal evaporation we have explored the promising field of thermally evaporated perovskite-based Multiple Quantum Wells (MQWs) [4]. MQWs structures can enhance the optoelectronic properties of nanoscale thin films, through control over electronic energy levels and quantum mechanical phenomena, and opening up avenues for unconventional optoelectronic functionalities.
We demonstrated how both thermally evaporated MAPbI3-based MQWs offer significant advancements in light-emitting and photodetection technologies, expanding their sensitivity into the near-infrared range and enhancing photoluminescence and charge separation efficiency.[5].
These recent works not only address critical challenges in scaling perovskite solar cell production but also open new avenues for optoelectronic device design, highlighting the versatility and promise of thermally evaporated perovskite materials for next-generation energy solutions.
References
1) Min, H., et al., Nature, 2021. 598, 444.; Yoo, J.J., et al., Nature, 2021. 590 587.
2) J. Li et al., Joule 2020, 4, 1035; H.A. Dewi et al., Adv. Funct. Mater. 2021, 11, 2100557; J. Li et al., Adv. Funct. Mater. 2021, 11, 2103252;
3) Dewi et al. ACS Energy Lett. 2024, 9, 4319−4322
4) Advanced Materials 2021, 33, 2005166; L. White et al. ACS Energy Lett. 2024, 9, 83;
5) L. White, ACS Energy Lett. 2024, 9, 4450.
1.3-I2
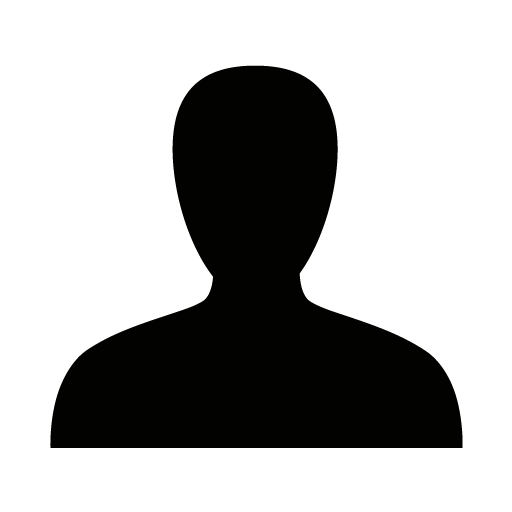
Climate change is a race against time, and the need for more efficient and cheaper solar energy is a high priority. Perovskite-silicon tandem solar cells are among the most promising options that can significantly contribute to the decarbonization of the global energy system through their increased power output. Two main sustainability considerations for any renewable technology that dictate whether it can fulfil its promises are efficiency and lifetime.
Perovskite-silicon tandem solar cells have made unprecedented strides in the field of photovoltaics (PV) regarding efficiency. At Oxford PV, we have demonstrated world-record efficiencies at both small-scale and full wafer scale. Last year, we revealed a 26.9% 60-cell module, which remains a record for a residential-size photovoltaic module. Nevertheless, long-term stability remains a major concern across the research community. Established PV technologies such as silicon (c-Si) and cadmium telluride (CdTe) have set the long-term stability benchmark at a 0.25-0.5% relative loss per year, with an expected lifetime of up to 25-30 years.
We are confident that perovskite PV can maintain high performance over multiple decades. In this talk, we will discuss the major R&D approaches we are following to achieve this goal. Unlike incumbent PV technologies, where outdoor performance data and knowledge of real-world degradation modes and their characteristics are available, it is imperative to deploy perovskite PV in the field before having performed outdoor testing for decades to demonstrate the reliability of the solar panels. Accelerated stress testing is designed to expose PV cells and modules to a variety of stressors that can mimic expected real-world conditions – the combined light and heat exposure test is a common route to evaluate the cumulative affect of these stressors in the field, and typically elevated temperatures are chosen to compress ‘years of operation’ into week-to-month long experiments. In this way, relevant degradation modes can be triggered and rapid iteration of technology can be made, However, it is crucial to both: a) choose the relevant parameter ranges that activate the degradation processes prominent under field-deployment conditions whilst avoiding the triggering of degradation artifacts unique to the stress conditions; and b) have consideration as to the means in which cell parameters are determined, either during, or following, stress tests.
By selecting the right stressing conditions and employing suitable models, we can calculate an acceleration factor and estimate real-world operation lifetime, which can be validated by comparing with corresponding outdoor performance data, providing reliable lifetime predictions. We will present activity at Oxford PV for each of these stages. The range of degradation modes in perovskites are well known, and have been reviewed extensively in the literature. A key consideration for enhancing cell performance longevity however, is to identify the critical degradation modes, those that dominate the measured power output. dAt Oxford PV, we focus on identifying the link between material properties and changes with device losses for the critical degradation modes, aim to introduce mitigating routes, and move to the next critical mode. Representative examples of optimized device performance and stability will be discussed, Lastly, looking towards scalability, being able to repeatedly obtain high-efficiency and stable devices is of paramount importance. By continuously tracking our device stability, we gain more insights into the correlation of process capability and reproducibility. This allows us to identify process windows and parameters affecting performance and stability, informing the design of scalable processes that can be transferred from R&D to high-throughput manufacturing.
1.2-I1
Copper chalcogenide-based nanocrystals (NCs) are a suitable replacement for toxic Cd/Pb chalcogenide NCs in a wide range of applications including photovoltaics, optoelectronics, and biological imaging. However, despite rigorous research, direct synthesis approaches of this class of compounds suffer from inhomogeneous size, shape, and composition of the NC ensembles, which is reflected in their broad photoluminescence (PL) band widths. A partial cation exchange (CE) strategy, wherein host cations in the initial binary copper chalcogenide are replaced by incoming guest cations to form ternary/quaternary multicomponent NCs, offers a valuable alternative.
A straightforward synthesis of binary copper chalcogenide NCs[1-2] provides a perfect basement for obtaining fairly monodisperse multicomponent particles by replacing a part of host copper ions by guest cations of choice. This partial CE allows for a precise control of the composition of the exchanging NCs by simple tuning the ratio between host particles and incoming cation precursors, while preserving the size, shape, and crystal structure of template NCs.[3] Host copper ions can easily be replaced by a range of different metal cations, making copper chalcogenides a universal platform to prepare different metal chalcogenide structures, otherwise inaccessible from direct synthesis, including 2D nanomaterials.[4-5] Partial Cu+-to-In3+ CE yields Cu-In-S[6] and Cu-In-Se[7] NCs with a uniform distribution of all elements over individual particles. At the same time, the simultaneous incorporation of In3+ and Zn2+ into Cu2-xSe NCs results in gradient alloyed Cu-Zn-In-Se NCs with a Zn-rich surface.[8] Without an additional shell growth these NCs exhibit near-infrared PL with narrow bands, reaching quantum yields of 20%. These results evidence that synthetic approaches that help to eliminate inhomogeneities in the NC ensembles can lead to narrower PL spectra. The large Stokes shift inherent to these materials, their absorption in the solar range, as well as their near-infrared PL within the biological window make them suitable candidates for applications in the area of solar energy harvesting and bioimaging.
1.2-O1
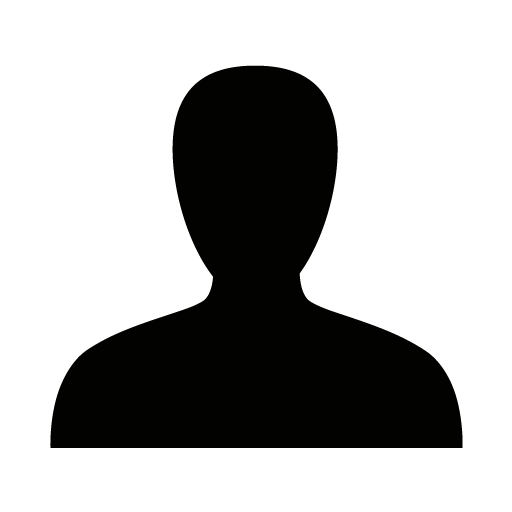
Eco-friendly I-III-VI quantum dots (QDs) are emerging as promising materials for next-generation light-emitting and energy-harvesting devices due to their non-toxic nature, tunable band gap, environmental stability, and water solubility. [1]However, intrinsic defects such as cation and anion vacancies, interstitials, and antisites significantly control their optical properties. Here, we synthesize and study AgInS₂ QDs that exhibit two emissions: a relatively narrow but weak near the bandgap, and a more intense but broad and strongly red-shifted one. The former indicates free exciton emission and the latter defect related luminescence. Coating these QDs with gallium sulfide (GaSx) to produce AgInS2/GaSx core/shell structures leads to a significant suppression of the defect-related emission and great enhancement of the free-exciton luminescence. Using steady-state optical- and ultrafast transient absorption spectroscopy, we further investigate the absorption features of these core and core/shell QDs. Essentially, we find that the emissive defects in these QDs are primarily on the surface and diminishing them enables band-edge excitonic transitions. A comprehensive understanding of such surface defects is therefore crucial for yielding pure and efficient free-exciton emission in I-III-VI QDs.
1.2-I2
CuInS2 quantum dot (QD) has been considered as one of the choicest toxic-metal-free QDs.[1-3] CuInS2 QD exhibit large Stokes shift (145 nm /4265 cm-1 /530 meV) in the solution phase and the FWHM of its PL emission is quite significant (105 nm /2415 cm-1 /300 meV). Photoluminescence quantum yield (PLQY) of core-only CuInS2 QD is quite low (~15%). Although a few ultrafast exciton-dynamical (femtosecond-early nanosecond) investigations have been reported in this QD system,[4-6] however, there is no report of detailed exciton dynamical analyses to understand (a) low PLQY, (b) large magnitude of Stokes shift and (c) large magnitude of FWHM of PL emission. Moreover, although the excited state lifetime of this QD is quite long (~4 microsecond), however, there is no detailed investigation of exciton dynamics from nanosecond to microsecond time domain. Temperature (4K to 300K) dependent steady-state PL measurement exhibits an emissive state just below the band edge. All these detailed steady-state and dynamical investigations and analyses clearly reveal the reasons behind (a) low PLQY, (b) large magnitudes Stokes shift, and (c) large FWHM of PL emission.
Upon alloy-shelling of this core CuInS2 QD, the PLQY of the core/alloy-shell (CAS) CuInS2 QD increases enormously and a near unity PLQY (0.96) could be achieved.[7] More interestingly, such high PLQY of CAS CuInS2 QD remains nearly stable for at least one year.[7] Ultrasensitive single particle spectroscopic investigation with such highly luminescent and stable QD reveal highly suppressed blinking (>80% ON fraction).[7] Interestingly, exciton trapping and detrapping rates and their ratios have been observed to be dependent on excitation energy/wavelength.[8] To the best of our knowledge, so far this is the only QD system in which very significant amplitude variations of both hole trapping and detrapping have been observed.[8] Unlike electron trapping, hole trapping in this CAS CuInS2 QD has been shown to be beneficial.[8] Simultaneous electron and hole trapping have been shown to be leading to very long ON time (130 s) without blinking, which is so far the highest for a toxic-metal-free QD.[8]
All these detailed dynamical analyses and the results will be elaborated.
1.3-I1
Ternary chalcopyrite quantum dots, such as AgInS2 (AIS) or CuInS2 (CIS), as well as quaternary kesterite quantum dots (QDs), reveal unique properties with no analogs in the realm of conventional binary AIIBVI QDs, in particular, high tolerance to compositional non-stoichiometry, doping and alloying, as well as remarkable composition- and size-dependent spectral characteristics, photoluminescence (PL) mechanisms, charge carrier dynamics, etc. The chalcopyrite CIS and AIS QDs revealed volcano-shaped dependencies of the efficiency of PL emission and interfacial charge on their composition, with the highest values reached far from stoichiometry, at In:Ag(Cu) ratios of 4-5, highlighting a unique potential of such non-stoichiometric QDs. The realization of this potential crucially depends on the availability and versatility of synthetic approaches, allowing the QD composition, size, surface chemistry, and ligand shell structure to be tailored to specific light harvesting/conversion applications. The present talk shows that conventional heating-up/hot injection syntheses in high-boiling-point coordinating solvents can be successfully rivaled by mild and relatively “green” approaches of direct synthesis and size-tuning of ternary/quaternary QDs in aqueous solutions.
Aqueous approaches proved to be universal and applicable to many ternary chalcopyrite QDs, such as CIS, AIS, and CAIS, as well as to quaternary kesterite QDs, in particular Cu(Ag)2ZnSnS4. These approaches allow the QD composition to be varied in a broad range (for example, In(Sn):Ag(Cu) ratio variable between 1 and 20), protecting QDs with various ligands (mercaptocarboxylic acids, multi-functional ligands such as glutathione or polymers, such as polyethylene imine), and covering QDs with various protective shells (ZnS, CdS, In2S3, etc.). When combined with fine size-selective precipitation and post-synthesis solvent transfer, the aqueous approaches deliver unprecedented synthetic flexibility and variability of QD composition and size, allowing the aqueous synthesis to be upscaled to a combinatorial high-throughput robot-assisted regime. The latter is capable of yielding hundreds of samples with different spectral characteristics and PL dynamics within the same synthetic protocol, exemplified in the present talk by a high-throughput PL study of core AIS and core/shell AIS/ZnS QDs.
The combinatorial potential of the aqueous synthesis of ternary/quaternary QDs can be further expanded by their unique tendency to spontaneous alloying, which results in more complex multinary chalcogenide QDs and allows more sophisticated combinatorial synthesis to be performed with several types of ternary QDs used as sacrificial precursors. This approach is exemplified by a high-throughput robot-assisted synthesis of non-stoichiometric chalcopyrite CuAg-In-SSe (CAISSe) QDs from individual CIS, AIS, CISe, and AISe QDs as precursors. The CAISSe QDs revealed unexpected volcano-shaped dependences of PL intensity and lifetime on both Cu:Ag and S:Se ratios, advocating a large potential of the high-throughput screening of aqueous QDs for the discovery of new materials with advanced functionalities.
The broad range of instruments for composition and size variation of multinary QDs available in aqueous syntheses, including precise size selection, spontaneous alloying of QDs, as well as various cation, ligand, and solvent exchanges are currently incorporated into a unified concept of high-throughput robot-assisted screening capable of delivering tens of thousands of different QD species to be tested as light emitters and photovoltaic absorber materials. The present talk highlights our developing strategy of combining high-throughput aqueous syntheses with accelerated characterization and machine-learning-assisted analysis of QD properties as functions of their composition and size. The latter is expected to provide meaningful feedback to select new compositions and steer the synthesis toward the desired QD properties, closing the “synthesis-characterization-analysis” loop and enabling automated high-throughput material discovery within the domain of multinary metal-chalcogenide QDs.
1.3-I2
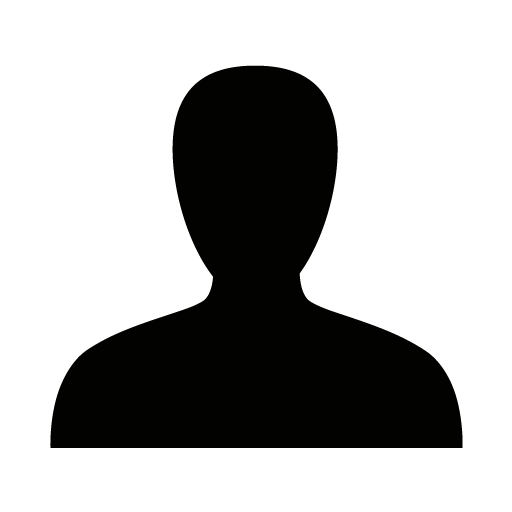
The interest in CuInS2 (CIS) quantum dots (QDs) has increased significantly in the past few years. CIS QDs have been studied for many applications like photodynamic therapy or solar cells[1]. They show exciting optoelectronic properties, such as broad photoluminescence (PL) with a large Stokes shift and long charge carrier lifetimes. Several mechanisms for the radiative recombination in CIS QDs have been proposed.
The most popular explanation is that radiative recombination results from an electron in the conduction band and a hole in a so-called confined hole state (CHS) related to Cu [2-3]. The range of such possible states would explain the broad PL and large Stokes shift. In addition, different synthetic characteristics, such as Cu:In stoichiometry, Zn doping or the passivation of the QDs can greatly affect the photophysical properties including the formation process of the CHS [4], as well as the structure.
X-ray techniques can be very useful tools to explore these kinds of systems from a new angle. X-ray absorption and emission spectroscopies provide information with element and oxidation state specificity, which can be useful to observe processes such as charge localization and transfer. These can be complemented with X-ray diffraction to have a more general view of the structure.
With the development of X-ray free electron lasers (XFELs), these techniques have become available in the ultrafast time-domain, allowing us to incorporate them in the study of the photophysics in many systems.
We will review our recent results obtained through a combination of laser, synchrotron and XFEL techniques. We focused on following the oxidation state of Cu through time-resolved Cu K-edge XANES and comparing the structure of the different samples through steady-state XANES and EXAFS at the Cu, Zn and S K-edges. This allowed us to gain insights into the structure, the surface passivation and the Zn incorporation through different synthetic routes. This was then complemented by optical studies [5].
1.3-O1
Kaifeng Wu obtained his B.S. degree in materials physics from University of Science and Technology of China (2010) and his PhD degree in physical chemistry from Emory University (2015). After his postdoc training at Los Alamos National Laboratory, he moved to China to start his independent research in 2017. His current work focuses on the ultrafast spectroscopy of carrier and spin dynamics in low-dimensional optoelectronic materials, as well as relevant applications in quantum information and energy conversion technologies. He is the winner of the 2022 Distinguished Lectureship Award by the Chemical Society of Japan, 2021 Future of Chemical Physics Lectureship Award by the American Physical Society, 2020 Chinese Chemical Society Prize for Young Scientists, 2019 Robin Hochstrasser Young Investigator Award by the Chemical Physics journal, and 2018 Victor K. LaMer Award by the American Chemical Society. He also serves as the Editorial Advisory Board of J. Phys. Chem. Lett.
Near-infrared to visible photon upconversion holds great promise for a diverse range of applications. Current photosensitizers for triplet-fusion upconversion across this spectral window often contain either precious or toxic elements, and have relatively low efficiencies. Although colloidal semicondcutor nanocrystals have emerged as versatile photosensitizers, the only family of nanocrystals discovered for near-infrared upconversion is the highly-toxic lead chalcogenides. Here we report zinc-doped CuInSe2 nanocrystals as a low-cost and lead-free alternate, allowing for near-infrared to yellow upconversion with an external quantum efficiency reaching 16.7%. When directly merged with photoredox catalysis, this system enables efficient near-infrared-driven organic synthesis and polymerization, which in turn solves the issue of photon reabsorption loss for nanocrystal-sensitized upconversion. Moreover, the broadband light capturing of these nanocrystals allows for very rapid reactions under indoor sunlight. Extending the reach of "solar synthesis" into the near-infrared may realize the century-long dream of conducting high added-value chemical transformations using sunlight.
1.3-I3
Maksym Yarema received his master degree in Chemistry from Lviv National University (Ukraine) in 2007. From 2008 to 2012, he worked towards his doctorate degree at the Johannes Kepler University Linz (Austria) under supervision of Prof. W. Heiss. In 2012, he joined the research group of Prof. M. V. Kovalenko at EMPA as Marie-Curie fellow. Since 2013, he is working in the Institute for Electronics, ETH Zurich (the research group of Prof. V. Wood), where he received the SNSF Ambizione Fellowship in 2016 and the ERC Starting Grant in 2019. His research interest spans various topics of solid-state and physical chemistry as well as chemical engineering. Particular focus is given for colloidal nanomaterials, their synthetic approaches and applications into optoelectronic devices, memory cells, and lithium-ion batteries.
While most research on I-III-VI nanomaterials has focused on stoichiometric compositions with equimolar amounts of Group I (Cu, Ag) and Group III (In, Ga) metals, we have taken a different approach by developing Indium-rich I-III-VI colloids (>50 at.% Indium in the cationic sublattice). [1],[2] These materials, though non-stoichiometric, remain perfectly charge-balanced semiconductors due to the presence of cationic vacancies and long-range atomic ordering. Notably, Indium-rich I-III-VI compositions are go-to materials for thin-film photovoltaics, as they exhibit fewer defects in CIGS grains and at interfaces.
In this talk, we will explore the potential of Indium-rich I-III-VI quantum dots. In addition to their size-dependent properties, these non-stoichiometric colloids offer broad solubility ranges (i.e., tunable Group I to Group III ratios), enabling precise control over the optical bandgap and photoluminescence wavelength. [3] We will examine how atomic ordering influences these properties in a non-linear fashion, leading to particularly efficient photoluminescence at select compositions. [2],[4] We will also discuss a generalizable synthetic method for Indium-rich I-III-VI nanocrystals, [3] capable of batch-upscaling to >10 grams of size- and composition-uniform quantum dots in the laboratory settings. [5]
2.1-O1
Fluorescent nanothermometers are nanoscale materials that posses temperature dependent specrtoscopic properties. These nanostructures are expected to revolutionize research of cell functions and provide strategies for early diagnostics if nontoxic, stable materials allowing for accurate and precise temperature measurement can be fabricated. In this work, we study temperature-dependent photoluminescence (PL) properties of CuInS2/ZnS core/shell colloidal quantum dots (QDs) encapsulated in micelles for solubility in aqueous environments. We demonstrate four properties that can be used for temperature readout: (i) intensity quenching, (ii) PL decay acceleration, (iii) peak energy shift, and (iv) change in the excitation efficency ratio. We explain the physical mechanisms responsible for the four modes and demonstrate single mode nanothermometer performance. Crucially, using multiple linear regression (MLR), we combine the four modes into a single multiparameter readout mode. We unambiguously demonstrate that the MLR mode significantly boosts the nanothermometer performance. Namely, the sensitivities are increased by up to a factor of 7, while the precision is improved by a factor of 3. We discuss the implications of these results to other nanothermometer materials. Our results show that CuInS2/ZnS QDs are excellent nanomaterials for intracellular in vivo thermometry and provide guidelines for further optimization of their performance.
2.1-I1
Copper indium sulfide (CIS) QDs present a promising alternative to traditional Pb and Cd based quantum dots [1] due to their low toxicity, photostability, and tunable optical properties. CIS-QDs can crystallize in chalcopyrite or zinc blende-like structures, allowing for compositional tuning and doping, which enhances stability and functionality. Recent efforts have focused on aqueous synthesis using water-soluble stabilizers to improve their colloidal stability and applicability in bioimaging.[2]
Our work involves the hydrothermal synthesis of manganese-doped Cu-In-Zn-S (Mn-CIZS) QDs, which exhibit dual emission from Mn²⁺ (~ms lifetime) and the CIZS host (~μs lifetime). Mn²⁺ emission depends on the host composition and involves an intermediate energy state. Mn-CIZS-QDs also exhibit stable, reversible temperature sensitivity under physiological conditions, making them promising as water-soluble luminescent temperature probes.[3]
The second topic of our work is related to hybrid systems of azobenzene-functionalized CIS QDs. These systems demonstrate quantitative photoisomerization to the (Z)-isomer under UV-visible light (from 365 nm to 533 nm) without back photoisomerization. This unique behavior is attributed to the direct interaction between the (Z)-isomer and CIS QDs, a novel light-induced ligand exchange mechanism, where photoactive azobenzene derivatives repleace ligands on the CIS surface promoting functionalization upon light irradiation.[4]
2.1-I2
Teresa Pellegrino since 2014 is tenured team leader of the group of “Nanomaterials for Biomedical Applications” at the Italian Institute of Technology, Genoa, Italy. She received her PhD in Chemical synthesis in 2005 from the University of Bari. Her current research interests focus on the development of inorganic nanostructures for drug delivery, magnetic hyperthermia, photo-thermal treatment and radiotherapy applications. She is coauthor of 150 papers in peer review international journals. She is the recipient of H2020 ERC Consolidator grant “ Magnetic Hyperthermia for Metastasized Tumor Treatment and Remote Manipulation of Microdevice -GIULIa” N. 101044020 and the Investigator Grant received by the Italian Association of Cancer Research IG-AIRC-2023’ entitled ‘Magnetic scaffolds for tumor treatment: combining immunotherapy, differentiation therapy and mild magnetic hyperthermia’
Semiconductor nanocrystals, also known as quantum dots, given their robust photoluminescent properties, single source excitation and multicolor emission properties have been employed for multiplexing and long-term imaging studies. [1] On the other hand, cation exchange (CE) reactions consisting of the replacement of cations in the nanocrystalline structure with different metal ions while maintaining in place the anion framework of th nanocrystals, have been extensively used for the synthesis of nanocrystals at different compositions. Here, we exploit CE reactions to radiolabel cadmium-free semiconductor NCs of ZnS, ZnSe and chalcopyrite (CuFeS2) NCs with Cu-64 radioisotope. [2] We have developed a one-step CE protocol that is straightforward and highly efficient and by tuning the type of ligand coating to be chosen as water soluble stabilizer agents we kept the NC colloidal stability. The amount of Copper-64 to be exchanged could be also tuned in a controlled manner from partial to 100 % cation exchange. This unique approach of CE reaction enables to tune the specific activity in a wide range (from 2 to 100 TBq/g ) with an unprecedentedly record value of specific activity up to 100 TBq/g. This protocol also enables to obtaining 64Cu:CuFeS2 with high radiolabeling yield which do not require any further work out for the purification thus speeding up the radiolabeled NCs preparation. In addition, among the NCs explored, CuFeS2 NCs even after partial-CE reaction with Copper-64 preserve a plasmonic resonance band, which peaks in the near infrared region making them promising NCs for photo-thermal therapy (PPT). The synergic toxicity of photo-hyperthermia and 64Cu mediated radiotherapy ionization here is used to prove the damage to glioblastoma and epidermoid carcinoma tumor cells as demonstrated in vitro on cell culture model. A modified version of this protocol has been also established to obtain sub nanometer copper-64 radio-clusters possessing also radio and photoluminescent properties. Finally, we have also extended the same CE reaction concept to radiolabel lanthanide-based nanoparticles (NPs), consisting of NaLnF4 composition (Ln= Gd, Lu) with Yttrium 90 (90Y ). Throughout this presentation, for the best performing materials, preclinical results to evaluate therapeutic efficacy and bio-distribution will be also discussed.
2.1-S1
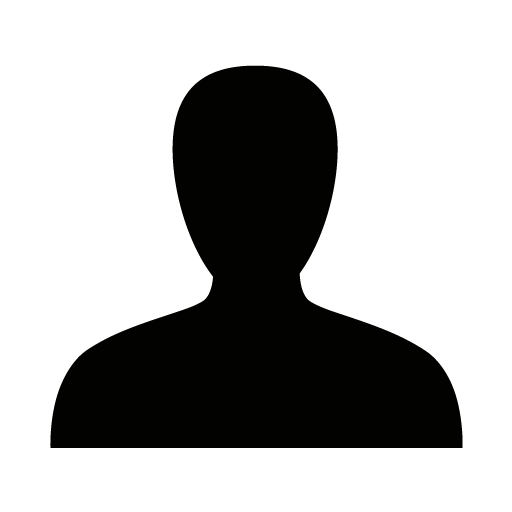
A short introduction to the RSC’s portfolio covering the breadth of energy, materials, and catalysis, with particular focus on our nanoscience journals, Nanoscale and Nanoscale Horizons.
1.1-I1
Bio Professional Preparation M.S. in Chemistry, with Honours, University of Bari, Italy, 1996 Ph.D. in Chemistry, University of Bari, Italy, 2001 Research interests Prof. L. Manna is an expert of synthesis and assembly of colloidal nanocrystals. His research interests span the advanced synthesis, structural characterization and assembly of inorganic nanostructures for applications in energy-related areas, in photonics, electronics and biology.
Halide perovskite semiconductors can merge the highly efficient operational principles of conventional inorganic semiconductors with the low‑temperature solution processability of emerging organic and hybrid materials, offering a promising route towards cheaply generating electricity as well as light. Following a surge of interest in this class of materials, research on colloidal halide perovskite nanocrystals (NCs) has gathered momentum in the last decade. This talk will highlight several findings of our group on their synthesis, for example our recent study on the influence of various exogenous cations and of acid-based equilibria on the growth of perovskite NCs, which can lead to the formation of NCs with peculiar shapes (for example hollow structures) and to NC heterostructures (for example CsPbBr3/PbS heterostructures) by promoting/suppressing the heterogenous nucleation of selected materials. I will also discuss our findings on the ordering of NCs in superstructures, and how cryogenic temperatures can influence the degree of ordering.
1.1-I2
While fluoride nanocrystals (e.g., NaYF4) are popular host materials for lanthanide-based up-and downconversion, ceramic oxide hosts (e.g., ZrO2 and HfO2) have found less widespread use, due to the synthetic challenge of producing colloidally stable oxide nanocrystals with a complex (e.g., core/shell) architecture. The oxides are however, more chemically and thermally stable.
We first present the results of our mechanistic investigation in the synthesis of zirconium and hafnium oxide from metal chloride and metal alkoxide in trioctylphosphine oxide (TOPO). We study the metal speciation in solution, we determined the decomposition kinetics and its mechanism and we study the nucleation and growth. We find evidence for an E1 elimination mechanism and the occurrence of amorphous particles as intermediate on route the final highly crystalline particles. This is a consequence of the rate imbalance between a fast precursor decomposition and a slow crystallization process.
Second, we demonstrate the epitaxial growth of hafnia shells onto zir-conia cores, and pure zirconia shells onto europium doped zirconia cores. The core/shell structures are fully crystalline. Upon shelling, the optical properties of the europium dopant are dramatically improved (featuring a more uniform coordination and a longer photoluminescence lifetime), indicating the suppression of non-radiative pathways.
Third, we dive deeper in lanthanide doped zirconia nanocrystals. We determine the incorporation efficiency of multiple lanthanide in zirconia. We show the influence of the surface chemistry on the emission spectrum and lifetimes of Eu3+ of Tb3+. Time resolved emission spectra allow us to differentiate different europium sites (surface and bulk).
These results launch the stable zirconium and hafnium oxide hosts as alternatives for the established NaYF4 systems.
1.1-O1
Colloidal lead halide perovskites (LHP) nanocrystals (NCs) are popular light-emissive materials for optoelectronic devices, of interest for LEDs, LCDs, lasers and quantum light sources. Most studies on LHP NCs focus on relatively large NCs exceeding 10 nm in size, exhibiting weak to no quantum confinement effects. Recently, we showed that perovskite quantum dots (QDs) can be synthesized using a newly developed synthesis route, resulting in perovskite QDs that are tunable between 3 and 13 nm range.[1] Further exploring the tunability of these QDs, we extend the emission of these materials through doping, as well as by coupling these QDs to anchored luminescent dyes.[2,3] First, we demonstrate the synthesis of size-tunable spheroidal CsPbCl3:Mn2+ QDs, which can be obtained by a water–hexane interfacial combined anion and cation exchange strategy starting from CsPbBr3 QDs.[2] The size dependence observation of the manganese PL efficiency and the slow ET rate suggest that Mn2+ mainly gets incorporated at the QD’s surface, highlighting the importance of strategies chosen for the incorporation of Mn2+ into perovskite QDs.
In a second approach to tune and extend the perovskite QD´s emission, we combined the perovskite QDs with dye molecules, make hybrid QD-dye systems that exhibit efficient ET from the QDs to the dye molecules.[3] With most QDs, ET usually proceeds through Förster resonance energy transfer (FRET), which requires significant spectral overlap between the QD emission and dye absorbance, and large oscillator strengths of those transitions which severely limits the choice of suitable dyes. As the perovskite QDs do not require passivating inorganic shells for bright emission, we can attach dye molecules directly to their surface, making ET mechanisms beyond FRET accessible. With the CsPbBr3-ATTO610 QD-dye system we achieved efficient ET from CsPbBr3 QDs to dyes with dimethyl iminium binding groups. The close binding of dyes to the CsPbBr3 surface facilitates spatial wavefunction overlap, resulting in efficient ET from CsPbBr3 to dyes with bright emission from the dye molecules, even with minimal spectral overlap. With steady-state and time-resolved photoluminescence experiments, we show that the ET proceeds via the Dexter exchange-type mechanism which significantly improves the tuneability of such QD-dye systems, and opening avenues for QD-molecule hybrids in a wide range of such as lighting applications
1.2-I1
Study of Nanoscale Atomic Diffusion in Metal-Semiconductor Core-Shell Nanoparticles
Sharona Horta1, Seungho Lee1, Rhys Bunting1, Mario Palacios1, Jani Kotakoski2 & Maria Ibáñez1*
1Institute of Science and Technology Austria
2University of Vienna
Abstract
Core-shell nanocrystals have garnered significant attention due to their potential for multifunctionality and synergistic effects across various fields including electronics, biomedical applications, pharmaceuticals, optics, and catalysis. To take full advantage of the functionality of these multi-component nanoparticles, it is imperative to possess a comprehensive understanding of their dynamic structural behavior and stability when exposed to external stimuli.
In this study, we focus on metal-semiconductor nanoparticles, in particular Cu1-x Aux@PbS, and investigate the differences in their dynamic structural evolution depending on the core composition using in-situ high-resolution transmission electron microscopy during heating. Our findings reveal that these nanoparticles transform from core-shell to Janus structure via the diffusion of core metal atoms towards the nanoparticle surface. The diffusion of the core atoms can occur either collectively or individually depending on the copper content within. To understand these differences, density functional theory (DFT) calculations were employed. The DFT calculations indicate that the diffusion mechanism is determined by the competition between the surface energy of the core and the interface energy between the core and shell. When the interface energy value is larger, the collective movement of the core atoms is favored, whereas when surface energy predominates, atomic diffusion becomes more favorable. Furthermore, strain mapping of this particle unveils significant changes in interface strain as temperature changes and core diffuses in a manner that ultimately minimizes overall strain. The insights obtained from these results contribute to the advancement of understanding diffusion at the nanoscale and the dynamic behavior of strain with temperature at the nanoscale.
1.2-I2
Debora Pierucci is a CNRS researcher at Sorbonne University, France. She earned her Ph.D. from Université Pierre et Marie Curie in 2013. Her research focuses on exploring the electronic properties of heterostructures composed of low-dimensional materials, using advanced photoemission techniques.
Colloidal nanocrystals (NCs), with their tunable optical properties, have emerged as essential components in modern optoelectronic devices [1]. While traditional optimization approaches focus on pristine material properties, they often neglect the influence of the complex operational environment, where NCs interact with transport layers, electrodes, and applied electric fields. This gap highlights the need for advanced techniques capable of probing the electronic structure of devices in situ and operando.
In this talk, I will show how scanning photoemission microscopy [2] addresses this need, providing unique insights into the energy landscape of NC-based devices during operation with sub-micron spatial resolution [3]. By unveiling the whole device energy landscape, this technique reveals key parameters such as diode built-in potential and transistor lever arm. Beyond scalar information, the high spatial resolution of this technique enables access to the vectorial distribution of the electric field, uncovering the current pathways within the NC film [4].
This approach significantly advances our ability to study nanocrystal-based devices under realistic conditions, fostering a deeper understanding that can drive rational design and performance optimization.
1.2-O1
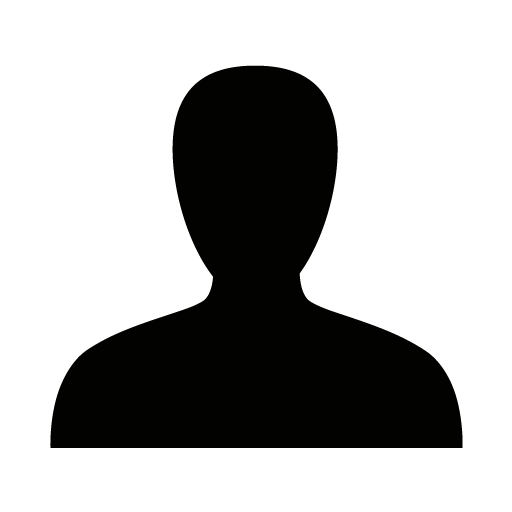
In the context of nanocrystal-based optoelectronic devices, the design of photodiodes faces a major challenge related to the design of building blocks as simple as the p-n junction that require some control on the carrier density profile. Gating appears as a possible alternative method [1] to shape the doping profile. The main limitations of the latter are related to the continuous application of DC bias, which increases energy consumption and can be a noise source.
In this work, we develop a general approach to achieve carrier density control by coupling the nanocrystal layer to a ferroelectric material. By utilizing the remnant polarization of the latter, the need for the application of gate bias is eliminated. The idea is to use the up and down change in the ferroelectric polarization to directly form a lateral p-n junction in the nanocrystal film, as has already been achieved for 2D materials.
We start by designing a ferroelectric-NC heterostructure made of Lead Zirconium Titanate (PZT) and HgTe NCs that act as an infrared active spin-coatable ink. The effect of the ferroelectric polarization on the HgTe band offsets is revealed using nano-beam X-ray photoemission microscopy. A photodiode is then built and enhanced photoresponse and reduced noise are obtained thanks to the built-in potential of the diode. [2] We then expand the concept to a large scale substrate using the commercially available periodically poled lithium niobate (PPLN) substrate. [3]
1.3-O1
How can boron-rich nanocrystalline films be optimized to meet the stringent mechanical demands of extreme environment applications?
Modern advances in clean energy, hypersonic travel, and nuclear technologies place extraordinary demands on materials' thermal and mechanical durability. High-stakes fields, such as aerospace and space exploration, require materials that withstand extreme conditions, often exceeding 4,000 °C, with substantial mechanical strength and oxidation resistance. Refractory materials like ultra-high temperature ceramics (UHTCs), while promising, are limited by high production costs and challenging synthesis processes. This study seeks to address this challenge by exploring nanoscale metal boride materials—specifically, strontium hexaboride (SrB6) nanocrystals (NCs)—as a cost-effective, mechanically robust alternative.
Nanocrystals (NCs) offer unique advantages due to their high surface area, tunable crystallization, and the ability to form films with nanoscale precision, which is critical for enhancing mechanical properties in thin coatings. Here, we investigate the potential of surface-modified SrB6 NCs, blade-coated onto silicon and sapphire substrates, as a pioneering solution for boron-rich, super-hard thin films. Through ligand modification with BF4 and BI3, these NCs achieve distinct structural formations on different substrates, significantly impacting their mechanical performance.
Our findings demonstrate that SrB6-BI3 films on silicon reach up to 10 GPa hardness and a Young's modulus between 180 and 200 GPa. In comparison, SrB6-BF4 films attain 5 GPa hardness and 170 GPa modulus on silicon, with a notably higher modulus of 300 GPa on sapphire, suggesting enhanced stiffness through substrate optimization. Atomic force microscopy (AFM) revealed crystallization patterns where SrB6-BI3 formed micron-sized crystals on silicon, while SrB6-BF4 created spherical clusters, further affecting mechanical properties.
This study highlights that by optimizing ligand choice, substrate selection, and minimizing defects, boron-rich metal boride nanomaterials can be tailored for demanding applications. These findings position SrB6 NC-based films as a promising, cost-efficient alternative to conventional super-hard materials like diamond, with potential breakthroughs in extreme environment applications.
1.3-O2
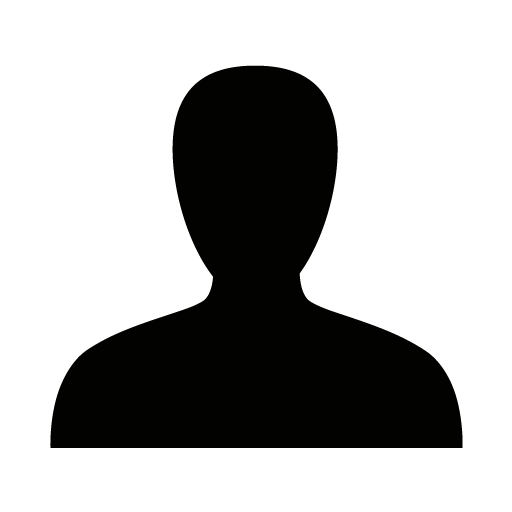
The recent emergence of quantum confined nanomaterials in the field of radiation detection [1], in particular lead halide perovskite nanocrystals, offers scalability [2] and performance advantages [3] over conventional materials. This development raises fundamental questions about the mechanism of scintillation itself at the nanoscale and the role of particle size, arguably the most defining parameter of quantum dots. Understanding this is crucial for the design and optimisation of future nanotechnology scintillators.
In this work, we address these open questions by theoretically and experimentally studying the size-dependent scintillation of CsPbBr3 nanocrystals using a combination of Monte Carlo simulations, spectroscopic, and radiometric techniques. The results show that the simultaneous effects of size-dependent energy deposition, (multi-)exciton population, and light emission under ionizing excitation, typical of confined particles, combine to maximize the scintillation efficiency and time performance of larger nanocrystals due to greater stopping power and reduced Auger decay.
The agreement between theory and experiment produces a fully validated descriptive model that predicts the scintillation yield and kinetics of nanocrystals without free parameters, providing fundamental guiding for the rational design of nanoscale scintillators.
1.3-I1
Perovskite nanocrystals (NCs) are emerging as promising materials for next-generation light-emitting diodes (LEDs) and scintillators, offering significant potential for optoelectronics and radiation detection [1,2]. This presentation explores recent advancements and challenges in developing perovskite-based materials for these applications. We focus on Ruddlesden–Popper (RP) interfaces in mixed halide perovskites (MHPs), which play a critical role in optimizing device performance [1,2]. Using aberration-corrected high-angle annular dark-field scanning transmission electron microscopy, we examine atomic-level defects and strain at these interfaces, revealing how halide migration affects the structural integrity and electroluminescence of MHP-based devices. In addition to lead halide perovskite NCs, we explore the growing interest in low-dimensional, lead-free copper halide perovskite (CHP) NCs for scintillation applications [3]. By employing mechanochemical methods, we synthesize 1D and 0D CHP NCs and investigate their scintillation properties, including decay times and Stokes shifts. Our findings show that tuning the I:Br composition ratio enhances scintillation performance, providing insights into the design of fast-decaying, ultrasensitive X-ray detectors. The presentation will also highlight ongoing research into nanophotonic structures, such as photonic crystals and plasmonic structures, that enhance light emission via Purcell effects, offering the potential for faster, brighter LEDs and scintillators in future technologies [4].
1.3-O3
In recent decades, lead halide perovskite nanocrystals (PNCs) have emerged as promising high-atomic-number scintillating materials for next-generation scintillators and photodetectors in ionizing radiation detection. Their strong light-matter interaction, tunable radioluminescence, and solution processability position them as key candidates for advanced applications. However, preserving their luminescence while ensuring environmental stability remains a challenge. Innovative strategies for embedding PNCs in impermeable host matrices have been developed [1], These approaches preserve the luminescence properties of the NCs in harsh environments and also prevent Pb dispersion, thus enabling their safe use in biological applications. On the one hand, study reveals the crucial parameters in the design of scintillation detector on isolated NC level [2] Here, we explore innovative embedding strategies using mesoporous silica to enhance PNC stability and performance. By spatially engineering PNCs, we can manipulate their collective scintillating behaavior, leading to cascading scintillation effects while maintaining a low overall lead content. Also, we propose a design of bismuth-loaded PNC plastic scintillator for gamma and neutron detection. Given that bismuth is known to quench the photoluminescence (PL) of perovskites instantaneously, to the best of our knowledge, our work is the first to successfully integrate perovskites with bismuth in a scintillation system, demonstrating a novel approach that overcomes the challenge and advancing perovskites’ potential for.broader radiation detection technologies.
1.3-I2
Colloidal coupled quantum dots molecules (CQDMs) were recently synthesized by “nano-chemistry”.[1-3] These CQDMs, made by fusing two CdSe/CdS core/shell quantum dots, uniquely consist of two coupled emitting centers. Such CQDMs raise significant fundamental interest as they manifest optical properties differing from those of the constituent quantum dots, which has been taken as a signature of electronic coupling, akin to that in molecules constructed from real atoms.
Recent theoretical studies, however, indicate that the distinct behavior of CQDMs arises mainly from relaxed exciton confinement, rather than molecular-like electronic coupling.[4] The reason is that CQDMs are designed to favor electron tunneling between the dots, but holes remain strongly localized inside the core of the individual dots. Consequently, upon photoexcitation, holes capture electrons and prevent them from tunnel-coupling. The same holds when CQDMs are populated with biexcitons (forming an artificial H2 molecule).[5]
Herein, we present a strategy to restore electronic coupling in CQDMs. By means of k·p simulations, we show that using trions (charged excitons) instead of neutral excitonic complexes, the ground state of the CQDM acquires strong molecular (bonding) character. This is because uncompensated Coulomb repulsions prevent carriers from localizing in individual dots. Positive trions (an artificial H2+ molecule) are found to be particularly apt for the practical realization of CQDMs with substantial electronic coupling.
2.1-O1
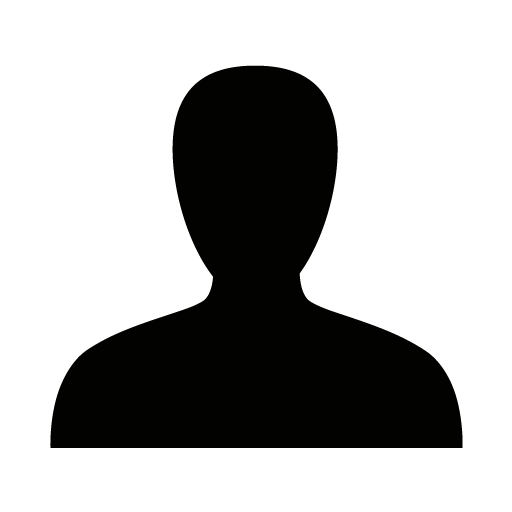
Quantum dots (QDs) as scintillators for radiation detection have garnered significant attention due to their unique photophysical properties. Previous studies have shown nanocrystal scintillators for X-rays, gamma, neutrons, and electrons[1][2][3][4]. The European Pathfinder project Unicorn proposes to develop novel nano-scintillators for detection of neutrinoless double beta decay(0νββ) for the first time. 0νββ, a rare nuclear process, is at the frontier of neutrino physics, offering insight into the fundamental nature of neutrinos. Cadmium isotopes, such as 116Cd, are promising candidates for such studies due to their favorable properties, including high natural abundance, a large Qββ value, and potential for inverse beta decay detection[5]. In this work, we developed a novel scintillator Cu-doped CdZnS/ZnS QDs for this application. This study presents a comprehensive investigation of the fundamental optical properties and scintillation dynamics of Cu-doped CdZnS/ZnS QDs. The results demonstrate that these QDs exhibit high quantum efficiency, ensuring a strong light yield. Additionally, their large Stokes shift effectively suppresses reabsorption, a critical feature for high-loading and large-volume detectors. Furthermore, we evaluated the performance of this material in gamma spectroscopy highlighting its potential as a scintillator for gamma detection, compared to conventional plastic scintillators. This work represents a step forward in the development of advanced scintillators for neutrino physics and rare event detection technologies.
2.1-I1
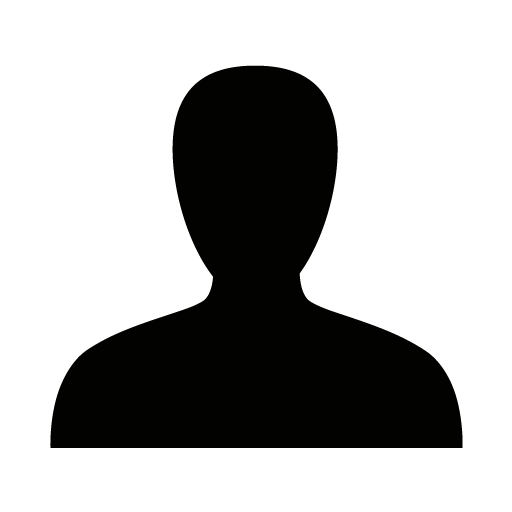
Nanostructured semiconductors dominate opto-electronics to date, mainly in the framework of quantum wells and dots grown by vacuum epitaxial methods. A next (r)evolution in this field is happening as we speak through the use of solution processable nanostructured materials. Indeed, by combining low temperature, substrate independent processing with size-tunable optical properties, such as luminescence and optical amplification (gain), these ‘nanocrystals’ are excellent building blocks to realize small footprint LEDs and lasers. In this talk, I will overview our efforts towards integrated laser sources based on colloidal nanocrystals. To do so, I will start out with discussing how optical gain, a premise to build lasers, is measured and quantified in these materials. Building on this framework, I will explain how net stimulated emission develops in and how we reached after nearly a decade of research a set of materials with excellent gain metrics on all fronts using so-called ‘bulk nanocrystals’ as a new design route. Next, I will show a few results of combining these flexible materials with integrated photonic cavities, thereby realizing ultra-small footprint lasers operating under quasi-CW pumping. Finally, I will present a route forward to transition these RGB lasers into the infrared spectrum where a select pick of ultrafast mid-infrared spectroscopy experiments gives a first flavor of the challenges , but also massive opportunities, lying ahead in the ongoing ERC project ‘NOMISS’.
2.1-I2
Kaifeng Wu obtained his B.S. degree in materials physics from University of Science and Technology of China (2010) and his PhD degree in physical chemistry from Emory University (2015). After his postdoc training at Los Alamos National Laboratory, he moved to China to start his independent research in 2017. His current work focuses on the ultrafast spectroscopy of carrier and spin dynamics in low-dimensional optoelectronic materials, as well as relevant applications in quantum information and energy conversion technologies. He is the winner of the 2022 Distinguished Lectureship Award by the Chemical Society of Japan, 2021 Future of Chemical Physics Lectureship Award by the American Physical Society, 2020 Chinese Chemical Society Prize for Young Scientists, 2019 Robin Hochstrasser Young Investigator Award by the Chemical Physics journal, and 2018 Victor K. LaMer Award by the American Chemical Society. He also serves as the Editorial Advisory Board of J. Phys. Chem. Lett.
The interconversion between singlet and triplet spin states of photogenerated radical pairs is a genuine quantum process, which can be harnessed to coherently manipulate the recombination products through a magnetic field. This control is central to such diverse fields as molecular optoelectronics, quantum sensing, quantum biology and spin chemistry, but its effect is typically fairly weak in pure molecular systems. Here we introduce hybrid radical pairs constructed from semiconductor quantum dots (QDs) and organic molecules. The large g-factor difference allows us to directly observe the radical-pair spin quantum beats usually hidden in previous studies, which are further accelerated by the strong exchange coupling of the radical pairs enabled by quantum confinement of QDs. The rapid quantum beats enable efficient and coherent control of charge recombination dynamics at room temperature, with the modulation level of the yield of spin-triplet products reaching 400%. As spin-triplets are ubiquitously involved in molecular and hybrid inorganic/organic photocatalytic and photonic devices, an efficient, quantum-coherent control over the triplet recombination yields of radical pairs could offer disruptive solutions to engineering the performance of such devices. Additionally, hybrid radical pairs constitute a unique material platform to merge the field of emerging molecular quantum sciences with solid-state quantum platforms to enable novel physical phenomena and quantum technologies.
2.1-O2
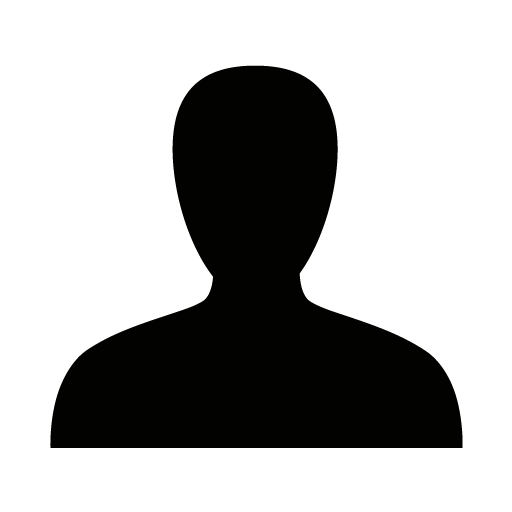
The current spectral coverage of colloidal quantum dot (CQD) lasers spans from the visible, using zinc and cadmium chalcogenide-based quantum dots, all the way through to the short-wave infrared (SWIR) region, up until telecoms wavelengths using lead sulphide (PbS). However, the full spectral tuneable range of the PbS quantum confinement (up to 2.5 μm) has yet to be explored. The so-called extended SWIR region (1.7 μm – 2.5 μm), has many applications such as in LIDAR, biological imaging and environmental monitoring and is currently served only by exotic and costly materials with limited-scalability. We extend the gain coverage of PbS CQDs up until 2500 nm and report lasing with emission tuned between 2150 nm and 2500 nm using distributed feedback cavities. A detailed size-dependent study of the gain behaviour of these PbS CQDs is performed, investigating the absorption cross-section and excited state dynamics of the CQDs. The optical gain threshold of the largest dots emitting in the extended SWIR is seen to reduce by a factor of 36 compared to smaller-sized CQDs emitting at telecoms, reaching an amplified spontaneous emission (ASE) threshold down to 42 µJ/cm2. From this study we were then able to predict and realise for the first time, optical gain and lasing from PbS CQDs under nanosecond pumping. This major milestone paves the way for the realization of compact and practical CQD infrared lasers in the extended SWIR region and potentially towards electrically driven laser diodes.
2.2-O1
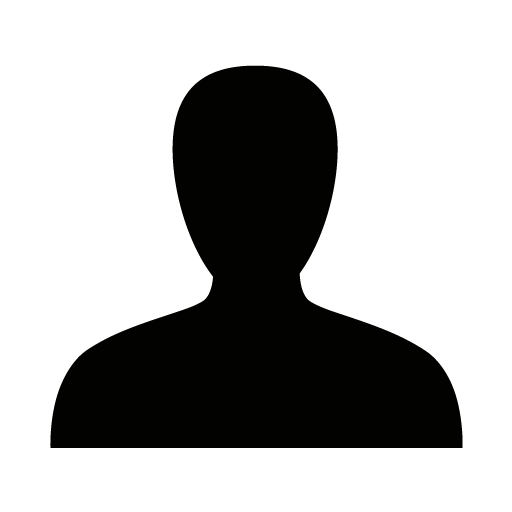
Colloidal quantum dots (QDs) show great promise as active gain materials for integrated photonics due to their solution processability. Unlike conventional semiconductors, colloidal QD layers can be directly deposited on various substrates using simple coating techniques such as spin coating, inkjet printing, or drop-casting. Recent advances in QD research have demonstrated their utility as low-threshold laser materials with remarkable robustness and stability.1,2 In this study, we utilized so-called type-(I+II) QDs2 to demonstrate well-behaved microdisk lasers.
Type-(I+II) QDs were recently introduced in the context of research into liquid QD lasing.2,3 They feature large optical-gain cross-sections and long optical gain lifetimes of ~3 ns. The latter property stems from a novel optical gain mechanism in which optical gain species are hybrid direct/indirect biexcitons with slow, trion-like Auger recombination. Due to their excellent optical-gain properties, type-(I+II) QDs are well suited for implementing both liquid- and solid-state lasers, including laser diodes.
To prepare microdisk lasers, we use standard photolithography and dry etching techniques. When excited using short optical pulses, QD-based microlasers exhibit low-threshold whispering gallery mode lasing. We applied similar fabrication techniques to incorporate microring resonators into high-current density electroluminescent structures. The developed devices exhibit strong dual-band electroluminescence due to the band-edge (1S) and first excited (1P) electronic states. This indicates the realization of population inversion in the QD medium. The next step is to achieve a net positive overall gain by optimizing the design of our fully stacked devices to enhance the modal gain and, in parallel, reduce the parasitic optical loss. The expected result of this work will be an electrically excited microdisk laser.
2.2-I1
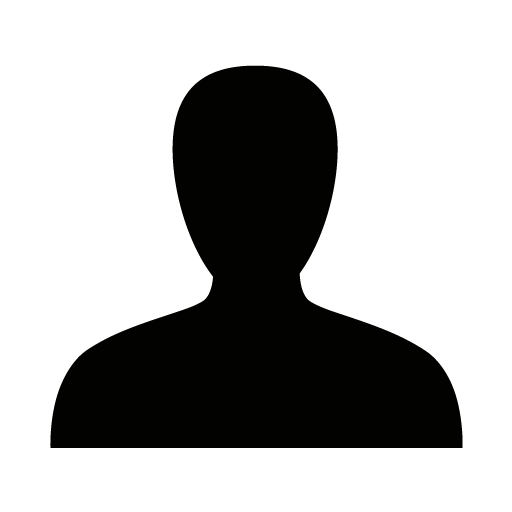
Artificial quantum systems with synthetic dimensions enable the exploration of novel quantum phenomena that are challenging to create in conventional materials. The synthetic degrees-of-freedom effectively increase the system's dimensionality without altering its physical structure, allowing for the study of higher-dimensional physics in lower-dimensional setups. However, synthetic quantum systems can suffer from intrinsic disorder, leading to rapid decoherence and limiting their scalability and collective coherence. This disorder-induced decoherence is a major obstacle in quantum information science and technology. Here we show that long-range interactions can mitigate decoherence and create persistent collective coherence preserved in highly symmetric collective excited states. These states exhibit exceptional robustness, capable of storing long-lived quantum memory despite disorder. We introduce an order parameter to quantify collective coherence, identifying a sharp transition from a zero to a non-zero collective coherence at a critical interaction strength or critical disorder. We denote this phenomenon as “supercoherence” and show its universality in a wide range of systems. This phenomenon not only challenges traditional views on the inevitability of decoherence in disordered quantum systems but also opens new avenues for harnessing collective coherence for quantum information storage and processing.
2.2-I2
In lead halide perovskites (APbX3), the effect of the A-site cation on optical and electronic properties has initially been thought to be marginal. Yet, evidence of beneficial effects on solar cell performance and light emission is accumulating. Here, we report that the A-cation in soft APbBr3 colloidal quantum dots (QDs) controls the phonon-induced localization of the exciton wavefunction. Insights from ab initio molecular dynamics and single-particle fluorescence spectroscopy demonstrate that anharmonic lattice vibrations and the resulting polymorphism act as an additional confinement potential. Avoiding the trade-off between single-photon purity and optical stability faced by downsizing conventional QDs into the strong confinement regime, dynamical phonon-induced confinement in large organic-inorganic perovskite QDs enables bright (106 photons/s), stable (>1h), and pure (>95%) single-photon emission in a widely tuneable spectral range (495-745 nm). Strong electron-phonon interaction in soft perovskite QDs provides an unconventional route toward the development of scalable room-temperature quantum light sources.
2.2-O2
Supercrystals represent three-dimensional orderings of colloidal nanocrystals (NCs), showcasing collective properties in photonics, phononics, and electronics applications.1,2 Recent studies have shown that such assemblies are directly produced during nanocrystal reactions.3–6 However, a fundamental understanding of in situ formed supercrystals that withstand typical NC purification processes remains underexplored, which is important for further use. Herein, we report the reaction precursor-mediated formation of stable PbTe supercrystals. Rationalizing the formation of these assemblies through small-angle x-ray scattering (SAXS) measurements, we unveil their formation mechanism. Our findings reveal that the supercrystal formation occurs in the presence of an excess of lead oleates in the crude solution. It should be noted that the formed supercrystals can be stabilized under specific conditions determined by the lead oleate cluster concentration, content of trioctylphosphine telluride (TOP-Te), NC size and the need of an annealing step at mild conditions. Furthermore, this approach allows for the continuous growth of a secondary phase within the supercrystal; for example in the case of PbTe supercrystals, a PbS shell can be grown on each PbTe NC constituent, resulting in core-shell PbTe-PbS supercrystals. Our work elucidates that reaction precursors play an important role in in situ SC formation and stabilization, implying the possibility of applying this knowledge to other NC reactions.
2.3-I1
Optical studies of colloidal nanocrystals (NCs) at cryogenic temperatures in high magnetic fields provide valuable information about their emission properties. Low temperatures (typically 1.7 – 4 K) are required to reduce the population of acoustic phonons and narrow the linewidth, and to reduce the population of the exited states. These conditions enable ensemble measurements. However, ensemble techniques generally give an “average” estimate of the underlying photophysics. Single NC spectroscopy, on the other hand, offers valuable additional insight into the properties of these materials, but has several disadvantages. First, the experimental complexity increases, and second, typically only NCs with bright and stable emission are selected for single-NC measurements, therefore, their properties not always represent the properties of all NCs in ensemble. Therefore, a combination of ensemble and single-NC measurements can provide a comprehensive understanding of the properties of these materials.
InP-based colloidal NCs are being developed as an alternative to cadmium-based materials. Since it is a relatively new material, understanding of their magneto-optical properties is still at an early stage. We have performed both ensemble and micro-photoluminescence measurements of InP/ZnSe/ZnS NCs [1]. The ensemble measurements revealed excitonic emission and provided information about hole ground state and exciton fine structure splitting. On a single-NC level, the trion emission has been discovered. The Zeeman splitting between the trion emission lines provided electron and hole Lande g-factors.
Colloidal CdSe-based nanoplatelets (NPLs) are quasi-two-dimensional nanocrystals with a strong quantum confinement in only one direction. They benefit from a lack of inhomogeneous broadening and have narrow and bright photoluminescence. To increase photostability of NPLs and enhance their quantum yield, it is desired to coat the CdSe core with a larger bandgap semiconductor shell. However, the shell growth leads to a large increase of the linewidth (>40 meV in core/shell NPLs versus ~10 meV in bare NPLs at cryogenic temperatures). Understanding of the broadening mechanisms is important, since narrow ensemble emission spectra are required in a lot of applications. We have demonstrated that at cryogenic temperatures the emission spectra of individual CdSe/CdZnS NPLs exhibit multiple isolated emission lines separated by several meVs spanning a range of 30 – 60 meV [2]. We believe that these features stem from trions localized in shallow (a few meV deep) traps. We have demonstrated that the population of occupied traps can be controlled optically.
2.3-I2
i
Group II-VI semiconductor nanoplatelets present narrow optical properties thanks to their thickness, which is the only confined direction, defined at the atomic scale without roughness. It makes them appealing for optoelectronic devices. Besides, in NPLs, the wide top and bottom facets are extended over 1000s of nm2. Thus, these facets can be assimilated to substrates for the self-assembly of molecules. At the end of the syntheses, the carboxylate ligands induce a tensile in-plane stress. These pristine ligands can be exchanged with X-type ligands, which can be halide, thiolate, phosphonate or chiral ligands. And, depending on their anchoring groups, the in-plane stress can vary from a tensile to a compressive stress, inducing thus a distortion of the crystal structure.
Here, we will first show that in CdSe NPLs capped with in-plane compressive stress thiolate ligands, Cd2+ are effectively exchanged by Cu+ cations, thus enabling the synthesis of Cu2-xSe NPLs with thicknesses comprise between 2 monolayers (MLs) and 5 MLs. Indeed, cation exchange is an interesting method to obtain nanoparticles which shape cannot be directly synthesized. In this case, the exchange most probably happens through the edges and its kinetic can be modified depending on the aliphatic chain length of the thiolate ligands. Such Cu2-xSe NPLs can be used as template for further cation exchange with Hg2+, leading to the synthesis of HgSe NPLs. However, the process is tedious and requires 2 consecutive steps including one in the glovebox. So we have demonstrated that a cation exchange from Cd2+ to Hg2+ could be co-catalyzed with Ag+ cations. Through this method, HgCdSe NPLs are obtained and enable to overcome the limitation of the direct cation exchange from Cd2+ to Hg2+ to only two cationic planes. The obtained NPLs are now showing a variation of the optical properties with quantum confinement.
Finally, I will show that pristine ligands can be exchanged with chiral ligands, in the aim of circular dichroism optical properties. We are showing a direct ligand exchange in ethanol from carboxylate to tartrate ligands, inducing the self-assembly of the tartrate ligands in two different conformations exhibiting opposite circular dichroism signal. The emergence of the circular dichroism optical properties comes from a good coupling of the ligands on the surface and an orthorhombic crystal distorsion. The dissymmetric factor can reach values as high as 1.2% for extended NPLs.
2.3-O1
Traditional infrared (IR) technology has relied on the epitaxial growth of CdHgTe (MCT) and InGaAs since 1960s.
While these epitaxial materials are well-established, they have not become cost-effective products for the consumer
market, limiting their applications. Solution-processed nanocrystals (NCs) exhibit tunable optical properties
spanning from the UV to THz spectra, making them suitable for applications such as single photon emission,
biolabeling, and down conversion for displays. Although the visible spectrum is commonly utilized, the potential of
NCs in the IR range has been largely overlooked. For the infrared spectral range, organic semiconductors are
intrinsically inefficient, leaving inorganic NCs as the most cost-effective and efficient option. Here, we present our
latest achievements in infrared light-emitting diodes (LEDs) and photodiodes (PDs), operating across various
wavelengths using tunable emissive colloidal materials. Our focus has been on CdHgSe nanoplatelets (NPLs) and
RoHS-compliant InAs quantum dots (QDs).
Mercury chalcogenides (eg. HgSe and HgTe) exhibit the most efficient emission in the near-IR to mid-IR range.
However, these materials are inherently fragile, making it extremely challenging to grow a shell over them. To
address this, we developed an innovative synthesis method starting with CdSe NPLs. We performed cation
exchange, replacing Cd with Hg, and subsequently grew a thick layer of CdZnS over the resulting CdxHg1-xSe NPLs.
This procedure allows fine tuning by adjusting the concentration of Hg cations and the stoichiometric ratio of Cd/Hg,
mirroring the epitaxial growth of MCT. Utilizing this tunable short-wave infrared (SWIR) emitting material, which
achieves a photoluminescence quantum yield (PLQY) of 55%, we designed and fabricated LEDs that emit at
wavelengths ranging from 1200 nm to 1700 nm. This builds on our previous work, where we achieved an external
quantum efficiency (EQE) of 7.5% at 1300 nm. [1] Here, we also showed the potential of using the same material as
the active layer of PD, with an EQE of 25% at 1200 nm wavelength.
In parallel, with an innovative synthesis of InAs/ZnSe core/shell QDs we increased the thickness of ZnSe thick shell.
Synthesizing InAs QDs has traditionally been a complex process, typically requiring the use of highly reactive,
flammable, toxic, and expensive chemicals such as tris-trimethylsilyl arsine (TMS-As). In recent years, researchers
have sought to replace TMS-As with cheaper, safer, and less reactive arsenic precursors. Among the alternatives
explored, tris(dimethylamino)-arsine (amino-As) has shown the most promise. Using amino-As along with Alane
N,N-dimethylethylamine as a reducing agent and ZnCl2 as an additive, we developed a method to synthesize InAs
QDs and InAs/ZnSe core/shell QDs with a shell thickness 1.5 monolayers. These QDs exhibit photoluminescence (PL) at 860 nm and a PLQY of 42% ± 4%.[2] Utilizing these QDs, we produced an LED with a turn-on voltage of 2.7V, an EQE of 5.5%, and a maximum radiance of 0.2 Wsr−1cm−2. [3] Building on these findings, we refined our synthesis
process to create InAs/ZnSe QDs with a tunable ZnSe shell thickness of up to 7 monolayers, achieving a remarkable
PLQY of approximately up to 70% ± 7% and a PL peak at 900 nm in solution. [4] The electronic structure of the thickshell QDs resembles that of type-I heterostructures, enhancing exciton confinement in the core region due to the ZnSe layer. We utilized these efficient QDs for making LEDs. The champion LED reaches an EQE of 13.3% and radiance of 12 Wsr−1cm−2, figures-of-merit that are comparable to devices based on complex core/multi-shell InAs QDs obtained via a tris-trimethylsilyl (TMS) arsine route. [5]
2.3-O2
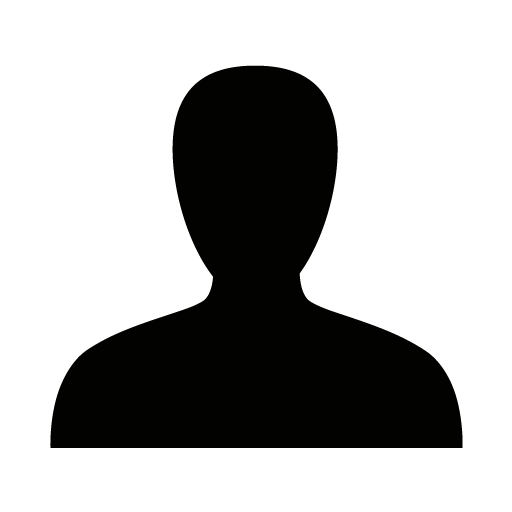
II-VI semiconductor nanoplatelets (NPLs) present optical features lacking of inhomogeneous broadening thanks to their 2D shape. Their thicknesses only present few atomic planes, such that any modifications of the surface chemistry induces a modification of their optical features. Recent studies have been dedicated to induce chiral light-matter interactions on these particles, to reach strong circular dichroism (CD) and circularly polarized luminescence (CPL) features, for example by grafting cysteine ligands on their surface.
Here, we propose to use chiral tartrate ligands [1]. Surprisingly, the exchange undergoes several stages, with an increase of the CD feature at the position of the heavy hole which red shifts over time followed by an inversion of the CD signal when the absorption saturates. The dissymmetry factor can reach values as high as 1.2 x 10-2. The peak inversion global aspect is influenced by the lateral aspect of the initial particle, the former surface chemistry, and the synthesis conditions.
This inversion is attributed to a mechanical relaxation of the ligand assembly that induces a different coupling between the inorganic core and the chiral ligands. This hypothesis is supported by surface chemistry characterization and XRD analysis.
1.2-I1
Perovskite-organic tandem solar cells (P-O TSCs) are developing rapidly, igniting tremendous interest in the multijunction community. However, their efficiency still lags behind that of other counterparts, and their current operational stability remains underexplored. This presentation will give a brief introduction into organic based multijunction solar cells and then turn over to hybrid multijunction devices. Two key aspect will be addressed specifically. First, we demonstrate a simplified interconnection layer (ICL) without an additional charge recombination layer (CRL), achieving an average efficiency of 25.12% (with a champion device reaching 25.53%) for P-O TSCs. Second, we present a detailed investigation to uncover how the single-junction sub-cells of multi-junction cells mutually stabilize each other, which leads to excellent long-term lifespan of P-O TSCs, retaining over 91% of their initial performance after 1000 hours of continuous metal-halide lamp illumination without UV filters. Besides, we also highlight the durability of ICLs, providing insights for advancing future tandem configurations.
1.2-I2
Thuc-Quyen Nguyen is a professor in the Center for Polymers and Organic Solids and the Chemistry & Biochemistry Department at University of California, Santa Barbara (UCSB). She received her Ph.D. degree in physical chemistry from the University of California, Los Angeles, in 2001 under the supervision of Professor Benjamin Schwartz. Her thesis focused on photophysics of conducting polymers. She was a research associate in the Department of Chemistry and the Nanocenter at Columbia University working with Professors Louis Brus and Colin Nuckolls on molecular self-assembly, nanoscale characterization and molecular electronics. She also spent time at IBM Research Center at T. J. Watson (Yorktown Heights, NY) working with Richard Martel and Phaedon Avouris. Her current research interests are structure-function-property relationships in organic semiconductors, sustainable semiconductors, doping in organic semiconductors, interfaces in optoelectronic devices, bioelectronics, and device physics of OPVs, photodetectors, and electrochemical transistors. Recognition for her research includes 2005 Office of Naval Research Young Investigator Award, 2006 NSF CAREER Award, 2007 Harold Plous Award, 2008 Camille Dreyfus Teacher Scholar Award, the 2009 Alfred Sloan Research Fellows, 2010 National Science Foundation American Competitiveness and Innovation Fellows, 2015 Alexander von Humboldt Senior Research Award, 2016 Fellow of the Royal Society of Chemistry, 2015-2019 World’s Most InfluentialScientific Minds; Top 1% Highly Cited Researchers in Materials Science by Thomson Reuters and Clarivate Analytics, 2019 Fellow of the American Association for the Advancement of Science (AAAS), 2023 Wilhelm Exner Medal from Austria, 2023 Fellow of the US National Academy of Inventors, 2023 de Gennes Prize in Materials Chemistry from the Royal Society of Chemistry, 2023 Elected Member of the US National Academy of Engineering, 2024 Fellow of the European Academy of Sciences, and 2025 ACS Henry H. Storch Award in Energy Chemistry.
Shortwave infrared (SWIR) has various applications, including night vision, remote sensing, and medical imaging. SWIR organic photodetectors (OPDs) offer advantages such as flexibility, cost-effectiveness, and tunable properties. In this talk, I will discuss the development of OPD materials processed from green solvents and device engineering to extend the wavelength detection range of OPD to a longer wavelength. We demonstrate a proof-of-concept in PTB7-Th:COTIC-4F blend system, achieving external quantum efficiency (EQE) > 50 % over a broad spectrum (450 – 1100 nm) with a peak specific detectivity (D*) of 1.1 x 1013 Jones at 1100 nm, while cut-off bandwidth, speed, and linearity are preserved. By employing a novel small-molecule acceptor IR6, a record high EQE = 35 % and D* = 4.1 x 1012 Jones are obtained at 𝝀 = 1150 nm. Additionally, we develop a high-performance non-halogenated-solvent-processed BHJ OPD utilizing a novel ultranarrow-bandgap NFA to achieve an exceptional EQE of ~20 % at λ = 1200 nm while retaining sub-μA.cm−2 dark current density level and D* > 3 × 1011 Jones over a broad spectrum of 400 – 1200 nm. This work emphasizes the importance of molecular design in optoelectronic devices.
1.2-I3
The strategic opportunities of emerging photovoltaics lies in their potential for sustainable large-area manufacturing using scalable solution-processing techniques such as slot-die coating. Perovskite solar cells are, facinatingly, to date the best solution-processable solar cell technology on the verge of commercialization. Mass-production, however, requires the development greener fabrication protocols.
In this talk we will addresses the dual challenges of scalability and environmental sustainability by exploring the development of more environmentally benign ink formulations for perovskite solar cells. Using protic ionic liquids (PIL) as ink additives in water- and alcohol-based precursor inks, we optimized the ink chemistry and process parameters to achieve uniform films with superior optoelectronic properties by slot-die coating. The PIL-based ink underwent a direct liquid-to-solid transition during film formation without forming intermediates, as confirmed by in-situ grazing incidence wide-angle X-ray scattering (GIWAXS). The optimized solvent system, a mixture of water, isopropanol (IPA), and MAP, enabled one-step slot-die coating at ambient conditions, achieving slot-die coated solar cell efficiencies up to 10%. This eco-friendly ink reduces toxicity, extends shelf life, and is stable in ambient environments, presenting a viable pathway for sustainable commercial applications.
This work highlights the potential for green ink formulations in scaling up perovskite solar cell manufacturing. Future efforts will focus on developing data infrastructure to integrate life cycle assessment (LCA) at early ink development stages, enabling the systematic design of environmentally optimized precursor inks and processes.
1.3-I1
Thomas D. Anthopoulos is a Professor of Emerging Electronics at the University of Manchester in the UK. Following the award of his BEng and PhD degrees, he spent two years at the University of St. Andrews (UK), where he worked on organic semiconductors for application in light-emitting diodes before joining Philips Research Laboratories in The Netherlands to focus on printable microelectronics. From 2006 to 2017, he held faculty positions at Imperial College London (UK), first as an EPSRC Advanced Fellow and later as a Reader and full Professor of Experimental Physics. From 2017 to 2023, he was a Professor of Material Science at King Abdullah University of Science and Technology (KAUST) in Saudi Arabia.
The rapid advances in increasing the power conversion efficiency (PCE) of organic photovoltaics (OPVs) have been mainly driven by advancements in new materials and the reduction of performance losses associated with traditional cell structures. As the PCE values of state-of-the-art OPVs have now exceeded 20%, the importance of cell engineering—including the active layer, interfaces, and light management—becomes even more crucial and, in most cases, determines the ultimate cell performance. In this talk, I will discuss our recent efforts to increase the PCE of OPVs to well beyond 20%. I will demonstrate how the use of engineered charge-extracting interlayers can help boost the overall performance of the cells as well as their operating lifetime. Moreover, I will highlight our latest advancements in utilizing molecular dopants to enhance the PCE and show how their combination with innovative interlayers and appropriate cell structures can improve material utilization and overall device sustainability, all while maintaining record levels of PCE.
1.3-S1
Celeste Brady is Deputy Editor for the Royal Society of Chemistry's journals ChemComm and Chem Soc Rev. She has been with the Royal Society of Chemistry for four years as Deputy Editor for the Energy portfolio and Development Editor for the Analytical portfolio. Prior to this she worked as an editor for the journal Epigenomics following her studies in natural sciences at University College London.
A brief introduction to the Royal Society of Chemistry's extensive range of journals, including Energy & Environmental Science and the EES Family. The EES Family is synonymous with exceptional research in energy and environmental science. Our premier journals are committed to publishing only the highest quality and exceptional work on energy and environmental science. The Royal Society of Chemistry publishes 58 journals with a commitment to rigorous, fair peer review and fast publication times.
1.3-I2
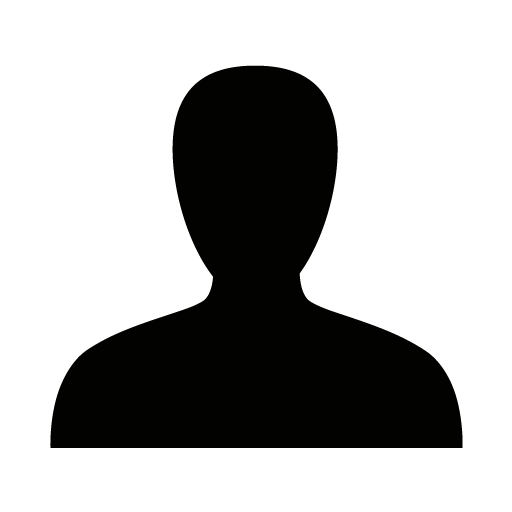
As the power-conversion efficiency of organic solar cells exceeds 20%, it becomes a priority to reproduce such performance in systems made from upscaleable materials and processes, and in systems with high operational stability. Small changes in chemical structure, solvent, fabrication process and device scale can all result in disappointing solar cell performance relative to a high-efficiency reference. Methods are needed to interpret changes in device performance in terms of the structural and spectroscopic properties of the active layers, in order to gain insight into how processing affects the basic operational mechanisms of the devices. At the same time, processing approaches that maintain the long term stability of the devices are needed.
In this talk we will present an approach to interpret the luminescence from active layer materials and devices in terms of basic molecular parameters and then analyse the effects of variations in material processing on these parameters in order to rationalise comparative device performance. Temperature dependent luminescence is analysed in terms of the Marcus-Levich-Jortner framework using Bayesian inference to distinguish the influences of different molecular parameters. We show the impact of process parameters such as solvent, additive and blend ratio on model parameters; and also explore the dependence of the quality of the fit on the range of data used in fitting. In a second study we show how the thermodynamic stability of organic heterojunctions can be controlled using novel ternary systems.
2.1-I1
Solution deposition from nanoparticle dispersions allows the use of eco-friendly processing agents such as alcohols or water for the fabrication of organic semiconductor thin-films for optoelectronic applications. Primary application of the eco-friendly device production from organic nanoparticles are solar cells where solvents are evaporated on large scale upon drying. Omitting surfactants to stabilize the dispersions is essential to not jeopardize the device performance. In this work, novel surfactant-free nanoparticle dispersions from high-performance organic semiconductors are synthesized by nanoprecipitation and electrostatically stabilized by electrical doping. The corresponding solar cells achieved power conversion efficiencies beyond 10%, matching the performance of reference solar cells deposited from toxic solvents. When applied to organic photodetectors, the nanoparticle approach allows the fabrication of thick absorber layers which enhance the quantum efficiency in the outer parts of the absorption spectrum, yielding enhanced broad-band detectivity. Aqueous dispersions of the organic semiconductor nanoparticles can be used for catalytic hydrogen generation.
2.1-I2
Conjugated polymers (CPs) have garnered significant attention in the fields of photodetectors[1], [2] and photoacoustic due to their tunable electronic properties, ease of processing, and potential for integration with flexible devices. Among these, shortwave infrared (SWIR) conjugated polymers offer a promising avenue for advanced photodetection and imaging applications, thanks to their ability to absorb and emit light in the 1–2 µm wavelength range, which is less susceptible to scattering and absorption by biological tissues compared to visible and near-infrared light. This feature enhances their suitability for deep tissue imaging, as well as for high-performance photodetectors with improved signal-to-noise ratios. In this work, recent advancements in the design and synthesis of new SWIR-responsive conjugated polymers, based on thiadiazolequinoxaline units as electron withdrawing units and various building blocks as electron donating segments will be presented including the incorporation of optimized π-conjugation and side-chain engineering that have led to significant improvements in their absorption, charge transport, and photodetection efficiency. Additionally, their biocompatibility and ease of functionalization open new possibilities for non-invasive bioimaging through photoacoustic application, enabling real-time monitoring of cellular processes and disease progression.
2.1-I3
<p>Bulk-heterojunctions (BHJs) comprising mixed phases of donor and acceptor organic semiconductors have propelled the performance of organic photovoltaics (OPVs) and organic photodetecors (OPDs) to performances closing in on those of competing technologies. However, poor control over interfaces and concerns over the long-term stability of such mixed phases mean that alternative architectures, such as bilayers or single-components, are re-gaining in research attention.</p> <p>Here, I will first present a simple and potentially low-cost hybrid organic/inorganic bilayer architecture for photodetectors and colour-tuneable solar cells, comprising the low-cost wide-bandgap inorganic semiconductor copper thiocyanate (CuSCN) and small-molecular acceptors. I will show that photodetectors using this simple bilayer can achieve an extremely low dark-current (10<sup>-8</sup> Acm<sup>-2</sup> @ -2V), which is partly enabled by low non-radiative voltage losses , and an extremely low noise spectral density [1]. Coupled with a light spectral respone that is widely tuneable through the choice of organic acceptor, this enables the fabrication of photodetectors with a specific detectivity in the near-infrared that approaches that of commerical Silicon photodetectors.</p> <p>Secondly, I will report on the characterisation and modelling of a variety of non-fullerene acceptors for single-component light-to-charge generation, which have been proposed to potentially offer a new avenue for opto-electronic devices. We combine experimental characterisation under varying conditions (field, temperature, excitation) with molecular and device-level calculations, to relate exciton and charge dissociation efficiency in single-component devices to molecular parameters, and investigate the potential of using free charge-generation in single organic semicondcutors for optoe-electronic devices.</p>
2.2-I1
Sustainably-printed organic semiconductors are cornerstones in the pursue of environmentally-friendly, large-area solution-processed energy generation technologies such as organic photovoltaics. Among them, poly[[6,7-difluoro[(2-hexyldecyl)oxy]-5,8-quinoxalinediyl]-2,5-thiophenediyl]] (PTQ10) shines as semiconducting polymer with an inherently low synthetic cost and adequate compatibility with non-halogenated and non-aromatic solvents, thus meeting the requirements for a low cost, human- and environmentally-safer open-air film production with uncompromised device performance.
In this talk, the microstructural features of the archetypal PTQ10 polymer are thoroughly studied by x-ray diffraction and atomic force microscopy methods for its application onto sustainably printed organic solar cells. PTQ10 films processed by either spin or bar coating in neat and co-solvent formulations that match the up-scaling green processing requirements are showcased and rationalized in terms of characteristic structural features (e.g., d-spacings, degree of paracrystallinity) within the Hansen solubility framework. The use of PTQ10 as donor polymer in a non-halogenated photoactive layer blend is then presented and exploited to form versatile indoor and outdoor organic solar cells [1]. The industrial potential of this organic photovoltaic blend is further demonstrated in all-printed modular form factors, accordingly deployed for agrivoltaic energy harvesting in a domestic greenhouse in Sweden [2]. Unprecedented failure modes related with module delamination are therein observed as a consequence of the harsh humidity and thermal cycling conditions found inside the greenhouse. Overall, the use of PTQ10 and its variants as donor polymer shows enormous potential for efficient and sustainably-printed organic solar cells, photodetectors and thin film transistors.
2.2-I2
Solution-processed nanocomposite films comprising small molecule organic semiconductors (OSCs) and inorganic colloidal quantum dots (QDs) are promising systems for low-cost, high efficiency, solar energy harvesting technologies.[1,2] In these systems, OSCs capable of singlet fission (SF) absorb high energy photons and generate triplet excitons which are harvested by inorganic QDs. Here, radiative recombination results in the emission of multiple lower energy photons which could be absorbed by an optically coupled silicon-photovoltaic (Si-PV) module, offering a route to surpass the radiative efficiency limit. Achieving efficient photon-multiplication requires a precise nanoscale morphology, with QDs uniformly dispersed within the OSC matrix at length scales comparable to the triplet exciton diffusion length. However, mismatches in size, shape, and surface energy between QDs and OSCs typically results in strong aggregation and phase separation. Whilst a proof-of-concept system has been achieved based on a tetracene derivative,[3] the emission of the OSC is spectrally mismatched to Si-PV absorption, limiting its use in practical applications. This work explores the self-assembly of high-triplet energy OSCs based on diphenylhexatriene (DPH) and dithienohexatriene (DTH) derivatives that offer improved spectral matching with Si-PV. In-situ grazing incidence X-ray scattering is employed during high-throughput blade coating to study the self-assembly of promising DPH-/DTH-based systems. Improved QD dispersions are obtained by tailoring QD surface chemistries and optimizing thermal processing conditions. The results provide design rules and scalable processing strategies for developing photon-multiplier films, paving the way for their integration into high-efficiency Si-PVs for enhanced energy harvesting.
2.2-O1
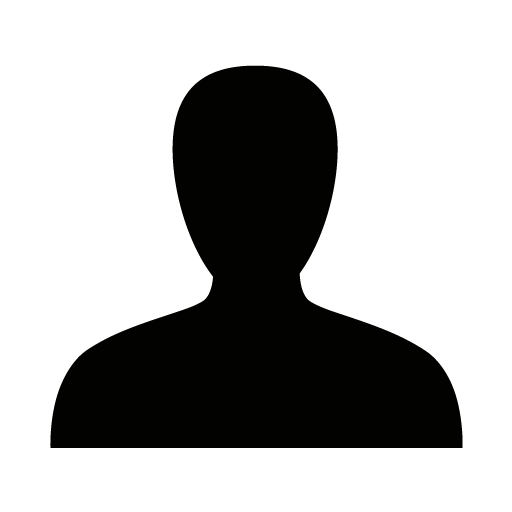
The efficiency of polymer solar cells (PSCs) has made impressive progress in the last few years. Still, the synthetic complexity of some of the best performing materials is high and is a possible bottleneck towards scalable commercial applications. In this work we present a new class of donor polymer that can be prepared in just two steps from commercially available starting materials.[1] The simplicity of the synthesis allowed a library of polymers with differing alkyl-chain lengths and comonomers to be readily prepared and investigated. The thermal, electrochemical and photophysical properties of the resulting polymer library helped towards the development of structure-property design guidelines. The polymers were furthermore investigated as donor materials in solar cell devices with Y6 and L8BO as acceptor, with the best performing material FO6-T showing highly promising power conversion efficiencies (PCEs) of 15.4 %. Recently, we also showed that our polymer FO6-T is compatible with green solvents such as 1,2-xylene and 2-MeTHF.[2] In order to study the scalability and commercial viability of the newly synthesised donors compared to state-of-the-art materials, I conducted a synthetic complexity (SC) analysis, which takes into account different industrially relevant parameters, such as hazardous chemicals involved and the number of synthetic steps, and we found that our material shows one of the lowest SC for well-performing donor materials to date.
We believe that these materials are highly promising for commercial application in PSCs due to strong device performance and a low cost and truly simple two step synthetic protocol.
2.2-O2
As our societies struggle to meet their ambitions for decreasing greenhouse gas emissions and the Paris Climate agreement, research into alternative solar energy conversion and storage technologies becomes even more relevant. Solar fuel production by photocatalysis is an economically promising route, especially when driven by earth abundant and organic materials. While organics’ bottom-up design possibilities promise tailorable structure-function relationships for enhanced activity, the advancement is often hindered by limiting knowledge of interwoven photo-physical processes and properties that lead to recombination losses.[1]
In this talk, I will explain how time-resolved (transient) spectroscopy techniques in combination with varying environmental conditions can be used to provide insights into the very beginning of the solar energy conversion process chain, focussing on exciton generation and separation, and charge stabilization. This enables us to better understand light-matter interactions, and to tailor them to address bottlenecks associated with exciton recombination.
Our recent study on a series of functionalized polymers with varying porosity has revealed that not a maximization of a BET surface area is key, but rather the active interaction area with the photocatalytic environment, if exciton or charge separation is driven by sacrificial agents.[2]
In context of photocatalysis, interactions with aqueous ions, which are highly relevant for enabling applications in sea water conditions, are typically disregarded. Our study on suspended nanoparticles in presence of different salt shows how ions can impact stabilization of excitons and significantly extend their lifetimes, thereby enabling a new way to address excitons’ commonly fast and rate-limiting recombination.[3]
Lastly, I will introduce Terahertz permittivity measurements as convenient technique to probe the complex permittivity, and with that the dielectric properties of organic semiconductors on ps-time scales. The dielectric response defines exciton binding and is hence relevant for charge carrier photogeneration in all solar energy conversion technologies, but its values are highly frequency dependent, and commonly extracted at timescales orders magnitude off the ps-regime. Our study focussing on carbon nitrides now reveals dielectric screening and transport properties at the early time scales of solar energy conversion process chains. At the same time, it shows that also in this ultrafast regime, the environment and ions can matter, and strongly enhance photophysical parameters.[4]
References:
[1] T. Banerji, F. Podjaski, J. Kröger et al.: Polymer photocatalysts for solar-to-chemical energy conversion. Nat. Rev. Mater. 6, 168–190 (2021).
[2] B. Willner, C. M. Aichiston, F. Podjaski et al.: Correlation between the Molecular Properties of Semiconducting Polymers of Intrinsic Microporosity and Their Photocatalytic Hydrogen Production. J. Am. Chem. Soc. (2024), https://doi.org/10.1021/jacs.4c08549.
[3] F. Podjaski*, S. Gonzalez Carrero, C. Aichiston, P. Khlat, K. Steward, L. Hart, S. Hillman, J.-S. Kim, I. McCulloch, J. R. Durrant. In preparation.
[4] R. Jahangir, F. Podjaski*, P. Alimard, S. A. J. Hillman, S. Davidson, S. Stoica, A. Kafizas, M. Naftaly, J. R. Durrant: Terahertz-permittivity of Carbon Nitrides: Revealing humidity-enhanced dielectric properties on the picosecond timescales relevant for charge carrier photogeneration. Submitted. Preprint: https://www.arxiv.org/abs/2411.06226.
1.3-I1
Juan Bisquert (pHD Universitat de València, 1991) is a Professor of applied physics at Universitat Jaume I de Castelló, Spain. He is the director of the Institute of Advanced Materials at UJI. He authored 360 peer reviewed papers, and a series of books including . Physics of Solar Cells: Perovskites, Organics, and Photovoltaics Fundamentals (CRC Press). His h-index 95, and is currently a Senior Editor of the Journal of Physical Chemistry Letters. He conducts experimental and theoretical research on materials and devices for production and storage of clean energies. His main topics of interest are materials and processes in perovskite solar cells and solar fuel production. He has developed the application of measurement techniques and physical modeling of nanostructured energy devices, that relate the device operation with the elementary steps that take place at the nanoscale dimension: charge transfer, carrier transport, chemical reaction, etc., especially in the field of impedance spectroscopy, as well as general device models. He has been distinguished in the 2014-2019 list of ISI Highly Cited Researchers.
The potentiation and depression of synaptic conductivity regulate the plasticity and adaptability of synapses. In this discussion, we examine the general dynamic characteristics of ionic or electronic current conduction in memristors, which underpin the fundamental principles of synaptic activity. Key model requirements for memristors or chemical inductors to achieve conductance adaptation in response to incoming stimuli are outlined. We also propose various criteria, such as hysteresis and rectification, to achieve these properties. Additionally, we describe a range of diagnostic methods that link nonlinear time responses, the nonlinear cycling of current-voltage curves, and the linear frequency responses from impedance spectroscopy to evaluate adaptation properties. The frequency domain analysis of memristors and more generally, of conducting systems with memory features of some kind, provides essential information about the dynamic behaviour of the system. The impedance response of a memristor can be represented as a linear circuit made of resistances, capacitors, and inductors, with voltage-dependent elements. The equivalent circuit properties also establish the criteria for a Hopf bifurcation that produces spiking of artificial neurons.
1.3-O1
The growing demand for data-driven applications calls for computing architectures going beyond traditional von Neumann architectures, where the separation between memory and processing units causes increasing latency and bandwidth bottlenecks [1]. This challenge has sparked interest in emerging ‘neuromorphic’ devices and systems, where memory and computation are seamlessly co-integrated. The brain-inspired circuits can be built from such devices by combining novel metal-oxide materials, such as HfO2 [2] and VO2 [3] that can implement synaptic and neuronal functions in the neural networks [4]. These neuro-inspired devices exhibit promising features, such as ultrafast and analog resistive switching at low energy costs. In this talk, I will present our work on VO2-based oscillator networks solving combinatorial optimization problems, including Graph Coloring, Max-cut, and Max-3SAT problems [5]. Additionally, the in-memory computing architecture of the system will be introduced by combining with HfO2-based resistive RAM (ReRAM) devices for enhanced performance and efficiency.
1.3-O2
A comparison of the resistive switching (RS) operation in memristors based on HfO2, Al2O3 and a bilayer made of both oxides is presented. The devices are fabricated using the TiN/Ti/dielectric/TiN stack, and they are measured for thousands of cycles to assess their cycle-to-cycle variability. A statistical analysis is performed including 1D and 2D algorithms. A compact modeling approach (using a modified version of the Stanford model) is implemented to describe RS operation and variability. Finally, a 3D circuit-breaker-based simulation tool is employed to fit the experimental data and assess the role of thermal effects in the different dielectrics employed.
Bipolar valence change memory devices have been fabricated and measured [1]. The memristors fabrication is based on highly doped N-type (ρ = 4 mΩ·cm) silicon wafers with a 20 nm-thick Ti adhesion layer. The bottom electrode consists of a 50 nm-thick W layer. The dielectrics were grown by atomic layer deposition. The top electrode is composed of a 200 nm TiN/10 nm Ti bilayer. Thousands of I-V curves were measured in both types of devices under the ramped voltage stress regime. Resistive switching was characterized by means of consecutive set and reset cycles. RS parameters, such as the set and reset voltages, were obtained.
The set and reset voltages are obtained using different algorithms to assess the variability. We calculate one-dimensional coefficients of variation (1DCV) and two-dimensional ones (2DCV), the latter are explained in [2].
We complement the study by means of a compact modeling approach. We are able to reproduce the change that takes place in the I-V curves of the different technologies. Simulation using a 3D circuit breaker tool [3] is also performed to fit average I-V curves for the technologies studied here.
1.3-O3
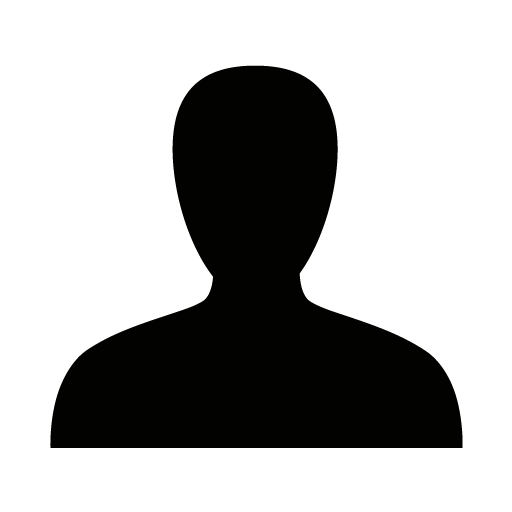
Valence Change Memory (VCM) cells are emerging as promising candidates for non-volatile memory and neuromorphic computing applications [1,2]. These devices typically employ a simple metal-insulator-metal (MIM) structure, where an applied voltage modulates the insulating oxide layer. This modulation drives the incorporation and redistribution of oxygen vacancies, enabling the oxide layer's resistance to switch dynamically between a high-resistance state (HRS) and a low-resistance state (LRS). These resistance states are non-destructively readable, offer rapid switching, and exhibit excellent scalability, CMOS (complementary metal-oxide-semiconductor) compatibility, and low power consumption [3–6].
Despite their advantageous properties, the reliability of VCM ReRAM devices remains a critical challenge, particularly as these devices approach commercial viability [7]. Experimental studies, such as those by Kempen et al., have demonstrated that extrinsic doping can significantly enhance the reliability of TaOx-based ReRAM by improving endurance and lowering the forming voltage [8].
Building upon these experimental insights, we incorporated dopants into our three-dimensional Kinetic Monte Carlo (3D KMC) simulation framework previously developed for analysing reliability phenomena in VCMs [9,10]. Our simulations investigated the influence of dopant concentration and energy levels on key reliability metrics, including variability and retention. The results corroborate the experimentally observed improvements, revealing the positive impact of doping on reliability and providing new insights into the underlying physical mechanisms. These findings contribute to advancing the understanding of dopant-driven reliability enhancements in VCM ReRAM technology.
1.3-I2
The industry adoption of memristor-based technologies hinges upon improvements in several key performance metrics for these devices, including controllable switching, multiple resistive states, reducing the variability over multiple switching cycles, and reduction in the switching and forming voltages, depending upon the specific requirements for different application domains. Of these, a new kind of memristor comprising two different metal oxides as active layers between inert electrodes, referred to as the bilayer resistive RAM (ReRAM), has been shown to possess gradual analogue switching characteristics [1], making it particularly suitable to act as the variable coupling element between oscillators within the energy-efficient neuromorphic computing framework of oscillatory neural networks (ONNs) [2,3]. However, the atomistic mechanisms underlying the unique behaviour of bilayer ReRAMs remain to be uncovered, which is crucial for understanding and further optimization of this technology for its integration with the ONN computing hardware. Another peculiar characteristic of this device is the high voltage requirement for the forming step, which is the first process when the initial structural changes related to the resistance-switching behavior occur in the pristine device [1]. In this work, we reveal the detailed ionic response in this device to understand its filamentary forming mechanisms and explain its observed characteristics using atomistic simulations. For this, we use an implementation of a novel molecular dynamics simulation framework, integrating the local electrochemical potential formulation (called EChemDID) [4] with the charge transfer ionic potential (CTIP) [5,6], to capture the accurate physics of this device. We clarified the differences in the peculiar responses of each of the anionic and cationic species in the pristine structure in response to applied voltage and joule heating in the device, and the physical reasons underlying these mechanisms. Our results indicate that an oxygen-rich region could form on top of the filament in bilayer ReRAM devices, as a consequence of repulsion of the Tantalum ions that occurs even at lower temperatures. Furthermore, the filament initiates at the cathodic interface of the active MO layers by agglomeration of the vacancies when the applied voltage is beyond a critical threshold voltage, and an increase in temperature significantly accelerates the growth of the filament, particularly owing to an increased thermally excited mobility of tantalum and oxygen ions promoting bond breakage and vacancy formation. These mechanisms reveal the key processes underlying forming in the bilayer ReRAM, which could be controlled for directed optimization and further improvement of the key performance metrics of these novel devices. This project has received funding from the EU’s Horizon program under Projects No. 101092096, PHASTRAC.
2.1-I1
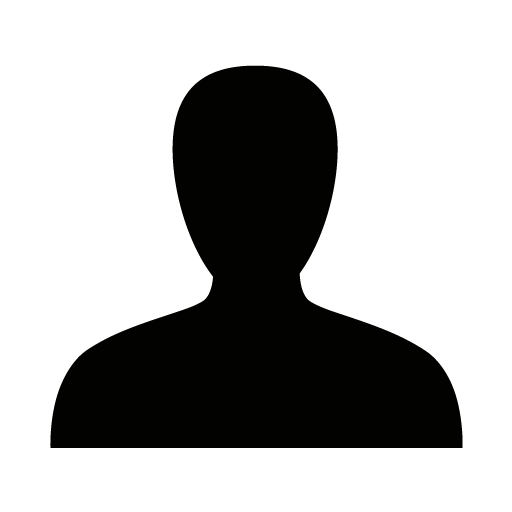
Memristor devices post-fabricated on top of CMOS circuits promise the avenue to implement low power neuromorphic cores implementing in-memory computing architectures, where the dense memristive matrix act as dense synaptic adaptive memory following bioinspired learning rules as spike-time-dependent-plasticity (STDP). However, the present CMOS-memristive technologies present integration density limitations due to the need of compound MOS-memristor synapses (called 1T1R) as well as problems with control memristive analog values and its variations.
Circuit design techniques can help to overcome this limitations as well as served as an orientation for technological developments to make possible the implementation of advanced low power CMOS-memristive SNN computing architectures.
At IMSE neuromorphic group, we have implemented a dense CMOS-memristive architecture exploiting a CMOL-like geometry to partially overcome the 1T-1R integration limit. Furthermore, we have developed stochastic binary STDP learning rule and experimentally demonstrate its robust performance on a CMOS-memristive hardware. During this talk, the CMOS-memristive SNN computing hardware will be explained, with experimental characterization results at the device and neural system level.
2.1-I2
In recent years, the cloud-based approach to data classification has been challenged by the edge computing paradigm, which has enabled real-time data processing at the network edge, ideally in close proximity to the sensor that is collecting the data. This paradigm presents significant challenges in terms of power efficiency, compactness, and latency [1, 2]. It is therefore necessary to explore unconventional hardware solutions that are able to meet these exacting requirements.
Brain-inspired architectures, particularly spiking neural networks (SNNs), have the potential to achieve low-latency computation and stateful, energy-efficient operations [3]. However, their current implementations are primarily based on digital or mixed-signal Complementary Metal-Oxide-Semiconductor (CMOS) technologies, which present significant challenges in meeting the demanding memory, area, and power constraints of computing at the network edge. [1].
The integration of emerging memory technologies in the back end of the line (BEOL) of CMOS circuits holds significant promise for enhancing the capabilities of CMOS technology [2, 4] for the development of neuromorphic hardware [5]. The exploitation of their unique properties – including operation voltages compatible with current CMOS technology, as well as analogue, neural-/synaptic-like behaviour – offers an attractive opportunity for realizing energy-efficient and massively parallel computing architectures in conjunction with CMOS technology [3, 5]. Indeed, these features enable efficient computation, neural dynamics, and synaptic plasticity, which are essential traits for emulating the brain's functionality in hardware [4, 6]. However, the achievement of this goal is still an open challenge at several levels, from the fabrication to the integration with circuits to the architecture.
This talk emphasizes the necessity of design-technology co-optimization (DTCO) of emerging memory devices and CMOS circuits to facilitate seamless integration and to leverage the strengths of both technologies. Additionally, it discusses the importance of identifying and addressing issues related to device variability, scalability, and system integration in the co-design of devices, circuits, and algorithms.
2.1-I3
Memristive devices are currently explored for neuromorphic and emerging unconventional computing concepts towards future low-power computing systems. These devices have been proposed as: (i) computing elements to support in memory computing in neural networks, (ii) building blocks to reproduce in hardware synaptic or neural functionalities, and (iii) key elements to build nonlinear dynamical circuits. In this framework, both volatile and non-volatile resistance switching memristive devices are widely studied to engineer various computing functions [1].
This talk will present our recent results in the field of volatile electrochemical memristors based on the Ag/SiOx/Pt structure and how to implement neuromorphic functionalities such as short-term plasticity, paired-pulse facilitation and inhibition, and integrative functions. We will discuss how the interplay between switching times and relaxation effect controls the memristors dynamics and possible various switching modes that can be used to reproduce key synaptic and neuronal functions [2].
The second part of the talk will discuss an unconventional computing approach based on analogue Pt/HfO2/TiN RRAM devices. We exploit the programmable nonlinearity of the non-volatile memristors to build an analogue nonlinear dynamical circuit based on the Murali- Lakshmanan-Chua (MLC) architecture [3]. The circuit can be tuned from periodic to chaotic behavior through the modulation of an input signal. We demonstrate its processing ability in an approach based on single-node reservoir for various nonlinear classification tasks.
2.2-O1
In the era of artificial intelligence (AI), the advancement of deep neural network (DNN) algorithms has pushed conventional digital hardware to its limits due to power efficiency constraints [1]. Recently, analog AI hardware, consisting of crossbar arrays of resistive memory devices, has garnered significant attention as a promising solution to overcome the inherent bottleneck of the von Neumann architecture [2]. These systems enable a more efficient mapping of neural network architectures to hardware, with resistive devices representing the weights. Here, resistive memory (ReRAM) technology, in a crossbar array configuration, play a crucial role in realizing analog AI hardware [3]. ReRAM devices store synaptic weights as conductance values, while the crossbar array physically implements the synaptic interconnect on real hardware. Thanks to its design compatibility with the neural network structure, the ReRAM-based crossbar array can accelerate NN inference as well as deep learning through parallel information processing and weight updating within the memory [4]. This presentation introduces conductive-metal-oxide/HfOx ReRAM technology for DNN hardware accelerators and explores the opportunities presented by both the devices and arrays in the system.
2.2-I1
Neuromorphic hardware systems emulate the parallel neural networks of the human brain, and synaptic weight storage elements are crucial for enabling energy-efficient information processing. They must represent multiple data states and be able to be updated analogously. In order to realize highly controllable synaptic devices, replacing the high-k gate dielectric in conventional transistor structures with either solid-electrolytes that facilitate bulk ionic motion or ferroelectric oxide allows for steady adjustment of channel currents in response to gate-voltage signals. This approach, in turn, accelerates backpropagation algorithms used for training neural networks. Furthermore, because the channel current in electrochemical random-access memory (ECRAM) is influenced by the number of mobile ions (e.g., Li or Cu cations) passing through the electrolytes, these synaptic device candidates have demonstrated an excellent linear and symmetrical channel current response when updated using an identical pulse scheme. In the latter case, which is known as the ferroelectric field-effect transistor (FeFET), the number of electrons accumulated near the channel rapidly varies with the degree of the alignment of internal dipoles in thin doped ferroelectric HfO2. This leads to a multilevel state. Based on the working principles of these two promising candidates, enabling gate-controlled ion-transport primarily in electrolytes for ECRAM and understanding the relationship between polarization and the ferroelectric layer in FeFETs are crucial to improve their properties. Therefore, this study aims to present our recent advances, highlighting the engineering approaches and experimental findings related to ECRAM and FeFET for three-terminal synaptic devices.
2.2-I2
Asal Kiazadeh, PhD in Electronics and Optoelectronics, leads the Memristor Group at CENIMAT/i3N and serves as a lecturer at the University of Nova de Lisboa, Faculty of Engineering. She has extensive expertise in flexible oxide electronics and has secured five project grants as a Principal Investigator and key team member, funded by national government agencies and the European Commission. Her research focuses on memristor technology for advanced communication systems, such as THz non-volatile RF switches, and computational domains, including neuromorphic vision and brain-inspired low-power neural network hardware. She has authored over 40 research articles, with 30 of them dedicated to memristor technology.
Amorphous oxide semiconductor (AOS) technology has emerged as a transformative platform for the development of advanced memory and logic devices, particularly in the field of flexible electronics. This talk will explore the evolution of AOS-based devices, from Thin-Film Transistors (TFTs) to Resistive switching RAMs (RRAMs), and their integration into a range of computing paradigms. AOS materials, such as Indium Gallium Zinc Oxide (IGZO), offer unique properties like high mobility, low temperature processing, and excellent flexibility, making them ideal for next-generation electronics. We will first examine the capabilities of IGZO TFTs, which have demonstrated remarkable performance in various display, sensor, and neuromorphic applications, highlighting their role as efficient access transistors in RRAMs crossbar arrays. Next, we will discuss the transition to RRAMs and how these devices exploit the resistive switching mechanism for non-volatile memory, enabling high-speed, low-power data storage. The advantages of RRAM technology, including its scalability, endurance, and energy efficiency, will be emphasized, particularly in the context of emerging neuromorphic computing systems such as Reservoir computing (RC). We also discuss the integration of AOS-based RRAMs into flexible and wearable electronics, highlighting their potential to revolutionize both conventional and unconventional computing paradigms. The goal of this talk is to provide insights into the progress of AOS-based RRAM technology and to inspire further exploration of its potential to reshape the future of computing, from memory-intensive applications to neuromorphic and AI-driven systems.
2.2-O2
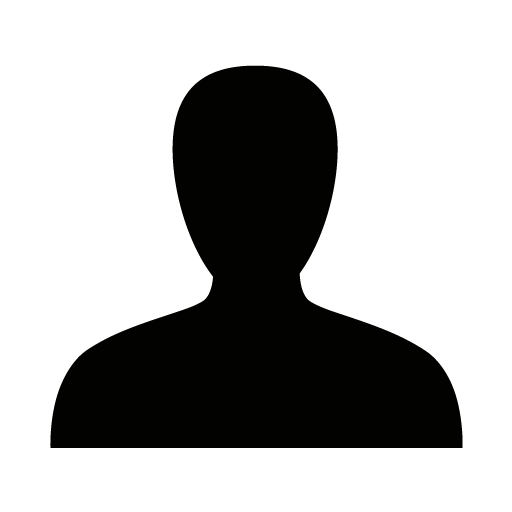
According to the advents of big data processing technologies and artificial intelligence (AI) generation, next-generation memristors have been in the spotlight as technologies to overcome the bottleneck limitations of von Neumann. Memristor (memory + resistor) is one of the key elements for high-performance integrated memory and neuromorphic computing applications with low energy consumptions and efficient processing. Among various memristor candidates, halide perovskites (HPs) have been actively studied as potential candidates for these devices due to their unique switching characteristics with low power consumption, simple fabrication, flexible integration compatibility across various sources for scalability, etc. However, there is still a lack of dynamic physical analysis and overviews about operating mechanisms and characteristics of HPs-based memristor and neuromorphic devices, which are essential for future application realization with high performance and accuracy. So here, we overview and outline the various characteristics and operating principles of the HPs-based memristors and the basic neuromorphic devices. First, briefly introduce HPs-based memristors and neuromorphic applications and discuss different switching types /operating mechanisms according to the conducting pathway occurring inside the active HP layer to figure out the beneficial types for the desired memory/neuromorphic device applications. Finally, show the analyze tools and physical dynamic models for the general insight to electrical actuation of the memory/neuromorphic systems from various perspectives.
2.3-I1
Miguel Muñoz Rojo received his PhD (2015) in Condensed Matter Physics & Nanotechnology from the Spanish National Research Council (CSIC) and M.S./B.S. in Physics from the Autonomous University of Madrid. He obtained a JAE pre-doctoral Fellowship from CSIC to study during his PhD how the reduction of dimensionality affects the transport properties of organic and inorganic thermoelectric materials. During this period of time, he carried out scientific stays at the Rensselaer Polytechnic Institute (New York, USA), the University of Bordeaux (France) and the University of California Berkeley (USA). In 2012, he participated in the 62nd Lindau Nobel Laureate Meeting in Physics after qualifying in an international competition among young talent scientists. From 2016 to 2018, he became a postdoctoral researcher at Stanford University, studying two dimensional (2D) materials and devices based on them for thermal, electrical, and thermoelectric applications. From 2018 to 2021, he was a Tenure Track Assistant Professor at the University of Twente. He has been successful in obtaining funding for his research in USA and Europe, including the prestigious ERC Consolidator Grant 2023, in the field of thermal conversion and management processes with national and international academic and industrial partners. He is now a permanent researcher at the National Research Council of Spain (CSIC) working at the Institute of Materials Science in Madrid (ICMM) with double affiliation as associate professor to the University of Twente. He is currently a Fellow of the Young Academy of Europe. His research focuses on multiscale thermal engineering, thermal management, energy harvesting, nano- and micro-scale thermometry and thermal sensing.
One of the greatest challenges of modern society is related to energy consumption, dissipation and waste. A prominent example is that of integrated electronics, where power dissipation issues have become one of its greatest challenges. In this talk, I will discuss how to characterize energy dissipation in electronics, like heating in transistors based on 2D materials or in the conductive filaments of resistive random-access memories (RRAM), using spatially resolved thermometry. As the size of materials and devices shrinks to nanometer, atomic, or even quantum scale, it is more challenging to characterize their thermal properties reliably. Scanning thermal microscopy (SThM) is an emerging method to obtain local thermal information of electronic devices by controlling and monitoring probe–sample thermal exchange processes. Gaining thermal insights of our electronics is essential to design energy efficient circuits and understand and optimize ultra-dense data storage.
2.3-O1
Antonio Guerrero is Associate Professor in Applied Physics at the Institute of Advanced Materials (Spain). His background includes synthesis of organic and inorganic materials (PhD in Chemistry). He worked 4 years at Cambridge Dispaly Technology fabricating materiales for organic light emitting diodes and joined University Jaume I in 2010 to lead the fabrication laboratory of electronic devices. His expertise includes chemical and electrical characterization of several types of electronic devices. In the last years he has focused in solar cells, memristors, electrochemical cells and batteries.
The ionic conductivity of halide perovskite is responsible for a memory effect that can be used in resistive memories.1,2 The ionic conductivity can be controlled by the dimensionality of the halide perovskite and this is connected to the long-term stability of several optoelectronic devices. In this work, we discuss how the interplay between ion migration and the chemical reactivity with the external contacts is key to maximize the reliability of the devices, to control the working mechanism and to reduce the energy consumption. Conductive and insulating states are formed via migration of halide vacancy and electrochemically active metals. We show that the working mechanism and performance of the memory devices can be tuned and modified from volatile to non-volatile response.3 Several configurations are evaluated in which structural layers are modified systematically: formulation of the perovskite,4 the nature of the buffer layer5 and the nature of the metal contact3,6. We show that in order to efficiently promote migration of metal contact the use of pre-oxidized metals greatly enhance the performance of the memristor and reduces the energy requirements. Overall, we provide solid understanding on the operational mechanism of halide perovskite memristors that has enabled increased stabilities approaching 105 cycles with well separated states of current and further improvements expected.
2.3-O2
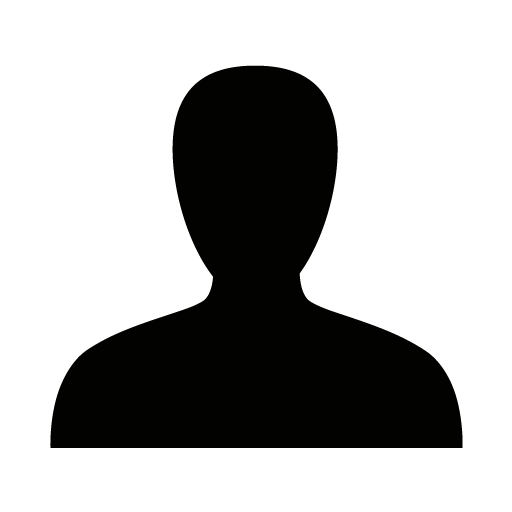
The ever-growing prevalence of AI and the IoT necessitates substantial increases in power consumption and data storage capacity. Memory storage devices that are fast, power-efficient, and have a high packing density are thereby gaining interest in the data storage industry. Neuromorphic computing (NC) fits resistive random-access memory (RRAM) devices well because of its decision-making and picture-recognition capabilities. A two-terminal resistive switching device based on Cu/CuO/FTO demonstrated excellent non-volatile RRAM appearances with 150 repeatable cycles, LRS and HRS stability in terms of retention is 1,000 s, and durability for 5000 cycles. The device was designed with inspiration from the human biological brain and was able to be synthesized at a low cost by thermal oxidation of Cu. The active material was CuO, with Cu and FTO serving as the top electrode and bottom contact. We investigated these devices' potential applications in neuromorphic computing. In addition, the devices show impressive mimicking abilities, displaying features such as synaptic weight, learning, and forgetting characteristics, spike time-dependent plasticity (STDP), and pulse-paired facilitation (PPF). In addition, the synaptic artificial neural network shows outstanding short-term (STP) and long-term (LTP) potentiation for six cycles in a row. Therefore, the current study on devices based on Cu/CuO/FTO offers a comprehensive analysis of resistive switching based on CuO active materials, which could lead to neuromorphic computing that goes beyond the von Neumann architecture.
1.2-I1
Perovskite solar cells (PSCs) are a promising emerging technology on the cusp of commercialisation. With record power conversion efficiencies >26%, they are excellent candidates for low-cost and low-embodied energy photovoltaics. Their emergence as a promising technology is timely as we are on the brink of significant climate change and face the limits of current linear economic models. Transition is necessary to ‘circular economy’ with widespread deployment of sustainable green energy technologies. However, the deployment of green technologies, including PSCs, presents significant sustainability challenges, including availability of critical raw materials, environmental impacts of mining and production and waste generation at end-of-life.[1,2] Achieving widespread deployment necessitates a continuous supply of critical raw materials and lifecycle optimisation early in development to ensure true sustainability. This includes minimising production-related environmental impacts, developing end-of-life strategies, designing for longevity, selecting low-impact materials, and substituting primary and critical resources. Here we will discuss the work of the SPECIFIC Innovation and Knowledge Centre and the UNESCO Chair in Sustainable Energy Technologies at Swansea University, which aims to advance the sustainability, understanding and scaling of perovskite photovoltaics.[1-5] It is our contention that perovskite photovoltaics, as an emerging energy technology, have great potential to be designed for a circular economy and optimised end-of-life processing to deliver significant sustainability benefits. We will discuss opportunities for the for distributed manufacturing of perovskite photovoltaics to help transform energy access as well as assessing resource constraints and implementing circular economy strategies.[1] Early evaluation of future waste streams and materials supply issues are essential to avoid technological lock-in and ensure sustainable pathways forward.
1.2-I2
Emmanuel Kymakis is a Full Professor at the Department of Electrical & Computer Engineering at the Hellenic Mediterranean University (HMU) and Director of the Institute of Emerging Technologies of the HMU Center for Research & Innovation. He received his B.Eng. (First Class Honors) degree in Electrical Engineering & Electronics from Liverpool University in 1999 and the Ph.D. degree in Electrical Engineering from Cambridge University in 2003. He and Prof. Gehan Amaratunga are the inventors of the polymer-nanotube solar cell. Before joining HMU, he was a technical consultant offering engineering and consultancy services in the realization of photovoltaic and solar thermal power plants. His multidisciplinary research lies at the interface between nanotechnology and electrical engineering and is centred on the development of printed optoelectronic and photovoltaic devices. He has published more than 140 research articles, which have attracted over 13.000 citations and an h-index of 62, while he has an extensive experience in the management of research and industrial projects (9,5 M€ research funding, design & implementation of 67 MWp of PV parks). Ηe is also included in the list of the top 2% of scientists in their respective fields by citation impact (PLoS Biol 17(8), e3000384). He serves also as scientific evaluator and member of panels of experts of various international governmental and nongovernmental agencies, member of scientific committee of various international congresses, and have been invited to give invited talks in more than 50 occasions. He has been an honorary lecturer at UConn and a recipient of an Isaac Newton and an EPSRC studentship. He was named as a 2014 ChemComm Emerging Investigator and has received two National Excellence Awards. He has served as a member of the founding General Assembly of the Hellenic Foundation for Research & Innovation (HFRI), a member of the Engineering sectoral scientific council of the National Council for Research & Innovation of Greece (NCRI) and a member of the Engineering thematic advisory council of HFRI. He is currently the Director of the interinstitutional Post-Graduate Program “Nanotechnology for Energy Applications” and serves as the work package leader of Energy Generation of the EU FET-Flagship Initiative Graphene.
Power conversion efficiencies (PCE) exceeding 26% have been achieved by perovskite photovoltaics (PePVs), which have emerged as a promising alternative energy solution. These efficiencies are comparable to classical silicon solar cells. They are appropriate for both low-power applications and large-scale solar farms due to their adaptability. Nevertheless, the preservation of high efficiency in large-area panels under real-world conditions continues to be a substantial obstacle. The complexities of outdoor exposure are not adequately captured by conventional lab-based testing protocols, as the performance and stability of the system are significantly influenced by fluctuating weather patterns and variable peak sun hours.The long-term performance of perovskite modules and panels in outdoor conditions will be the primary focus of this presentation, with an emphasis on the origins of a variety of degradation factors. Some of these factors are intrinsic to the perovskite active layer, while others are extrinsic, such as lamination failure. The effects of gloomy storage and light soaking on the panels and their partial recovery are investigated using detailed measurement protocols.The sensitivity of perovskite panels to environmental conditions such as humidity, high temperatures, and light exposure, which are significant degradation sources, poses a challenge to their long-term stability. Experimental results suggest that the solar farm experiences a more significant degradation during the summer as a result of protracted exposure to high temperatures and solar irradiance, which significantly impedes lamination stability. However, this degradation was discovered to be partially reversible following a period of dark storage. Changes in the light soaking phenomenon (LSP) were observed, as well as enhancements in the electrical parameters of the solar farm following dark storage. The time required for recuperation was contingent upon the severity of the panel degradation. Recovery occurred within the day-night cycle during the initial phases of operation; however, as degradation progressed, additional time was required. Panels were unable to regain their properties with dark storage alone at extremely low degradation levels, necessitating subsequent light exposure for performance recovery. This suggests a multifaceted interplay of degradation and recovery mechanisms that are contingent upon dark storage and light exposure. The rate of degradation of electrical parameters was also influenced by seasonal and environmental conditions, as evidenced by the seasonal behaviour of degradation. Visual inspections revealed that the panels had developed defects over time, which were linked to lamination failure and the penetration of oxygen and moisture. These defects had a negative impact on the panels' performance. The restoration of electrical parameters was impeded by severe optical degradation, even after dark storage. Studying the voltage mismatch of perovskite photovoltaics is another essential stage in the commercialisation of this photovoltaic technology, as it is essential to comprehend its influence on rate of degradation. This encompasses the identification of the most effective connection configuration (parallel and series) for the efficient long-term operation of a solar farm, as well as for the upscaling and fabrication of panels. In summary, the stability of photovoltaic perovskites is significantly influenced by lamination. This research demonstrates that the electrical properties of the panels can be restored provided that the lamination prevents the penetration of external factors (including moisture and oxygen). Nevertheless, it is imperative to conduct additional research on the ageing process in outdoor environments in order to distinguish between recovery scenarios in terms of the extent of degradation and long-term stability.
1.2-O1
Dr. Hadjipanayi is a research scientist at the Photovoltaic Technology group in the Department of Electrical and Computer Engineering of the University of Cyprus working on the investigation of the optoelectronic characteristics and photovoltaic performance of novel solar cell devices and her latest work focuses on the characterization of perovskite-based PV and measurement protocol development.
She has received her BSc in Physics (2001) from the University of Cyprus and her DPhil (PhD) in Condensed Matter Physics (2006) from the University of Oxford. Her employment record includes a Post-Doctoral Research Associate position at the Quantum Information Processing Interdisciplinary Research Collaboration (QIP IRC), Department of Physics, University of Oxford (2006-2009) and an Associate Research Scientist post at the Energy, Environment and Water Research Centre of the Cyprus Institute (2009-2012). Her research interests lie within the area of fundamental and applied physics of novel materials which are promising for future energy-efficient technological applications, especially in the field of solar energy. More specifically and more recently, these include: Investigation of optoelectronic properties and degradation mechanisms of novel solar cell devices including multi-junction solar cells, nanostructured silicon cells, perovskites; Development of accurate standardized and non-standardised testing protocols for new solar cell technologies.
Maria has over 10 years’ experience in national and European research projects as a partner and as a Coordinator covering the full project life-cycle involvement: from initiation to implementation, monitoring and reporting. She led the efforts to attract funds and develop a new strategic infrastructure unit at the University of Cyprus, the DegradationLab, which focuses in the accurate characterization of new and emerging solar cells, and is currently the Head of this new lab (https://fosscy.eu/laboratories/degradation-lab/).
Accurate predictive models are required for photovoltaic (PV) performance reliability assessment and failure diagnostics [1]-[3]. Studies have shown that machine learning models can accurately predict the power conversion efficiency of perovskite solar cells (PSCs) based on composition and structural parameters [4]-[5]. Machine learning algorithms have been utilized before [6] using indoor stability data sets to predict the outdoor stability of perovskite-based devices. However, machine learning models using high-throughput outdoor stability data from perovskite-based devices to predict their time-series power output is still limited in the literature [7-8].
This work aims to utilize several data-driven algorithms (based on machine learning principles) to predict the power output of different perovskite devices (both single and tandem configuration). Namely, three gradient boosting models (CatBoost, XGBoost and LightGBM) have been employed for predicting the PV performance and output power from perovskite-based devices based on long-term outdoor data. The prediction performance of the different machine learning models was evaluated using yearly datasets containing instantaneous field measurements obtained from the outdoor test site in Nicosia, Cyprus. In all cases, the PV time series dataset was split into a random 70:30% train and test set approach. More specifically, 70% of the dataset was used for model’s training, while the rest 30% was used for testing the accuracy of the models. Prior to the model development, data quality checks were performed [9] along the most influential input parameters using statistics (Pearson correlation) were identified.
For the evaluation of the predictive accuracy of the constructed models, the normalized root mean square error (nRMSE) metric was used [8]. The obtained results demonstrated that all models provide good predictive quality (nRMSE<7%) using the instantaneous measurements. Better prediction performance was provided by the LightGBM regression model which presented the lowest nRMSE (<4%) across the whole test set. Dependence of the prediction accuracy of the models with output power levels was detected with larger discrepancies between the actual and predicted power to obtained at lower power levels.
Evaluation of the performance of the models at different train set data partition as well as at different filtering conditions is underway. This study will provide evidence regarding the dependence of the predictive accuracy on the train set duration, data filtering conditions and irradiance profile classification.
1.3-I1
Senol Öz obtained his diploma in chemistry in 2013 at the University of Cologne
(Germany). Completing his PhD under supervision of Prof. Sanjay Mathur in 2018 at
University of Cologne (Merck KGaA PhD scholarship). In 2019 he joined Prof.
Tsutomu Miyasaka`s group as a post-doctoral fellow at Toin University of Yokohama
under a JSPS scholarship. His research interests include the synthesis, chemical
engineering, and solution processing of inorganic-organic hybrid metal halide
perovskite materials for photovoltaic application. He is currently a senior R&D project
leader at Saule Technologies and managing director of Solaveni GmbH.
Perovskite solar technology has rapidly transitioned into a commercially viable solution after just over a decade of intensive global research. Distinguished by its high specific power, cost-effective production, and exceptional performance under low-light conditions, it stands out among photovoltaic technologies for its broad applicability. Hybrid organic-inorganic perovskites uniquely combine the ease of solution processing typical of organic small molecules and polymeric semiconductors with the superior physical properties of high-performance crystalline inorganic semiconductors. This synergy results in a novel material class that harnesses the advantages of both organic and inorganic domains.
To enable the large-scale production of perovskite photovoltaics, the adoption of sustainable, safe, and industry-compatible processing methods is essential. Achieving sustainability begins not only with processing techniques but also at the material level. Solaveni, a Germany-based company, has pioneered the sustainable synthesis of perovskite precursors through innovative green halide chemistry. This presentation highlights the benefits of these novel synthesis routes for organic alkylammonium halides and metal halides, evaluated through life cycle assessment (LCA) and benchmarked against conventional production methods. The LCA examines the environmental impacts of these precursor materials—assessing resource consumption, energy use, and emissions—to provide critical insights into their sustainability profiles and ecological footprints. Furthermore, we introduce novel green recycling approaches for perovskite materials that enable rapid dissolution and recovery, fostering the development of a circular economy.
1.3-O1
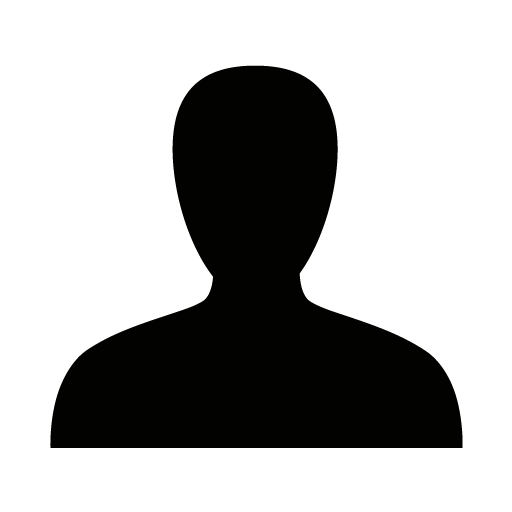
ORIGIN OF DEGRADATION IN THE LONG-TERM PEROVSKITE MODULE OUTDOOR PERFORMANCE IN DIFFERENT OUTDOOR REGIONS
In the domain of perovskite formulations, module architectures, and layer stacks offering possibilities for processing highly efficient devices, comparing performance stability data across literature presents a considerable challenge. While perovskite outdoor stability data has been investigated by some research groups [1][2] [3] most samples, even for many perovskite compositions and architectures, have been relatively short-lived. Specific investigations include extensive datasets, yet measurements are confined to cells[4],[5],[6]leading to conclusions influenced by the small device areas and lack of interconnections. Despite the presentation of high-performing devices showcasing operational stability, the hurdles in achieving fabrication on a larger scale persist. Various consortia and partnerships, involving universities, companies, research institutes, and national laboratories, have been established to address these issues. One such consortium is US-MAP (Manufacturing of Advanced Perovskites), which collects and uniformly reports outdoor performance data for perovskite modules.
Our methodology comprises a reproducible and scalable process, an operationally stable perovskite formulation, and a photo and thermally stable layer stack (layer stack: ITO/NiOx/ FA0.80Cs0.20Pb(I0.94Br0.06)3 /ETL/ITO). The robustness of this process is demonstrated by its run-to-run reproducibility, establishing a foundation for an equitable comparison of outdoor performance among samples. We present the real-time performance of our perovskite modules over 4 years and discuss the strategies employed to achieve more extended stability as we scaled up our modules to 800 cm2 [7].
Our innovative approach integrates a feedback loop based on in-lab characterization, outdoor performance results, and degradation analysis to enhance our understanding and enable adjustments for prolonged lifetimes. In the conference we will elaborate on our approach to the performance tests that have led to process modifications, giving rise to new generations of modules characterized by improved performance, stability, and area coverage.
We investigate the data for the correlation between outdoor performance and irradiance levels, alongside temperature variations. Figure 1 displays the mini-module PCE evolution up to the summer of 2024 alongside the temperature variations of the modules. Preliminary analysis suggests a consistent burn-in period for all samples, within the initial 3 months of outdoor deployment. This results in a performance decline of approximately 30% from the initial levels, followed by a performance stabilization over extended durations. From this dataset extending from 6 months in some locations to +4 years in others, we expect to discern dependences on irradiance levels, seasons, climates, and temperatures. Insights from the outdoor deployment of modules in Cyprus highlighted potential reasons for module failure, such as thermomechanical issues or the formation of ionic barriers. Through further investigation and deeper characterization, we aim to determine whether permanent degradation arises primarily from one of these stressors or a combination of both.
Figure 1: Upper plot: PCE evolution over time for samples considered in the study. Lower plot: Module temperature variations for different locations considered in the study.
Using the insights gathered from outdoor performance data, our objective is to refine our perovskite formulation, layer stack, and module design. Looking ahead to future developments, we are focusing on Gen 3 modules, to enhance the performance of our large-area modules while maintaining their exceptional stability.
1.3-I2
Eugene A. Katz received his MSc degree (1982) in Semiconductor Materials Science and Ph. D. (1990) in solid state physics from the Moscow Institute of Steel and Alloys. In 1995, he joined the Ben-Gurion University of the Negev and has been working in the Department for Solar Energy and Environmental Physics ever since (now as a full professor). His research interests include a wide range of photovoltaic materials and devices, such as organic and perovskite-based photovoltaics, concentrator solar cells operated at ultra-high solar concentration (up to 10,000 suns), etc. He has published more than 120 peer-reviewed papers on these topics. In 2018 Prof. Katz was awarded the IAAM Medal (by the International Association of Advanced Materials) for the outstanding research in the field of New Energy Materials & Technology.
Perovskite solar cells have exhibited meteoritic growth in their power conversion efficiency (PCE). However, there is a consensus among researchers in the field that the durability of perovskite photovoltaics under real operational conditions still requires considerable improvement. We should identify the dominant degradation mechanisms under multiple stresses in outdoor operation. It is even more complicated due to the well-known (but not still well-understood) metastable day-night dynamics of the perovskite PV. Another key aspect is that PSC degradation rates can strongly depend on electrical bias. We will demonstrate that even mechanisms of such degradation can be different at short-circuit, open-circuit, or maximum power point, for example. Furthermore, we will also report experimental results on the effect of electrical bias on PSC behavior after stress relief, including promoting the recovery process.
Research strategies towards improvement of PSC operational stability exhibiting (at least partially) reversible degradation may include both (1) mitigation of the degradation mechanisms and (2) promotion of the recovery process. Most of the published studies on perovskite PV were aimed at the former strategies. Knowledge about attempts to promote the recovery of perovskite PV is limited. Electrical “therapy” of degraded perovskite solar cells is possible but still should be developed.
2.1-I1
Halide perovskite photovoltaics is surging towards commercialization with the first industrially manufactured panels installed in the PV fields. With this, it is more important than ever for the researchers in the perovskite community to focus on identifying all the degradation and failure modes that could be triggered under real-world conditions to finding ways to overcome them.
In this contribution, we will share insights on the topic accumulated over the last 5 years of outdoor studies with perovskite solar cells exposed in Berlin, Germany. After testing a variety of perovskite absorbers, cell architectures and module encapsulation schemes, it is not surprising that we observed a host of different degradation pathways depending on the device architecture, including both extrinsic and intrinsic instability mechanisms [1]. Despite this, champion devices on our test field are already more than 4 years old providing an optimistic perspective of the possibility of reaching the desired operational lifetimes.
Severe failures occur if water vapour enters the device due to flaws in encapsulation. It results in different patterns of damage spreading depending on whether it originates from a specific weak spot (glass crack, imperfect adhesion of the edge sealant, etc.) or the overall high water vapour transmission rate of the encapsulation materials used. We also observe that encapsulation could be responsible for other degradation effects, such as layer delamination or chemical interaction between perovskite and encapsulation materials [2].
Intrinsic device stability, which is not related to the device encapsulation, is also critical. We confirmed such mechanisms as increased ionic losses under outdoor operation (due to electric field screening) [3], increased bulk defect concentration, localized phase segregation, and the formation of macroscopic defects. We also often see a change with ageing in the cell transient behaviour in the day-night cycle patterns. This constitutes another loss mechanism, often referred to as fatigue. Finally, we observed for a range of samples that the device layout can affect the ageing behaviour through various edge effects.
References
[1] S. Baumann, G. E. Eperon, A. Virtuani, Q. Jeangros, D.B. Kern, D. Barrit, J. Schall, W. Nie, G. Oreski, M. Khenkin, C. Ulbrich, R. Peibst, J. S. Stein, M. Köntges, Stability and reliability of perovskite containing solar cells and modules: degradation mechanisms and mitigation strategies. Energy Environ. Sci., 2024,17, 7566
[2] U. Erdil, M. Khenkin,* W. M. Bernardes de Araujo, Q. Emery, I. Lauermann, V. Paraskeva, M. Norton, S. Vediappan, D. K. Kumar, R. Kant Gupta, I. Visoly-Fisher, M. Hadjipanayi, G. E. Georghiou, R. Schlatmann, A. Abate, E. A. Katz, C. Ulbrich, Delamination of Perovskite Solar Cells in Thermal Cycling and Outdoor Tests. Energy Technol. 2024, 2401280
[3] S. Shah, F. Yang, E. Köhnen, E. Ugur, M. Khenkin, J. Thiesbrummel, B. Li, L. Holte, S. Berwig, F. Scherler, P. Forozi, J. Diekmann, F. Peña-Camargo, M. Remec, N. Kalasariya, E. Aydin, F. Lang, H. Snaith, D. Neher, S. De Wolf, C. Ulbrich, S. Albrecht, M. Stolterfoht, Impact of Ion Migration on the Performance and Stability of Perovskite-Based Tandem Solar Cells, Adv. Energy Mater. 2024, 2400720
2.1-I2
The transition to renewable energy is critical for decarbonizing global energy systems. Solar panels, alongside wind turbines, play a pivotal role in achieving this transformation. With projected annual photovoltaic production surpassing 1 terawatt peak (TWP) in 2025, the deployment of tens of billions of solar panels is essential to meet energy goals. However, as these panels reach the end of their lifecycle, the challenge of sustainable disposal or recycling arises. Opting for circular recycling over landfilling offers numerous benefits, including the recovery and reuse of valuable materials, which are vital to maintaining production sustainability. This underscores the urgent need for a circular photovoltaic market where production and recycling capacities are aligned.
Lead perovskite solar cells, a next-generation photovoltaic technology, have entered the field as a promising new technology with their high efficiency, scalability, and unique material properties, such as tunable bandgaps and high absorption coefficients. Perovskite solar cells hold both promises and new challenges with respect to recycling and sustainability of photovoltaic technologies. On the one hand, perovskite solar cells can be deposited from solutions, enabling material recovery with very high selectivity. On the other hand, perovskites pose environmental challenges, particularly regarding the sourcing and recycling of lead halides. Addressing this, our research focuses on i) developing circular recovery and recycling processes for all materials used in a perovskite solar cell and ii) integrating perovskite solar cells into a circular economy by repurposing lead waste into materials for new solar cell fabrication.
With respect to circular recycling of perovskite solar cells, we demonstrated a closed-loop recycling of MAPbI3 perovskite solar cells that achieved a 99.97% mass recovery through a layer-by-layer solvent extraction method. Critical components, including ITO glass, SnO2, MAPbI3, and spiro-OMeTAD, were recovered and purified. Devices fabricated with recycled materials maintained performance on par with those using virgin components, with peak efficiencies reaching 19%. A techno-economic analysis showed that implementing this recycling approach could reduce material costs by 63.7% in lab-scale production. These results underscore the potential of closed-loop recycling to enhance the sustainability and cost-effectiveness of perovskite photovoltaic technology.
With respect to utilizing lead, we developed a recycling process starts to convert contaminated lead into lead iodide (PbI₂). This method achieves near-complete conversion, with a Faradaic efficiency close to 1. An essential step involves purifying the synthesized PbI₂ through single-crystal growth, yielding, in one example, 35% pure PbI₂, while the remaining material is recycled for subsequent loops. This scalable process produces PbI₂ at approximately 1g per hour, with potential for industrial application by increasing electrode area.The recovered PbI₂ is directly utilized to fabricate new perovskite solar cells, achieving efficiencies exceeding 20%. This result not only demonstrates the viability of recycling perovskite materials but also establishes a sustainable pathway for addressing the environmental concerns of lead waste. By converting contaminated lead from various sources, including legacy lead waste from industries and household applications, our approach eliminates significant ecological hazards and contributes to a circular economy.
2.1-I3
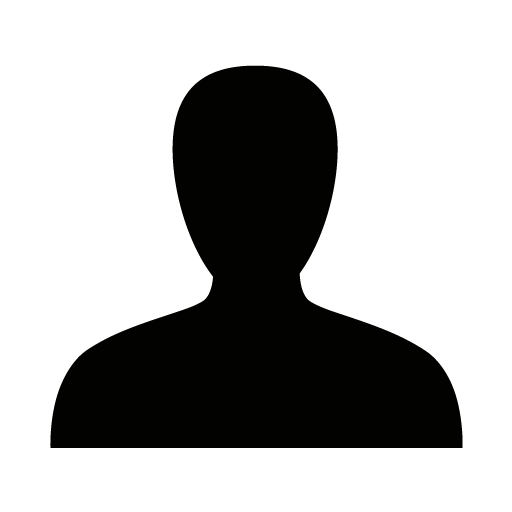
Chinese companies like Microquanta, GLC Power and Utmolight are already producing perovskite modules and are planning production lines of 1-2 Gigawatts each in the next years. Also for tandem devices perovskites become more and more import, OxfordPV has the first modules on the market and according to the 15th edition of the International Technology Roadmap for Photovoltaics, 10% global market share of perovskite-silicon tandems are estimated for the year 2034.[1] This sounds not so much but will correspond to > 100GW, as for 2028 the global PV market is estimated to be 876 GW. [2]
These upcoming huge amounts in mind, recycling of perovskites will be an important issue in the future and in contrast to silicon modules, there is no existing industrial recycling concept for perovskite modules or perovskite-silicon-tandem modules.[3] Here we will present first results of the PeroCycle project, funded by the Deutsche Bundesstiftung Umwelt (DBU). Within the project we investigate a direct recycling approach: Perovskite mini-modules are produced and encapsulated at ZSW, in the second step the modules are sent to Solar Materials where the glasses are separated in one piece from the absorber materials. In the third step, the glasses are cleaned and the absorber material is purified at Solaveni. Finally, with the recycled glasses and perovskite materials new modules will be manufactured at ZSW and analyzed to evaluate the feasibility and quality of the recycling process.
Besides recycling, we focus also on stability issues. An outdoor test of our semitransparent modules is running since 18 months at HZB and we carry out damp heat and constant illumination tests.
2.2-I1
The long-term stability of perovskite solar cells (PSCs) depends not only on the inherent stability of their device layers but also on the effectiveness of their encapsulation. In commercial photovoltaic (PV) technologies, thermal lamination with over-device sealants, such as ethylene vinyl acetate (EVA) or similar polymeric films, is commonly employed. However, PSCs require an additional edge-sealing layer to significantly reduce water vapor permeation rates, ensuring enhanced protection for the device[1].
Glass frit sealants, known for their superior hermeticity and mechanical durability[2], have emerged as a highly effective encapsulation solution for PSCs. When applied through laser-assisted edge-sealing techniques, these materials have successfully protected PSCs under different standard durability tests as outlined by IEC61646 protocols [3, 4].
This discussion focuses on the influence of encapsulation hermeticity on the long-term stability of PSCs. Specifically, we examine the impact of the atmospheric composition within the cavity of glass frit-sealed devices. Our study investigates the light-induced degradation of perovskite films sealed under different atmospheres, including air, nitrogen, argon, and carbon dioxide. Results indicate that encapsulated printable HTM-free PSCs demonstrate significantly improved lifetimes when sealed under nitrogen and carbon dioxide atmospheres compared to air.
These findings highlight the role of controlled internal atmospheres in mitigating degradation and ensuring the durability of PSC devices.
2.2-I2
Perovskite solar cells have established themselves as the front runner new technology to enter the PV market. Their fast development is now promising that both single-junction and tandem perovskite modules will soon enter industrial production. First modules have reportedly already been commercially available, nevertheless, there are still things to learn about perovskite field operation, arising questions about their long-term stability. While stability is an important and often researched topic, the large scale stability tests have not been performed often. Instead, typically only the stability of the champion device is shown. Several factors contribute to that, such as complex and expensive equipment as well as availability of a large number of similarly performing perovskite devices.
In the presentation, we will show our recent results obtained with the in-house developed WLED system that is designed for simultaneous long-term MPP tracking of up to 144 perovskite solar cells. Besides discuss reproducibility of our devices, the influence of light intensity on stability of the devices, and compare stability under cyclic and continuous illumination. Special focus will be paid to disentangling humidity and light as main degradation stressors for perovskite devices. For that, capping of devices with Al2O3 is implemented, enabling long-term testing without moisture degradation. This makes long-term measurements in air are possible without the need for encapsulation, which should accelerate mass testing of perovskite solar cells. Finally, we will also discuss aspects of perovskite tandem solar cell testing and present a dedicated bichromatic setup for their testing.
2.2-O1
Laboratory-scale power conversion efficiencies (PCE) of ABX3 type perovskite solar cells have surpassed 26%. However, the typical solution-processed methods, involving spin-casting, antisolvents, or gas quenching, and using N,N-Dimethylformamide (DMF) and Dimethyl sulfoxide (DMSO) solvent systems, pose scalability and stability challenges. To address this, we optimized a DMF/DMSO and antisolvent-free solvent system for 1.48eV FAPbI3-based p-i-n architecture solar cells using 2-methoxyethanol (2-ME). We incorporated N-Methylpyrrolidone (NMP), a non-volatile and Pb2+ coordinating additive, to inhibit non-perovskite phase formation during room-temperature deposition. Unlike common Pb2+ coordinating agents such as DMSO, DMPU, or DMAc, our method showed no precipitation at any concentration. With high reproducibility, we achieved a stable maximum power point tracking (MPPT) of ~23.00% for a 0.25 cm2 and ~22% on a 1 cm2 aperture area, attributed to enhanced photoluminescence quantum efficiencies (~8%), compact and pinhole-free morphology, and a highly oriented crystal structure. This technique is easily scalable for larger areas. Despite these efficiency gains, stability remains crucial for commercial viability. Our study investigates the high stability of optimal bandgap FAPbI3 perovskite using in-situ 2D XRD. For examples: we stressed thin films at 130°C and 50% RH, noting that DMF films turned yellow, while 2-ME films retained their black phase. Optoelectronic and Photovoltaics Devices response tests revealed ~no degradation in 2-ME films and devices, following Damp Heat, ISOS-D2 and ISOS-L3 protocols. Loss quantification using half-stack PLQE measurements identified the perovskite/C60 interface as the performance limiter.
2.2-O2
Markus Kohlstädt is a project manager and senior scientist at Fraunhofer Institute for Solar Energy (ISE) and the Freiburg Materials Research Center (FMF) of University of Freiburg. He studied Chemistry and was awarded a PhD by University of Freiburg in 2009. By now, he has more than 13 years experience in in the fabrication and characterization of Organic and Perovskite solar cells and modules, with focus on cell stack development and upscaling. In 2022, he was appointed leader of the team “Thin-Film Perovskite Photovoltaics” at Fraunhofer ISE.
Besides demonstration of scalable processes for realization of perovskite based photovoltaics, stability of corresponding devices under operating conditions needs to be demonstrated to rationalize their industrial fabrication and marketability. To this end, we investigated outdoor stability of encapsulated 4-terminal perovskite-silicon tandem modules. In parallel, the stability of the individual devices in a single junction architecture was tested, i.e. of silicon solar cells illuminated with a spectrum not filtered by the wide band gap perovskite top cells and of semitransparent perovskite single junction modules without silicon solar cells at their back.
It is quite frequently discussed that applied load, e.g. by tracking the maximum power point (MPP) of the device under test, can positively affect stability, possibly due to a higher concentration of free charge carriers under open circuit conditions [1]. We performed comparative stability tests with devices in single or tandem configuration and held at open circuit or maximum power point and could not find significant changes with regards to device stabilty, which confirms one of our earlier studies on single junction perovskite modules [2]. Periodic scans of the full IV curves of all devices allowed tracking of individual solar cell figures of merit and their analyses in dependence of e.g. illumination conditions or temperature.
1.1-O1
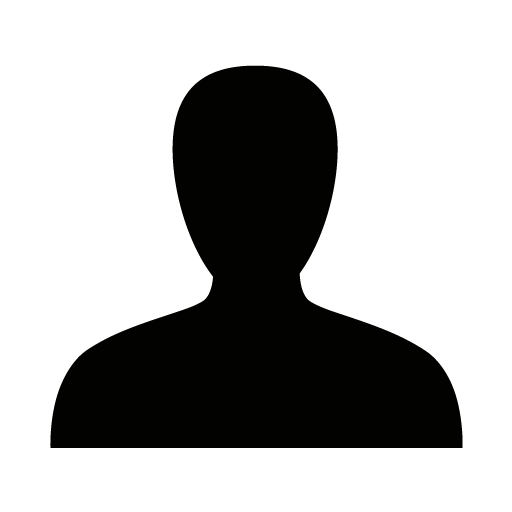
All-solid-state batteries are identified as the next generation of batteries. The advantage of these technologies lies in the solid nature of the electrolyte, which could enhance safety and energy density of the systems. The current state of the art is still far from the anticipated expectations, and ongoing studies identify several challenges responsible for this gap. Firstly, all-solid-state technology requires careful management of the pressure applied. It must take into account the mechanical properties of the components and be adapted to ensure good ionic conductivity1. Secondly, the fabrication of components must be homogeneous in composition and densification. The distribution and contact of active material and electrolyte particles are fundamental to achieving the expected capacity. Finally, these materials must be chemically and electrochemically compatible to avoid accelerated degradation of the system2. In our research, we aim to develop all-solid-state prototype references through the coating process. The objective is to study the performance during prolonged cycling and to investigate the failures mechanisms. Optimization of formulations, processes, and cycling conditions has enabled us to achieve five-centimeter-square pouch-cell format prototypes with reproducible performance over several hundred cycles. The electrochemical test was conducted at a low current density of 70 µA·cm⁻² under 60°C with a relatively low pressure of 3 MPa. The work presented will focus on the development of prototypes. The design of the separator, with a thickness of 400 µm obtained by coating, will be particularly detail, as it is the critical component of this technology3. The use of the coating process creates porosity during the solvent evaporation step. This porosity is not suitable because it could affect ionic conductivity and create preferential pathways for lithium dendrite growth. However, the main advantage of this process is that it is widely known and used in current battery manufacturing plants. Therefore, the transition to all-solid-state battery production would be facilitated and accelerated. In this presentation, various optimizations and strategies to overcome the porosity issues will also be discussed.
1.1-I1
Rechargeable batteries can contribute to powering electric transportation and storing electrical energy generated from intermittent renewable sources. Li ion batteries (LIBs) are the current battery of choice and new types of batteries such as solid-state Li metal batteries (SSLMBs) are also under active research. There are constant demands for further improving rate capability and energy density of batteries. Thick electrodes can increase energy densities at the cell level, but one of the limiting factors is slow ion transport that reduces capacity at increasing (dis)charge rates. This problem is exacerbated in SSLMBs as the ion transport coefficient is usually lower in solids than in liquids. Here, two novel processing technologies have been developed to optimise the battery electrode microstructure and improve ion diffusion kinetics: (i) directional ice templating (DIT) for fabricating thick (900 µm) cathodes with vertical pore arrays and porosity gradient for LIBs [1]; and (ii) directional freezing and polymerisation (DFP) for fabricating cathodes with vertical arrays of solid polymer electrolyte (SPE) directly incorporated in the cathode microstructure during processing for SSLMBs [2]. Both techniques reduced the tortuosity of ion diffusion pathways through electrode thickness from ~3.3 for conventional electrodes to 1.5.
A new operando correlative imaging technique of combining X-ray Compton scattering imaging (XCS-I) and computed tomography (XCT) has also been developed that allows pixel-by-pixel mapping of Li+ chemical stoichiometry variations in a LiNi0.8Mn0.1Co0.1O2 cathode in an SSLMB [3]. This technique shows how the anisotropic electrode microstructure improved Li+ ion diffusivity, homogenised Li+ ion concentrations, and improved energy storage performance.
1.1-O2
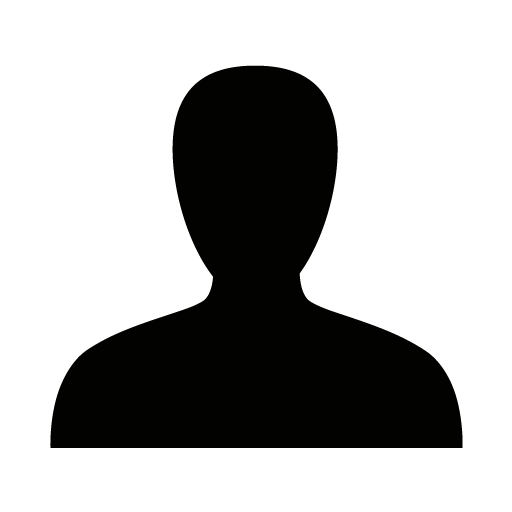
Nowadays, commercially available lithium batteries are based on liquid electrolytes, due to their high ionic conductivity at room temperature. But the search for new battery technologies that can substitute the existing ones has become very important in the last decades, mainly due to issues related to safety, durability and manufacturing complexity. Hence, all-solid-state lithium-metal batteries (employing solid electrolytes) have gained attention because of their higher energy density, while being safer, and more chemically and thermally stable than conventional lithium-ion batteries [1,2]. When it comes to solid electrolytes, ceramics offer higher chemical and thermal stability, better mechanical properties and wider electrochemical window. However, their low performances and interfacial detrimental interactions with the electrodes still need to be improved for their implementation into final devices.
A possible strategy to address these drawbacks and improve battery performance involves the employment of innovative manufacturing processes, as 3D printing and ultra-fast high temperature sintering (UHS). Both techniques are explored in this work for NASICON-type ceramic electrolytes and Co-free cathodes.
Li1.5Al0.5Ge1.5(PO4)3 (LAGP) electrolytes were printed by stereolithography (SLA) in complex geometries generally impossible to produce with conventional ceramic manufacturing techniques. Up to date, only a few studies have reported the production of full ceramic electrolytes by 3D printing [5-7]. Here we present the development of LAGP full-ceramic electrolytes by SLA, exploring different complex geometries to improve the electrolytes behaviour (i.e. area specific resistance, critical current density, available capacity in a full battery). Crack-free dense (>80% of theoretical density) LAGP electrolytes were succesfully prepared, showing conductivities in good agreement with LAGP manufactured by conventional techniques (10−4 S cm−1), without detrimental reactions or degradation due to the debinding step. Additionally, plating/stripping galvanostatic tests were carried out on symmetrical cells Li/3DPrinted-LAGP/Li up to 200h.
In parallel to the development of electrolytes, Co-free composite cathodes have also been studied. LiFePO4 (LFP) was chosen as active material to be combined with LAGP in the composites. The cathodes were attached by an optimized conventional thermal treatment (650 ºC, 4h, N2 atmosphere) onto LAGP sintered pellets. The compatibility of the involved materials was investigated showing no degradation phenomena or unwanted reactions. Finally, a full cell Li/LAGP/LFP-LAGP(50:50) was mounted, tested by EIS and cycled at constant currents. Despite the limited capacity detected, these first results represent a promising step forward towards full ceramic batteries based on these combination of materials.
UHS was also developed for both electrolytes and cathodes. This technique was presented in 2020 as a sintering technique capable of densifying ceramics in seconds, to reduce both the manufacturing times and energy consumption respect to conventional thermal processes, while avoiding degradation phenomena [8-10]. In the case of materials containing lithium, UHS can also be helpful to minimize lithium losses [8-10]. UHS evaluation on sintering of electrolytes and cathodes will be presented. LAGP was sintered by UHS in less than 2 minutes reaching very high densification (88%) and ionic conductivities comparable with the ones obtained by conventional sintering. Furthermore, LFP-LAGP composite cathodes were successfully attached on LAGP electrolytes by UHS avoiding detrimental interfacial interactions. As last step UHS was successfully applied for debinding and sintering of 3D printed LAGP electrolytes, lowering the times needed for these processes from days to minutes.
Finally, advanced characterization techniques as Grazing-Incidence Wide-Angle X-ray Scattering using Synchrotron radiation (GIWAXS) and Glow Discharge Emission Spectrometry (GDOES) were employed to study possible side reactions between these materials, and lithium content differences comparing conventional thermal treatments and UHS.
1.2-I1
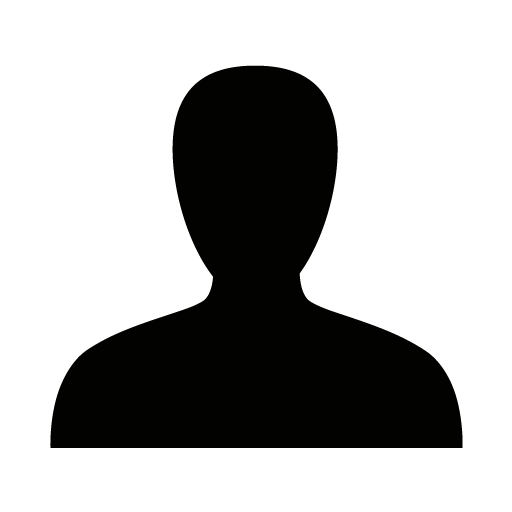
All Solid-State Batteries (ASSBs) are emerging as the next-generation energy storage devices due to their superior safety and performance potential compared to traditional lithium-ion batteries (LiBs). The presence of a solid electrolyte enhances safety by minimizing flammability and mitigates dendrite propagation, enabling the use of high-capacity lithium metal anodes (3860 mA h/g). Combined with high-voltage cathodes like LMNO, ASSBs promise significant improvements in energy density, both gravimetric and volumetric beyond incumbent LIBs. Ceramic materials show mechanical properties that can reduce the propagation of dendrites and interesting ionic conductivity for lithium ions.
Oxides exhibit high stability with lithium metal and mechanical properties to prevent dendrite propagation, but face challenges in room-temperature ionic conductivity and require high sintering temperatures to achieve the mechanical and structural qualities to be used as electrolytes.
Sulfides achieve high ionic conductivities (up to 10 mS/cm) at room temperature and high relative densities (>90%) without sintering. However, their air sensitivity delayed their entrance into the battery market.
Halides, with moderate ionic conductivities (1-2 mS/cm), bridge the gap between oxides and sulfides. They offer room temperature processability and operation with less toxic byproducts, despite their sensitivity to atmospheric exposure.
Across all ceramic electrolyte types, optimizing interfaces, particularly with lithium metal, remains a key challenge for achieving higher performance in ASSBs. This work focuses on the intrinsic behavior and reactivity of these electrolytes with individual cell components and examines the synergies formed by combining different chemistries. Advanced characterization techniques such as NMR, EIS, and DRT are employed to investigate the phenomena occurring during the operation of solid-state batteries. We present here practical cases on how material design, processing techniques, and interface engineering play a significantly effect on the performance of the cell.
1.2-O1
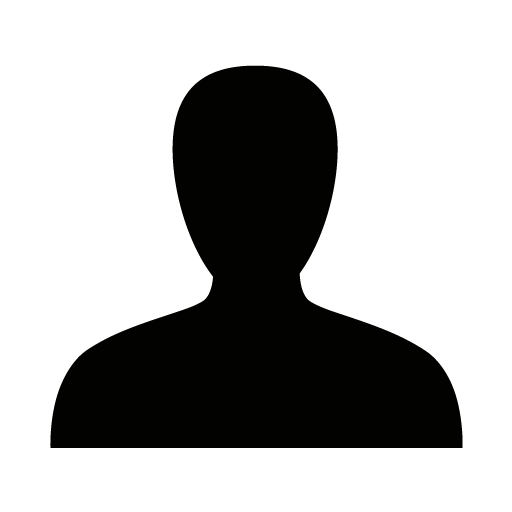
The performance of high energy density solid-state Li-metal batteries is typically also limited due to unstable interface between solid electrolytes and the Li metal. This is particularly critical when employing NASICON-based solid electrolytes, where the transition metal tends to reduce in the presence of Li-metal, together with the solid-electrolyte decomposition. In this work, the interface stability of the Li1.3Al0.3Ti1.7(PO4)3 (LATP) and Li1.5Al0.5Ge1.5(PO4)3 (LAGP) with Lithium metal has been improved by coating of the electrolyte with binary metal oxides, such as Alumina and Zirconia, as a function of thickness employing the atomic layer deposition (ALD) technique. Furthermore, our studies suggest that after coating the as deposited Alumina and Zirconia layer with specific metal, the assembled coin cells can run with low overpotentials and high stability even at medium current densities. For these studies, the coin cell CR2032 have been assembled in symmetrical mode (Li/interlayers/solid-electrolyte/interlayer/Li) and cycled with current density of 6.4, 12.8 and 25.6 μA cm-2 for 2 h platting and stripping conditions (50 cycles at each current density). Impedance studies also suggest an improved interface with low contact resistance before and even after the cycling at high current densities as compared to the pristine LATP as well as LAGP. During this presentation, we will discuss the electrochemical performance of our surface-modified cells and present structural characterization to address the mechanisms for the observed improvements.
1.2-I2
Li1-xAlxTi2-x (PO4)3 (LATP) ceramics are claimed to be one of the most attractive for Solid State Electrolytes (SSEs) ceramics for the next generation of Li batteries.[1] This is due to its advantages, such as non-flammability, excellent electrochemical stability, no leakage risk, non-volatile materials, and low production costs. As the most important component of All Solid-State Lithium Battery (ASSLB), the high ionic conductivity, good interfacial compatibility and stability are the basic requirements, but some of them still remain a challenging. In addition, the search for suitable electrolytes with sufficiently high ionic conductivity does also includes the development of new eco-friendly processing techniques.
As an alternative, solid electrolyte LATP ceramics have been successfully sintered by Cold Sintering Process (CSP) using transient liquid phase (TLP) and solid modifiers. This process allows samples to be sintered below 200 ºC, meaning that composites such as ceramics in polymer can be sintered. And, of course, to reduce CO2 emissions in line with the European Climate Law, which requires a reduction in net greenhouse gas emissions of at least 55% by 2030.[2]
Here, we have studied the CSP to sinter ceramic electrolytes at 150 ºC and ~700 MPa, to boost a competitive ionic conductivity. A screening upon the LAPT solid electrolyte, from morphology and particle size, the nature and content of TLP, including the effect of polymer addition, shows that SE show relative density in the range of 85 – 90 % of the theoretical value of LATP, and the total ionic conductivity achieved by CSP is competitive with those sintered by high temperature treatments. Electrochemical impedance spectroscopy (EIS) technique is employed during the sintering process as a control tool to monitor the densification.[3]
Moreover, EIS is also used during the cycling of the half-cells to identify limiting factors of the cold-sintered electrolyte kinetics and to predicts the overall electrochemical behaviour. The results suggest that ionic conductivity fading can occur in the prepared samples due to the low grain boundary ionic conductivity and secondary phases in the intergranular regions, and that additives used in the processing are critical to the final microstructure.
The viability of a simple and innovative method of densifying ceramic powders at low temperatures by applying uniaxial pressure and using a transient acid solution is demonstrated.
1.2-I3
Ainara is a Tenured Scientist at the Intituto de Ciencia de Materiales de Madrid, CSIC and Visiting Reader in Energy Materials in the Department of Materials, Imperial College London. Her research focuses on the quantitative anlysis and optimisation of ion and electron dynamics in complex oxides, bulk surfaces and interfaces. She uses a combination of structural, chemical and electrochemical analysis including surface sensitive techniques and operando characterisation to develop the next generation of solid-state electrochemical devices such as metal anode all-solid-state batteries, low and intermediate temperature solid oxide fuel cells and electrolysers. She has been awarded with fellowships and grants as PI up to €3,3M and is involved in several UK , Spanish and European Commission projects. She has published over 80 (>3.2k citations h=29, i10=52) research papers in this field and holds 2 patents on their applications.
Enabling scalable processing of halides with high Li conduction
R. Artal1, R.del Olmo2, N. Stankiewicz2, S. Daubner3, R. Jimenez-Rioboo1, S. Cooper3, I. Villanueva2, A. Aguadero1,3
1 Instituto de Ciencia de Materiales de Madrid, CSIC, Madrid, Spain
2 POLYMAT, University of Basque Country, San Sebastian, Spain
3 Imperial College, London, United Kingdom
The development of all-solid-state batteries (ASSBs) has the potential to be transformational leading to safer, longer life and higher energy and power density performance to that of conventional based on liquid organic electrolytes. To achieve this goal, solid electrolytes that possess high cation conductivity combined with the adequate electro-chemo-mechanic stability and sustainable, low-cost and large scale processability have to be developed.
In this work, we focus the study on families of chlorines using non-critical elements that can act catholytes due to their high stability at high voltages (>4V). These chlorines have been reported to have very high Li conductivities when disorder is enforced by high energy mechanical milling leading to metastable phases high low thermal stability. This imposes a limitation in the scalability of the production of these systems and in their integration in full devices.
Here, we analyze a range of inorganic and polymer-inorganic solid electrolytes with a combination of experimental and computational techniques, to qualitatively understand the structural and chemical factors affecting the ion mobility in these systems in correlation with their processability. We use a combination of high frequency range (3GHz-mHz) electrochemical testing with solid-state NMR, synchrotron and neutron diffraction studies and FIB-SEM analysis with multiscale simulations to correlate local and long range structural and chemical features with the electrochemical performance. Finally, we propose a highly disorder spinel based on non-critical materials as a potential electrolyte high combined enhanced electrochemical performance and processability.
1.3-O1
As the demand for safer and more sustainable energy storage systems continues to rise, all solid-state batteries (ASSBs) have emerged as a promising alternative to traditional lithium-ion batteries. By replacing liquid electrolytes with solid-state ones, the resulting solid batteries offer the potential for enhanced safety operation and higher energy density, as well as the elimination of harmful and flammable organic compounds. In this line, the development of a green solid-state electrolyte is a fundamental part of the transition to a new generation of solid-state batteries, since solid electrolytes can prevent and minimize the failure mechanisms derived from dendrite growth in lithium-metal batteries, while reducing the amount of critical organics and liquids. Among all the types of solid electrolytes within the inorganic families (NaSICON, LiSICON, garnet, perovskites…), the Li1.3Al0.3Ti1.7(PO4)3 (LATP) a NaSICON-like phosphate, stands out due to its theoretical high ionic conductivity (3 mS cm-1), air and humidity stability and lack of scarce transition metals. [1]
However, ceramic bodies like the LATP have traditionally been processed following solid-state reactions involving high-temperature routes, which result in a high energy consumption and large CO2 emissions. To assess these problems, the Cold Sintering Process (CSP) has emerged as a new sintering route that enables the obtention of dense ceramics like LATP employing a low processing temperature (less than 300 ºC), mid-to-large pressures (hundreds of MPa) and a small amount of a transient liquid phase (TLP).[2] Furthermore, with the CSP it is possible to incorporate polymeric additives to form composites with enhanced electrochemical properties taking advantage of the low operating temperatures. In this way, this new processing technique opens the door for the obtention of new hybrid materials acting as solid electrolytes for the new ASSBs.
In this work, authors present a comprehensive study of the application of the Cold Sintering Process in the manufacture of composite solid electrolytes (CSEs) based on LATP and a 10% PEO2-LiTFSI polymer matrix additive, by focusing on some of the most important processing variables such as the TLP content, the ceramics particle size, the nature of the dopants and the sintering pressure, which will determine the final behavior of the electrolyte in a lithium-metal battery. It is worth mentioning that by optimizing the above-mentioned variables, CSEs with a maximum ionic conductivity of 0.5 mS cm-1, a low activation energy (0.296 eV) and good cycling stability (160.1 mAh g-1LFP at C/10 and 75.8 mAh g-1LFP at 1C) are obtained at a maximum pressure and temperature of 300 MPa and 150 ºC, respectively. Furthermore, in operando electrochemical impedance spectroscopy (EIS) is employed as a powerful characterization technique, since it is not only used during the sintering process as a control tool to monitor the densification, but also during the cycling of CSP-obtained half-cells (with an LFP|CSE|Li structure) to identify potential failure mechanisms derived from the stacking of the layers.
1.3-I1
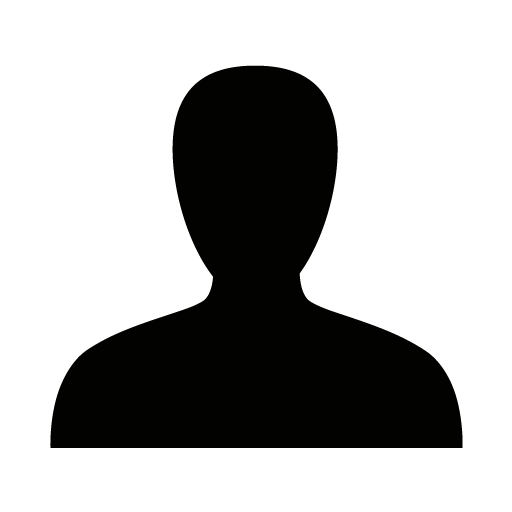
Thin film solid ionic conductors have been identified as a key component for miniaturised all-solid-state sensors and batteries since the 1980’s. Among them, amorphous Li-based materials prepared by vacuum deposition techniques are particularly attractive as electrolytes for making lithium microbatteries and integrating the latter into smart autonomous microsystems. In that respect, lithium phosphorus oxynitride, prepared by reactive sputtering of a Li3PO4 target under a pure nitrogen atmosphere, exhibits a combination of interesting properties such as an ionic conductivity of ~ 2.10-6 S.cm-1 at room temperature, a very low electronics conductivity < 10-14 S.cm-1, and passivating behaviour on lithium metal, which led to the market launch of first microbatteries. Since then, energy density specifications for IoT and medical applications have led to changes in conventional cell design with more stringent requirements for ionic conductivity. In recent years, there has also been renewed interest in these thin-film amorphous electrolytes as part of the development of next-generation batteries for automotive applications. Used in the form of coatings, they can solve reactivity problems at the electrode/electrolyte interface and improve the cycling behaviour of metallic lithium [1].
Amorphisation and then partial nitridation of lithium orthophosphate, leading to the formation of a disordered material containing condensed units with bridging nitrogen [2, 3], was found to be an effective means of boosting ionic conductivity. The introduction of a second glass former, such as in Li-Si-P-O-N, can also provide an additional tenfold increase through a mixed former effect [4], while maintaining exceptional mechanical properties [5]. This evolution illustrates the need for research into materials that are more and more complex in their composition, and not confined to ‘simple’ systems, to identify the best ionic conductors. In this respect, high-throughput experimentation is an attractive approach for screening multi-element compositions [6]. It usually involves a combinatorial synthesis method capable of rapidly producing a large number of samples with different compositions, i.e. material libraries, combined with automated characterisation methods that allow various specific properties to be rapidly measured on all the samples produced.
The development of a specific experimental approach for the high-throughput screening of thin film solid electrolytes based on the synthesis of material libraries by magnetron co-sputtering on 4’’ wafers and the rapid characterisation of their thickness (stylus profilometry), composition (LIBS, ICP-OES), structure (Raman spectroscopy) and conduction properties (Impedance spectroscopy) will be presented [7]. Mapping the composition of these thin films containing low-Z elements, particularly Li, on large substrates is undoubtedly the most difficult stage in this process. Laser-induced breakdown spectroscopy (LIBS) appears to be the most appropriate technique for achieving this objective, as it meets the key requirements of speed, spatial resolution and sensitivity [8]. However, further developments are required to achieve elemental quantification. To this end, combined ICP-OES, LIBS and ion beam (RBS, NRA) analyses were carried out.
Ultimately, screening large parts of the compositional space of Li(Si,P)(O,N) reveals general relationships between composition, local structure and ion transport properties, but also raises questions about the influence of synthesis conditions on the degree of disorder in these amorphous materials
1.3-I2
The nanoscale structure and chemistry of materials and interfaces largely govern the performance of (solid‑state) batteries.[1] Thin film solid-state batteries (TFSSB) have well-defined geometry (parallel layers of known thickness and area) and compact size, making them ideal model systems for studying material and interface properties.[2,3] The thin-film architecture allows for relatively easy investigations of (buried) interfaces and materials that would be challenging to measure in bulk cells that have multi-phase components with often random structure. Here, I will show recent highlights from our work on transition metal fluoride conversion cathodes (TM = Fe, Cr, etc.) for TFSSBs. I will focus on scanning transmission electron microscopy investigations into the nanostructure-performance relationship and its evolution during cycling. Briefly, the performance of TM fluoride cathodes is dependent not only on the choice of the transition metal but also on its nanostructure and mixing of the constituent phases. For example, in Fe-LiF cathodes, we observe electrochemical activation, wherein the particle size of the cathode coarsens and the elemental distribution changes during cycling which is associated with a gradual increase in discharge capacities. I will also talk about the challenges associated with this approach in terms of sample preparation and handling. This work uses the TFSSB system as a model to study fundamental mechanisms and electrochemistry that will ultimately improve bulk conversion cathodes based on transition metal fluorides.
1.3-I3
Julia Amici has a PhD in Material Science and Technology from Politecnico di Torino (Italy), focused on polymers and polymeric coatings. She conducted her Post Doc in the Electrochemistry Group at Politecnico di Torino DISAT, on post Li-ion technologies, in particular Li-Air and Li-Sulfur (Li-S) batteries. She participated to different European and national projects on Li-Air, Li-S and Li-ion systems, preparing, testing and optimizing electrode materials and various electrolytes. She is currently Associate Professor at Politecnico di Torino and her research activities are focused on synthesis and characterization of highly efficient composite polymer electrolytes for Li-ion, Li-air and Li-S batteries. She is the P.I. for Politecnico di Torino in the EU funded projects SUBLIME (H2020) and ADVAGEN (Horizon Europe), both on all solid-state Li-ion batteries. She is actively participating in Battery2030+ initiative (co-author of the Roadmap: “Inventing the Batteries of the Future, Research Needs and Future Actions”) and has been selected as an expert in WG1:” New and Emerging Battery Technologies” of ETIP EBA Batteries Europe Platform. She is author of above 60 publications in international peer-reviewed journals, on materials, Li-ion, Li-Air and Li-S systems and 2 patents.
To decrease carbon dioxide emissions, unprecedented efforts are being undertaken toward the development of efficient and inexpensive electric vehicles and stationary energy-storage systems. Lithium-ion batteries represent an efficient solution, that have transformed personal electronics and enabled the market introduction of electric vehicles. However, the ever-growing energy storage industry imposes great demands that current lithium-ion batteries could hardly satisfy. In this perspective, the use of metallic lithium as anode, both in Li-ion cells and in the so-called “post Li-ion technologies”, would represent the “holy grail” of battery research thanks to its extremely high theoretical specific capacity (3860 mA h g-1), the lowest redox potential (-3.040 V vs the standard hydrogen electrode) and a low gravimetric density (0.534 g cm-3).
However, metallic Li also presents many challenges derived primarily from dendrite formation upon cycling causing both safety issues and poor cycling performance. In addition, liquid electrolytes contain combustible organic solvents that can cause leakage and fire risks during overcharge or abused conditions, especially in large-scale operation. Therefore, replacement of liquid electrolytes with a solid electrolyte has been recognized as a fundamental approach to effectively address the above problems.
Among the solid-state systems under study, polymer-based electrolytes represent a good compromise in term of room temperature ionic conductivity, thermal and electrochemical stability, and above all, interfacial contact. In lithium metal batteries, the preparation of methacrylate-based polymer matrix, in a one pot, solvent free, UV or thermally induced, radical polymerization, is an inexpensive and quick method to obtain versatile membranes [1]. Meanwhile, eventual activation with small amount of ionic liquids can allow to obtain composite electrolytes with outstanding room-temperature conductivities, while preserving the non-flammability of the system thus enhancing the safety [2]. The simplicity of the formulation and the preparation method open the road to highly versatile electrolytes, adaptable in function of the final application [3]. Additionally, the insertion of particular groups in the matrix can introduce self-healing abilities (for example through dynamic hydrogen bonding), further improving the safety features of the cells [4]. Last but not least, bio-derived macromolecules can be functionalized with methacrylate groups to be then directly used as oligomers in the same kind of procedures, obtaining interesting quasi solid state electrolyte systems directly from waste [5, 6, 7], and fully integrating the final device in a circular economy approach.
2.1-I1
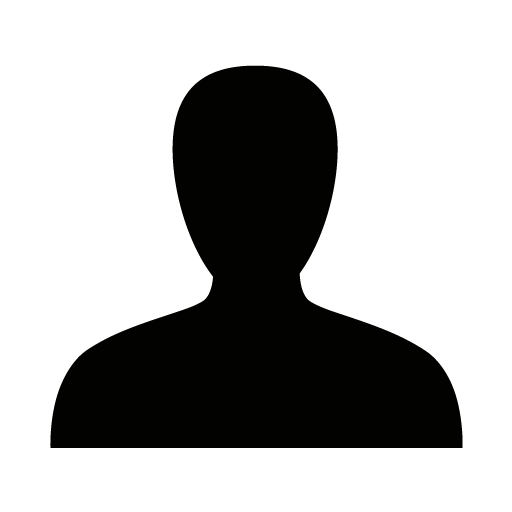
The development of high-performing garnet based all-solid-state batteries requires intensive knowledge of the materials and processes throughout the whole cycle life of the battery to ensure ecological and economical battery production. Life-cycle analysis of the battery production line uncovered the production of raw materials and the temperature treatment steps as the crucial factors. Therefore, a better understanding of the garnet-based ceramic cathodes is needed.
3D analysis and reconstruction of the cells allowed the modelling of realistic microstructures and connects them to experimental data. This realistic cell model facilitates a deeper understanding of the limiting factors towards higher energy densities. Based on these findings, an optimized microstructure was derived. With the introduction of sequential tape-casting and an optimized sintering strategy to mitigate stresses in the material during high-temperature treatments, cathodes with this optimized microstructure were produced. These cathodes show a significant increase in the available areal specific capacity of ceramic batteries.
The introduction of advanced processing methods, as well as significant improvement of the anode side, an analysis of the degradation mechanisms in the cathodes during operation helped uncover the nature of capacity losses during cycling. Based on these findings, a strategy for direct recycling of the ceramic cells was derived, regaining over 40% of the initial capacity after complete degradation.
2.1-O1
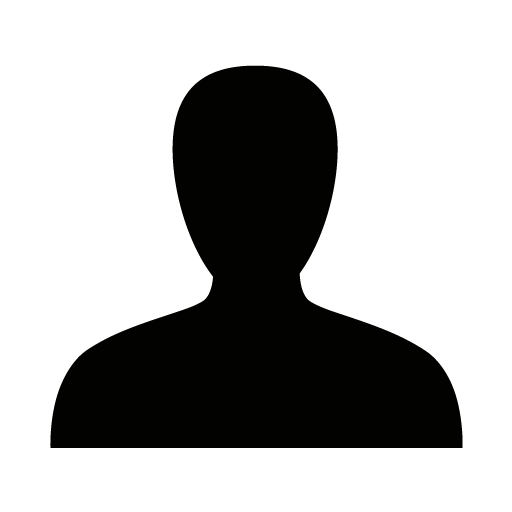
Perspective on Warm Isostatic Pressing for Mass Production of Solid-State Batteries
T. Rabe, Västerås / SE-721 66, M. Dixit, Oak Ridge / TN 37830, C. Beamer, Columbus / OH 43035, J. Shipley Västerås / SE-721 66, J. Fischer Västerås / SE-721 66, Ilias Belharouak / Oakridge National Laboratory 5200, 1 Bethel Valley Rd, Oak Ridge, TN 37830, United States
In recent years, there have been serious commercial advancements with regards to solid-state batteries (SSBs), in which liquid electrolyte is replaced by a solid-state electrolyte (SSE).[1,2,3] Cell concepts with functional layers realizing zero-excess lithium metal anodes were developed, combining overall reduced weight and lithium metal anodes, achieving superior energy density (>1000 Wh/l).[4,5] The need for external pressure, also called “stack pressure”, of SSBs due to cell volume change in operation and subsequent particle contact loss was reduced to a minimum (~2 MPa) with cells based on highly conductive sulfide SSEs densified by warm isostatic pressing (WIP).[5,6] Considering mass production, it seems that the common, uniaxial methods for calendering lead to insufficient composite density and lower electrochemical performance.[7] Most individuals working in battery production are critical towards WIP, because of its batch process characteristic. Ironically, the knowledge about WIPs scaling potential is unknown to the community.[8] Vessel volumes for presses which are already employed in mass production have reached 2000 l and above. With this presentation Quintus Technologies will give the audience at the “MATSUS conference” a summarizing perspective of basics for (warm) isostatic pressing and the status for industrial production of SSBs from an equipment suppliers view.
Literature: [1] J. Janek, W. Zeier, Nat. Energy 2016, 1, 16141. [2] D. H. S. Tan, S. Meng et al., Joule 2022, 6, 1755-1769. [3] J. Janek, W. Zeier, Nat. Energy 2023, 8, 230-240. [4] Y.-G. Lee et al., Nat. Energy 2020, 5, 299-308. [5] W. Choi et al., ACS Appl. Mater. Interfaces 2024, 16, 26066-266078. [6] Y.-T. Chen, S. Meng et al., Adv. Energy Mater. 2024, 2304327. [7] C. A. Heck, Batteries & Supercaps 2024, 7, e202300487. [8] M. Dixit, T. Rabe et al., Device 2024, 100370.
2.1-I2
High-temperature sintering of composite cathodes triggers lithium loss and secondary phase formation, one of the major challenges in oxide-based solid-state batteries. However, the interdiffusion during sintering in the different partial pressures of lithium and oxygen remains poorly understood. Here, we study the reaction mechanism for the formation of impurity phases during the co-firing of Li-garnet (Li7La3Zr2O12) and LiCoO2 at high temperature and propose an alternative processing strategy that enables the production of impurity-free all-oxide composite cathodes. By using Li-rich atmospheres, the secondary phase of LaCoO3 is avoided and the composite cathodes exhibit >95% relative density. The electrical resistance of the composite cathode is increased by 3-4 orders of magnitude. Electronic conductivity of over 1 mS cm-1 is achieved at room temperature. An unprecedented areal discharge capacity of 3.48 mAh cm-2 is realised at current densities of 0.25 mA cm-2, demonstrating the high performance of the cathodes. Our results encourage further demonstration of all oxide solid state battery prototypes in combination with thin oxide electrolytes and lithium metal anodes.
1.1-I3
Perovskite-silicon tandem solar cells are on the brink of commercialization, with efficiencies skyrocketing in the past 5 years, now being the most efficient two-terminal configuration ever made. Yet, the reliability of these devices is still a big question mark. This is in part due to the short time since their development hindering decade-long tests due to sheer time constraints, but also because there is a lack of established accelerated aging protocols due to a lack of understanding of primary failure modes. In the presentation, I will cover our recent results looking at the perovskite top cell and how nanometric defects (1) - how they trigger device degradation and how they can be mitigated – but also at effects on the um- and cm-scale, such as how the choice of the silicon cell texturing affects performance and stability (2), as well as long-distance ionic effects (3,4).
1. Othman et al., Adv. Mater. 2024, accepted
2. Turkay et al., submitted
3. Artuk et al., submitted
4. Jacobs et al., Energy Environ. Sci., 2022,15, 5324-5339
1.1-I1
Metal halide perovskites have demonstrated exceptional properties for various technological applications. However, several challenges still impede their full commercial expansion. From a sustainability perspective, this communication reviews the current status of some of the most significant challenges and, in certain cases, offers potential solutions.
One of the key challenges is the toxicity associated with materials, precursors, and solvents. This issue has gained increased relevance following the publication of the Safe and Sustainable by Design Framework in Europe.[1] The adoption of deposition techniques other than spin-coating presents new difficulties for improving efficiency, but it also offers considerable opportunities for reducing energy consumption and waste from an environmental and economic sustainability perspective. Nevertheless, significant technological optimization is still needed, particularly in the selection of green solvents and their optimal drying and recycling processes.[2]
Techniques such as hot injection and microwave synthesis for producing perovskite nanoparticles also face notable challenges in scaling, enhancing performance, improving energy efficiency, and increasing circularity. Another limiting factor affecting commercialization is the criticality of materials in Europe and other countries, particularly metals like cesium,[3] rubidium, antimony, and bismuth.[4] Beyond their inclusion in official lists, the issue is more complex and intriguing when we consider the dynamics of systems, including global resource availability and the geopolitical factors that influence them.
Additionally, we present findings from environmental impact studies employing life cycle analysis methodology on metal halide perovskites, highlighting unresolved issues related to inventory shortages and deficiencies, the lack or obsolescence of toxicity characterization factors for certain substances (such as metals and solvents), and the unknown behavior of nanoparticles in ecosystems and their effects on human health.
Finally, the silicon photovoltaic technology has yet to resolve the issue of what to do with solar panels at the end of their life cycle. We should not allow metal halide perovskites to face the same fate. We still have the opportunity to adopt circular practices from this early stage of research, including mechanisms like recent digital product passports.
This presentation aims to stimulate discussions, exploring innovative solutions and collaborative efforts to address these pressing challenges in the sustainable development of perovskite technologies.
1.1-I2
Since 2019, Yana Vaynzof holds the Chair for Emerging Electronic Technologies at the Technical University of Dresden. Prior to that (2014-2019), she was a juniorprofessor in the Department of Physics and Astronomy, Heidelberg University (Germany). She received a B.Sc degree (summa cum laude) in electrical engineering from the Technion - Israel Institute of Technology (Israel) in 2006, and a M.Sc. degree in electrical engineering from Princeton University, (USA) in 2008. She pursued a Ph.D. degree in physics under the supervision of Prof. Sir. Richard Friend at the Optoelectronics Group, Cavendish Laboratory, University of Cambridge (UK), and investigated the development of hybrid polymer solar cells and the improvement of their efficiency and stability. Upon completing her PhD in 2011, she joined the Microelectronics group at the University of Cambridge as a Postdoctoral Research Associate focusing on the research of surfaces and interfaces in organic and hybrid optoelectronics. Yana Vaynzof was the recipient of a number of fellowships and awards, including the ERC Starting Grant, Gordon Y. Wu Fellowship, Henry Kressel Fellowship, Fulbright-Cottrell Award and the Walter Kalkhof-Rose Memorial Prize.
In recent years, remarkable progress has been made in the field of metal halide perovskite solar cells, resulting in power conversion efficiencies (PCE) surpassing 26%. These advances are made possible by compositional and additive engineering of the perovskite active layer and the development of more efficient charge extraction layers. Most research efforts are dedicated to processing perovskites from solution, often from highly toxic solvents such as dimethylformamide (DMF). While this is manageable for lab-scale fabrication, using such solvents raises considerable safety and environmental concerns about fabricating perovskite devices on a mass scale. To solve this challenge, green solvents suitable for perovskite processing must be identified, and perovskite deposition processes must be adapted to the properties of the new solvents. Alternatively, solvent-free deposition methods must be developed that are compatible with industry processing on a large scale. In this talk, I will present promising green solvent alternatives to DMF and highlight recent developments in vapor-based deposition methods of metal halide perovskites.
1.2-O1
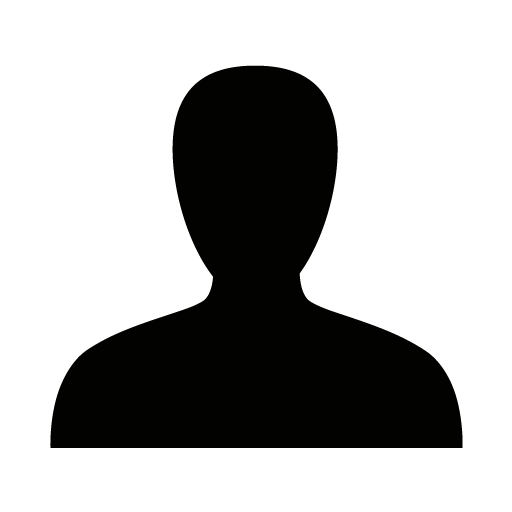
Today, hybrid halide perovskite solar cells show high power conversion efficiencies (PCE >25%), but volatile organic cations (MA+, FA+) can limit their thermal stability. Therefore, inorganic perovskites such as CsPbI3 appear as promising materials for perovskite PV devices [1]. In addition, their band gap, around 1.7 eV, is close to the ideal one for perovskite-on-silicon tandem applications. The inorganic perovskites are considered as good candidates for the development of stable and efficient single junction and tandem solar cells.
Despite many obvious advantages, the PCEs achieved with inorganic CsPbI3 perovskites remain lower than those of their hybrid counterparts. The difference is mainly due to the difficulty of synthesising a stable CsPbI3 perovskite phase. According to Goldschmidt tolerance factor calculations, CsPbI3 is just at the limit of forming a stable perovskite phase due to the relatively small size of Cs+ cations compared to MA+ or FA+ cations. Therefore, the simultaneous formation of non-perovskite phases is often observed during the synthesis of CsPbI3.
Here, we present our strategy to develop inorganic perovskite as an absorber, deposited by slot-die coating in ambient atmosphere, a relevant industrial large area deposition technique that has not yet been explored for this type of perovskite.
Firstly, we focused our attention on the ink preparation to obtain stable inorganic perovskite films. After investigating several additive strategies, dimethylammonium iodide (DMAI) [2] was chosen to stabilise CsPbI3. Several parameters of the precursor solution such as the additive concentration and solvent ratio were further optimised. Furthermore, the composition was tuned by adding bromide [3]. Secondly, using this ink, the deposition of the inorganic perovskite thin film by slot-die coating, in ambient atmosphere, was optimized. Deposition parameters were refined based on morphological, structural, and optical characterizations of the deposited inorganic perovskite layers. Uniform thin films with a surface area of up to 5x10cm2 were obtained (Fig.1).
Finally, to test our developed thin films, we implemented the optimised inorganic perovskite absorber in solar cells. A PCE of the same order of magnitude as the PCE obtained for solar cells deposited by spin-coating in ambient atmosphere on a small surface area of 2.5x2.5cm2 was obtained on 5x10cm2 (Fig.2). After further optimisations, we obtained a maximum power conversion efficiency of 12.7% for solar cells containing inorganic perovskite deposited by slot die coating in ambient atmosphere.
1.2-I1
Iván Mora-Seró (1974, M. Sc. Physics 1997, Ph. D. Physics 2004) is researcher at Universitat Jaume I de Castelló (Spain). His research during the Ph.D. at Universitat de València (Spain) was centered in the crystal growth of semiconductors II-VI with narrow gap. On February 2002 he joined the University Jaume I. From this date until nowadays his research work has been developed in: electronic transport in nanostructured devices, photovoltaics, photocatalysis, making both experimental and theoretical work. Currently he is associate professor at University Jaume I and he is Principal Researcher (Research Division F4) of the Institute of Advanced Materials (INAM). Recent research activity was focused on new concepts for photovoltaic conversion and light emission based on nanoscaled devices and semiconductor materials following two mean lines: quantum dot solar cells with especial attention to sensitized devices and lead halide perovskite solar cells and LEDs, been this last line probably the current hottest topic in the development of new solar cells.
Halide perovskite solar cells have revolutionized the photovoltaic field in the last decade. In a decade of intensive research it has been a huge improvement in the performance of these devices, however, the two main drawbacks of this system, the use of hazardous Pb and the long term stability, still to be open questions that have not been fully addressed. Sn-based perovskite is the most obvious alternative to Pb, producing degradation materials less toxic and presenting the highest performance among the different Pb-free halide perovskites, but presenting a lower stability than their Pb-containing counterparts. In this talk, we highlight how with the proper additives and encapsulation Sn-based halide perovskites can increase significantly their stability allowing their efficient application in tin iodide perovskite solar cells. Nevertheless, as in the case of Pb-based halide perovskite the potential applications of tin-perovskites is not limited to photovoltaic devices. In addition, we report the use in Sn-based LEDs, photodetectors and to drive photocatalytical reactions.
1.2-I2
The commercial success of perovskite solar cells (PSCs) depends on transitioning from lab-scale fabrication to high-throughput, low-cost manufacturing. Roll-to-roll (R2R) processing presents an ideal route, offering continuous production with reduced material waste. However, the shift from batch processing to fully inline deposition introduces challenges in coating uniformity, interlayer compatibility and defect control. These issues must be addressed to unlock the full potential of perovskites for industrial-scale photovoltaics.
This talk will provide a broad overview of R2R deposition strategies for PSCs, focusing on SnO₂ electron transport layers, PEDOT and SAM-based hole transport layers, perovskite absorber layers, P3HT, and solution-processed carbon electrodes. A key emphasis will be on scalable approaches to layer deposition, particularly slot-die coating, and how solvent engineering and interfacial adhesion strategies can overcome the challenges of high-speed perovskite module fabrication. Recent advancements in fully solution-processed electrode deposition will also be discussed, particularly the replacement of evaporated metal contacts with carbon-based alternatives, demonstrating a pathway toward fully printed, low-cost, and highly stable PSCs.
Looking ahead, building on our existing work on registration-based interconnects, we aim to explore laser-patterned interconnects as a route to improving module performance while reducing processing complexity. The next steps also include integrating real-time quality control and inline lamination of encapsulation layers to further enhance the scalability and long-term stability of R2R perovskite module manufacturing. These developments will be critical for bridging the gap between laboratory-scale advances and commercially viable roll-to-roll perovskite solar production.
1.2-O2
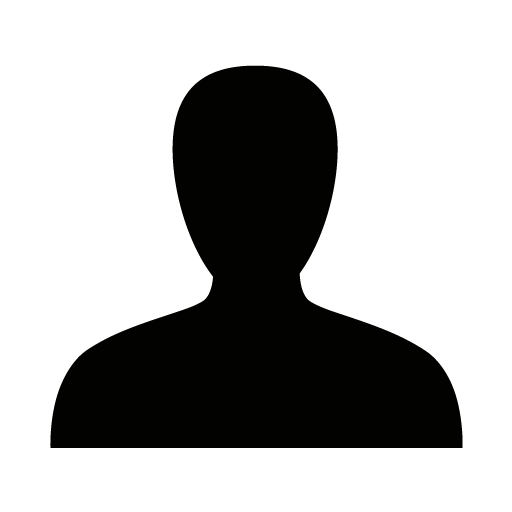
One of the major drawbacks of lead halide perovskites and an obstacle to their commercialization is their lack of long-term chemical and structural stability. When exposed to external stressors, these materials either chemically degrade or structurally transform into other crystal phases. Understanding the end-of-life mechanisms of perovskites is essential for developing better strategies to prevent their degradation and enable reversible process for re-manufacturing thin films and devices. I investigated the synergistic role of humidity and oxygen in the degradation pathway of lead halide perovskites used in solar cells. This was achieved using synchrotron-based in-situ grazing-incidence wide-angle X-ray scattering (GIWAXS) and X-ray photoelectron spectroscopy (XPS) (Hidalgo et al., J. Am. Chem. Soc. 2023). This initial work highlighted the interplay between structural changes and surface chemical reactions in lead halide perovskites, leading to the evaluation of a surface passivation strategy and compositional engineering, resulting in more durable lead halide perovskites for photovoltaic applications.
1.2-O3
Perovskite solar cells (PSCs) hold great potential as technology for the next generation of thin-film photovoltaics. However, industrial-scale fabrication processes must be significantly improved for PSCs to become commercially viable. Scalable solution deposition techniques, such as slot-die(SD)-coating, are difficult to control during the entirety of the perovskite thin-film formation, because of complex fluidics, drying, and crystallization dynamics during high solvent removal. Drying with a narrow gas-purged slot-jet is an established drying method that provides a sufficiently high solvent mass transfer to remove the solvent from the wet thin-film, inducing a prompt nucleation. In prior studies, a model on drying dynamics of perovskite thin-films enhances the understanding of the complex drying process, analyzing impacts of gas-flow velocity and web speed from a fundamental view point[1;2]. Moreover, we validated the model on large-area substrates, predicting drying windows by in-situ monitoring of SD-coated thin-film drying processes[3]. The results lead to concrete rectification work on the drying procedure, enhancing the optimally dried area. However, slot-jet-drying still comes with inhomogeneous fluidic dynamics and disturbed gas flows, causing unintended fluidic backflows, non-uniform drying patterns and severe edge effects, impeding progress for perovskite/silicon tandem solar cells (TSCs). Hence, there is need to investigate and optimize the formation of polycrystalline perovskite thin-films fabricated via scalable deposition methods.
In response, we address the necessity for precise drying conditions, enhancing the quality of perovskite thin-films through i) a novel convection-drying method, advancing the homogeneity of the drying process of SD-coated solution thin-films on the entire area, and ii) an adjusted material composition strategy for 2-step processed SD-coating, leading to higher operational stability. Specifically, we introduce a gas-assisted-drying system that provides uniformly distributed drying rates for SD-coated, 2-step processed triple-halide perovskite wet thin-films. Precisely defined drying conditions are established using a stationary, gas flow-controlled two-dimensional nozzle array pattern, consisting of impinging jets and surrounded by suction holes for local solvent removal. This design enables precise control of drying rates. The specific drying system used in this work is inhabited in an in-house glovebox system, meaning the drying circle is fully integrated into the inert atmosphere.
We systematically evaluate and compare the implemented novel drying strategy with established slot-jet-drying by fabricating SD-coated PSCs with alternative drying approaches over an area of 100 cm2 with all scalable techniques. Remarkably, we successfully demonstrate the correlation of applied process parameters at exact sample positions with the resulting thin-film morphology, grain size distribution, and optoelectronic properties, resulting in PSCs with efficiencies up to 19.6% and high PCE-yield of >90% on the entire substrate. We validate an enhanced area utilization with 5x5 cm2 solar mini-modules on the quadrants of 10x10 cm2 substrates, each reaching a PCE ≥17.6% (17.7% ±0.2%). The results imply high homogeneity during the coating and drying processes, with only minimal relative upscaling loss due to module scribing and interconnection resistances, outlining the relevance of our scaling methodology. Consequently, we employ the drying method to a material composition suitable for tandem applications (Eg ~1.68 eV) based on a work of Pappenberger et al[4]. We demonstrate TSCs with PCEs of up to 24.6%, fabricated with all scalable techniques. Particularly, the successful fabrication of TSCs (mean PCE ~24.0%) with a homogeneous PCE distribution of ±0.7%, confirms the importance of a systematically controlled drying technique within an optimized 2-step process. This work underlines the necessity of precise control as well as an understanding of drying dynamics being vital to facilitate large-scale solution-based high-quality optoelectronic thin-films consistently.
Our full research paper“Spatially Regulated Gas Flow Control for Drying of Large Area Slot-Die-Coated Perovskite Thin-Films”by Kristina Geistert et al. is in preparation.
1.3-I1
Metal halide perovskites are gaining attention in detectors of high-energy photons, showing potential in thin-film dosimetry as well as imaging, the latter when prepared as single crystals. Typically, perovskites are made from solutions of the halide salts precursors, which might represent a challenge towards scalability and substrate integration. Here we will discuss dry synthetic methods for the fabrication of perovskite photodetectors. Perovskite thin-film detectors can be made using physical vapor deposition (PVD), an established industrial method. PVD allows for high-purity films with controlled thickness and easy integration into multilayer devices. We present PVD-based detectors with low dark and noise currents, stable reverse bias, and X-ray sensitivity >30 μC/Gy/cm². For thick perovskite materials, we introduce a dry method to prepare free-standing perovskite-polymer composite disks, tunable from 25 to 250 μm in thickness. These composites can be laminated onto rigid or flexible substrates, creating functional detectors with high environmental stability and promising limit of detection.
1.3-I2
Silvia Colella is a researcher at the National research council, CNR-NANOTEC, in Bari, Italy. She received her PhD in “Nanoscience” at National Nanotechnology Laboratory in Lecce (Italy), in 2010. She has been visiting student in the group of professor Luisa De Cola at the Westfälische Wilhelms-Universität of Münster (Germany), where she dealt with the synthesis and photophysical characterization of electroluminescent metal complexes. In 2010 she joined BASF – The Chemical Company (Strasbourg) with a Marie Curie fellowship as experienced researcher in the frame of the EU project ITN SUPERIOR, working on Dye Sensitized Solar Cells. She continued as post-doc researcher at the Institut de science et ingénierie supramoléculaires (ISIS) in Strasbourg, France. In 2012 she started her independent research in Lecce (Italy) at the University of Salento in collaboration with CNR-NANOTEC, the team focused on the conception and optoelectronic characterization of innovative optoelectronic devices based on hybrid halide perovskites. Many high impact publication were produced in this time interval, among them one of the first report in halide perovskite for PV exploitation (Colella et al, Chemistry of Materials, 2013 25, 4613-4618).
Silvia Colella is author of >70 peer-reviewed publications in renowned international journals (including Energy and Environmental Science, Advanced Materials, ACS Energy Letters).
Her scientific production led to >3000 total citations and a h-index of 28 (https://scholar.google.it/citations?user=S2TZd_4AAAAJ&hl=it; https://www.scopus.com/authid/detail.uri?authorId=24170650100).
Metal halide perovskite (MHPs) solar cells represent a promising newcomer in the front of emerging photovoltaic technologies, therefore a potential player to challenge the dramatic energy crisis and climate change that we are facing. The exceptional properties of MHPs derive from their hybrid organic-inorganic nature, which allows also for low-cost and straightforward processing. Solar cells containing MHPs as absorbing layer have already achieved a power conversion efficiency above 26,5 %, close to the efficiency of silicon-based devices. Nevertheless, a major limitation, still preventing the uptake of the technology, is related to the reduced stability of these devices when exposed to operative conditions, namely temperature, light, and moisture. Herein, an effective defect passivation of MHP surfaces is a key strategy to tackle both the stability and the enhancement of solar cell performances. Although many solution-based approaches have been tested, we have explored in the last years an innovative use of plasma, as a solvent-free, scalable, industrially available and non-invasive processing to enhance MHP solar cells performances [1]. The effect of cold plasmas fed by gases as Ar, , and both on lead-based (MAPbI3) [2] and lead-free (FASnI3) [3] perovskites was investigated in terms of optochemical and morphological modifications and correlated to the performance of the photovoltaic devices. An interesting improvement in power conversion efficiency (PCE) was observed for the Ar-treated MAPbI3 perovskites, ascribed to a modulation of surface defects, while a newsworthy suppression of the intrinsic tendency of tin (II) to oxidize to tin (IV) was obtained for the N2-plasma treated FASnI3 perovskites. Starting from these encouraging results, further surface plasma processes have been investigated [4], among which the promising treatment with sulfur-containing molecules, proved to obtain a good passivation of surface defects, through the formation of Pb-S bonds, allowing the improvement of both the fill factor and the PCE of the investigated solar cells, opening new applicability scenarios for these plasma-based processes [5].
References:
[1] V. Armenise, S. Covella, F. Fracassi, S. Colella, and A. Listorti, ‘Plasma‐Based Technologies for Halide Perovskite Photovoltaics’, Solar RRL, 2024, doi: 10.1002/solr.202400178.
[2] A. Perrotta et al., ‘Plasma-Driven Atomic-Scale Tuning of Metal Halide Perovskite Surfaces: Rationale and Photovoltaic Application’, Solar RRL, 2023, doi: 10.1002/solr.202300345.
[3] S. Covella et al., ‘Plasma-Based Modification of Tin Halide Perovskite Interfaces for Photovoltaic Applications’, ACS Applied Material & Interfaces, 2024, doi: 10.1021/acsami.4c09637.
[4] V. Armenise, S. Colella, A. Milella, F. Palumbo, F. Fracassi, and A. Listorti, ‘Plasma-Deposited Fluorocarbon Coatings on Methylammonium Lead Iodide Perovskite Films’, 2022, doi: 10.3390/en15134512.
[5] S. Covella et al., manuscript in preparation.
1.3-O1
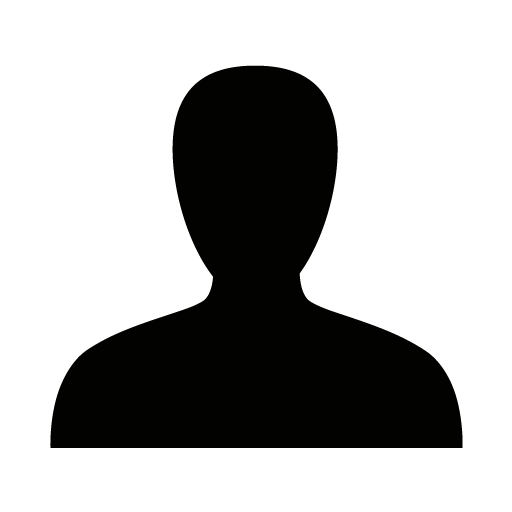
In the past decade, the efficiency of lead halide perovskite-based solar cells has reached levels comparable to those of established silicon solar cells. This advancement makes perovskite solar cells (PSCs) particularly promising for future commercial applications. However, challenges such as long-term stability and scalability still need to be addressed before they can be commercially viable. Additive engineering is one approach that holds the potential for overcoming these challenges.
In this study, we presented a novel additive engineering approach for the formation of FAPbI3 perovskite layers by vapor deposition. The approach is based on a two-step deposition process in which PbI2 is vaporized together with additives. We found using SEM, XRD, UV-vis, and XPS that the additives significantly improve the efficiency of the precursor conversion process to FAPbI3 and lead to an improved microstructure of the perovskite films. When integrated into p-i-n solar cells without interfacial modifications, FAPbI3 layers achieve an efficiency of 18.34%, which is significantly superior to devices fabricated without additive engineering.
1.3-O2
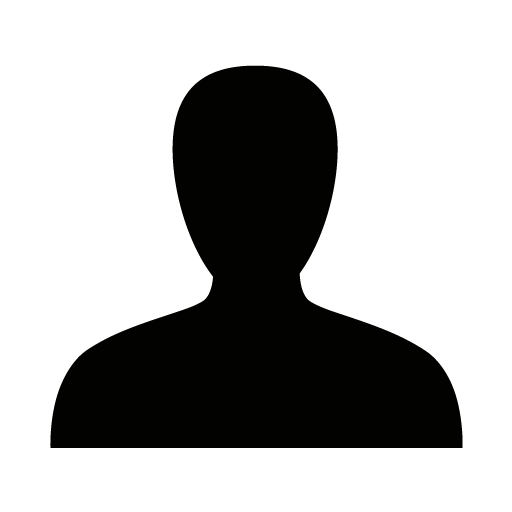
Metal halide perovskite semiconductors have shown significant potential for use in photovoltaic (PV) devices. While fabrication of perovskite thin-films can be achieved through a variety of different techniques, thermal vapour deposition is particularly promising, allowing for high-throughput fabrication and large-scale production[1]. However, the ability to control the nucleation and growth of these materials, particularly at the charge-transport layer/perovskite interface, is critical to unlocking the full potential of vapour-deposited perovskite PV[2].
In this study, we explore the use of a templating layer to control the growth of co-evaporated perovskite films, and find that such templating reproducibly leads to highly oriented films with identical morphology, crystal structure, and optoelectronic properties, independent of the specific substrate on which the perovskite was deposited[3]. When incorporated into solar cells, devices based on this approach showed reproducible improvements, yielding vapour-deposited FA0.9Cs0.1PbI3-xClx solar cells with steady-state solar-to-electrical power conversion efficiencies over 19.8%. Our findings provide a straightforward and reproducible method of controlling the charge-transport layer/perovskite interface in vapour-deposited perovskite solar cells, further clearing the path toward large-scale fabrication of efficient perovskite optoelectronic devices.
1.3-O3
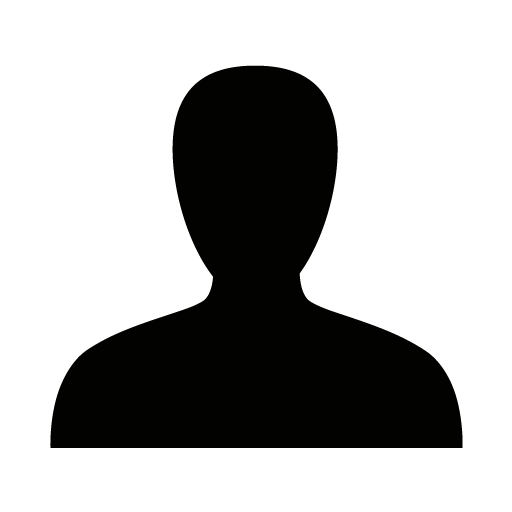
In this work, we analyse the photoemission of lead halide perovskite quantum dots synthesized within nanoporous metal oxide films with either electron scavenging (such as TiO2) or electron blocking (SiO₂) properties as a function of the excitation power density. In good agreement with previous reports [1-3], at low excitation fluences, scaffolds that facilitate charge extraction from the perovskite electronic bands, such of TiO2, give rise to a lower emission intensity and quantum yield (QY) with respect to more insulating ones like SiO2. Counterintuitively, the situation reverses under higher irradiation fluences, for which electron scavenging scaffolds favours a highly intense amplified spontaneous emission (ASE) from perovskite quantum dots. This effect is understood by analysing the interplay between the efficiency of carrier transfer to the matrix and the different electronic processes occurring at each irradiation stage, i.e., trap-assisted and band-to-band recombination at lower and intermediate fluences, and the competition between Auger recombination and population inversion at higher ones. These findings open the path to develop highly efficient light emitting perovskite QD films, leading future progress for their potential application in advanced photonic devices.
1.3-O4
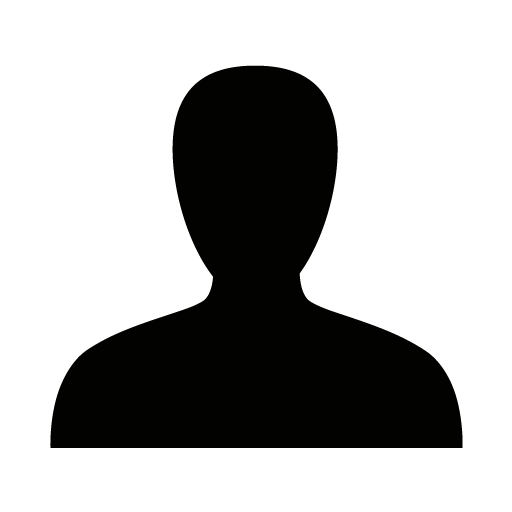
Water exposure is typically associated with adverse effects on the structural integrity and photoluminescence of lead halide perovskites, often hindering their performance in optoelectronic applications. Nevertheless, we report a humidity-induced process for the in situ synthesis of CsPbBr₃ nanocrystals (NCs) within a magnesium acetate matrix, achieving an outstanding near-unity photoluminescence quantum yield (PLQY).
Moreover, the process is facilitated by the controlled introduction of water in combination with pH modulation via an acetic acid/acetate buffer system. This setup generates hydroxide ions (OH⁻), which passivate electronic trap states within the CsPbBr₃ NCs, further enhancing their optical stability and efficiency.
By transforming water exposure from a destructive factor into a beneficial tool, this method represents a novel paradigm in perovskite chemistry as an advanced strategy to improve the stability and performance of lead halide perovskites through precise water and pH control.
Our approach showcases remarkable optical properties, boasting a near-unity PLQY alongside exceptional reproducibility and repeatability under low-demanding process conditions. Its greatest advantage lies in its exceptional versatility and compatibility with high-throughput roll-to-roll printing techniques, enabling the fabrication of cost-effective, large-area, and high-performance devices. As a result, this approach has the potential to significantly reduce costs, improve sustainability, and expand applications across various light-emission technologies, such as down-conversion and gas sensing.
2.1-I1
Antonio Guerrero is Associate Professor in Applied Physics at the Institute of Advanced Materials (Spain). His background includes synthesis of organic and inorganic materials (PhD in Chemistry). He worked 4 years at Cambridge Dispaly Technology fabricating materiales for organic light emitting diodes and joined University Jaume I in 2010 to lead the fabrication laboratory of electronic devices. His expertise includes chemical and electrical characterization of several types of electronic devices. In the last years he has focused in solar cells, memristors, electrochemical cells and batteries.
Ion migration in halide perovskites and its relation with the external contacts has very important implications in solar cells, photodetectors, X-ray detectors and memristors.1 Ion migration poses a negative effect in some optoelectronic applications controlling the hysteresis and the long term stability. Here we discuss the effect of the dimensionality of the crystalline structure of halide perovskites in relation to the ion migration and the connection with stability of solar cells.1 Alternatively, the stability can be enhanced by an adequate selection of the external contacts to be compatible with the migrating ions. On the other hand, the ionic conductivity of halide perovskite is responsible for a memory effect that can be used in resistive memories expanding the applications for this type of materials. Several configurations are evaluated in which structural layers are modified systematically: formulation of the perovskite including 2D perovskites,2 the nature of the buffer layer3 and the nature of the metal contact4. We show that in order to efficiently promote migration of metal contact the use of pre-oxidized metals greatly enhance the performance of the memristor and reduces the energy requirements. Importantly, these halide perovskite devices show potential in both volatile and non-volatile memristive devices that find applications in neuromorphic computing.5 Overall, the interplay between migrating ions and chemical interactions with the contacts can be extrapolated to the different optoelectronic devices fabricated with halide perovskites.
2.1-I2
Ivan Scheblykin obtained Ph.D. in 1999 from Moscow Institute of Physics and Technology and Lebedev Physical Institute of Russian Academy of Sciences on exciton dynamics in J-aggregates. After a postdoctoral stay in the KU Leuven, Belgium, he moved to Sweden to start the single molecule spectroscopy group at the Division of Chemical Physics in Lund University where he became a full professor in 2014. His interests cover fundamental photophysics of organic and inorganic semiconductors and, in particular, energy transfer, charge migration and trapping. The general direction of his research is to comprehend fundamental physical and chemical processes beyond ensemble averaging in material science and chemical physics using techniques inspired by single molecule fluorescence spectroscopy and single particle imaging.
Material and technological research in neuromorphic computing has garnered significant attention in recent years, with numerous examples of memristors serving as simple electrical synapses. In addition to electrical input and output, light is increasingly used as both an input and output signal in optical memristors, where light transmission is modulated.
In my talk, I will introduce a new concept for an optical memory device based on photoluminescence, termed the "memlumor"—a luminophore with memory.[1] The photoluminescence quantum yield of a memlumor "remembers" the history of previous excitations through parameters governing its photophysics and photochemistry. Metal halide perovskites have proven to be highly promising materials for memlumors. We demonstrated the synergetic coexistence of both volatile and non-volatile memory effects in perovskites over a broad timescale, ranging from nanoseconds to days.[1] We identified the origin of this complex response as the phenomena of photodoping and photochemistry triggered by light input, both of which are closely tied to defect states and their photoinduced dynamics.[2] Remarkably, defects and their temporal dynamics—typically viewed as detrimental to device performance—are essential for memlumor operation.
I will showcase the memlumor properties of CsPbBr₃, which exhibits memory effects over timescales from nanoseconds to minutes, with switching energy as low as 3.5 fJ. Additionally, I will discuss a novel method of multi-pulse time-resolved photoluminescence,[3] specifically suited to study trapped charge carriers in luminescent semiconductors and memory effects induced by them.
Memlumors, as novel optical dynamic computing elements, have a potential to provide a new dimension to existing optical technologies, paving the way for their application in photonic neuromorphic computing.
2.1-O1
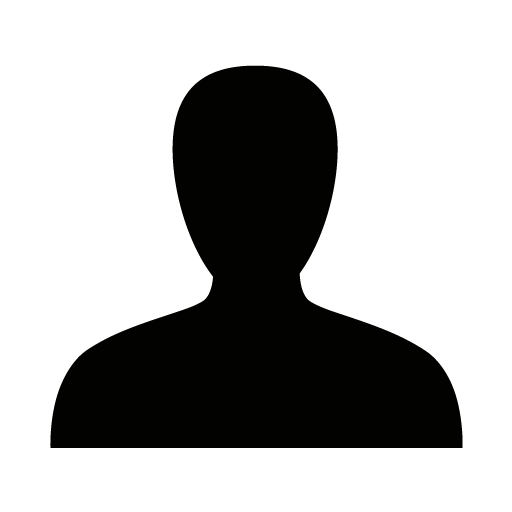
Halide perovskite memristors exhibit excellent properties for neuromorphic computing including analog resistive switching, endurance, and low power requirements. However, the reproducibility and stability are limiting factors for the practical application of these devices. Recently, our research group demonstrated that introducing an interfacial buffer layer between the metal contact and the perovskite active layer can improve the stability of the halide perovskite memristors and even modify the activation mechanism [1, 2]. Nevertheless, the mechanisms through which these devices work remain unclear, and a deeper understanding is required to achieve a rational optimization.
In this work, we present the fabrication of highly reproducible memristors using Ag as active electrode, a highly stable perovskite formulation (MAPbBr3), and a buffer layer containing oxidized silver (AgI). These memristors show high reproducibility in fabrication and stability (>104 cycles of pulse trains) [3]. This highly reliable system enabled an in-depth study of the mechanisms operating in perovskite memristors, which provided insights into the nature of the dual volatile and nonvolatile responses. Short-duration pulses at the activation voltage lead to a two-state volatile response due to the formation of an ionic double layer close to the contacts, which returns to the initial state once the bias ceases. In contrast, long-duration pulses lead to a gradual increase in current and a nonvolatile response caused by the appearance of a chemical inductor. The observed multi-state nonvolatile regime is related to the formation of Ag+ conductive filaments and provides suitable conditions for analog computing. Overall, we provide a clear understanding of the nature of these two operating regimes in halide perovskite memristors and show the tools to investigate them in other systems.
2.1-O2
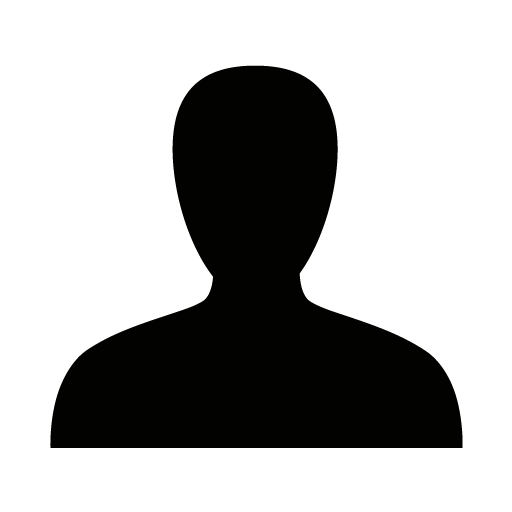
Polarizers are ubiquitous components in modern optoelectronic devices including displays and photographic cameras. However, achieving efficient control of light polarization remains an unsolved challenge. The main drawback of the existing display technologies relies on the substantial optical losses due to the use of polarizers for generating polarized light. In this context, organometal halide perovskite (OMHP) nanostructures offer a promising solution owing to their tunable optical properties—including adjustable bandgap, photoluminescence, and efficient light emission with minimal non-radiative recombination.[1–3] Their outstanding electrical properties have elevated hybrid perovskites as the material of choice in photovoltaics and optoelectronics. Among the different OMHP nanostructures, nanowires and nanorods have lately arisen as key players in controlling light polarization for lighting or detector applications.
In this work,[4] we propose applying an evolved version of the vacuum technique Glancing Angle Deposition (GLAD) as an advanced alternative to synthesising anisotropic-supported OMHP nanostructures. Our approach is a two-step fabrication procedure consisting of the room temperature deposition of PbI2 at glancing angles, followed by deposition of CH3NH3I at normal incidence (0º). As a result, highly anisotropic perovskite nanostructures resembling “nanowalls” have been fabricated. Such alignment degree endows the samples with anisotropic optical properties such as UV-visible absorption and photoluminescence. Moreover, their implementation in n-i-p solar cells can be used to develop self-powered polarization-sensitive photodetectors along the visible range. Thus, the use of Glancing Angle Deposition yields OMHP nanostructures with high anisotropy, controlled microstructure, and thickness, demonstrating potential for scalable, large-area fabrication. This technique is fully compatible with microelectronic and optoelectronic processing methods including CMOS and roll-to-roll technologies, which opens the path towards developing tuneable anisotropic optoelectronic devices based on OMHP.
2.2-I1
Dr. Clara Aranda Alonso, received her doctorate degree in Science from University Jaume I in 2019 at the Institute of Advanced Materials (INAM) (Castellón, Spain). She worked as postdoctoral researcher at the Forschungszentrum Jülich and Institute for Photovoltaics (ipv) at the University of Stuttgart (Germany) for two years. Then she moved to the Institute of Materials Science (ICMUV) at the University of Valencia (Spain) as a Margarita Salas fellow. Currently, she is working at Universidad Pablo de Olavide in Seville (Spain). Her work is focused on the synthesis and characterization of wide band gap perovskite materials, both in thin film and single crystal configuration, for photoconversion devices such as solar cells, photodetectors and memristors, using impedance spectroscopy as the main characterization tool.
Hybrid-halide perovskites exhibit a unique combination of electronic and ionic characteristics, with the latter posing significant challenges to understanding and controlling the operational mechanisms of perovskite devices. The movement of ions within these materials can lead to issues such as recombination, photovoltage losses, and instability. To address these detrimental effects, various strategies have been employed with notable success. However, the fundamental mechanisms underlying these strategies often remain obscured.
A key distinction between electronic and ionic charges lies in their temporal dynamics: ionic responses are significantly slower, involving processes that occur over extended time frames. Impedance spectroscopy (IS) spans a wide range of time scales, enabling the separation of contributions from different components and processes within the device. In fact, at lower frequencies, IS can yield valuable insights into ion dynamics and their influence on electronic performance by quantifying resistances and capacitances. A notable feature often observed in this frequency range is the "loop" or inductive behaviour. Although the precise origin of this phenomenon is still under debate, its associated time constant can reveal crucial information about the kinetics at the interfaces and their effects on the bulk material.
This contribution will focus on quantifying this time constant and its implications for understanding perovskite device performance. We will explore the relationship between this inductive behaviour, the mechanisms behind a record photovoltage of 1.65 V achieved by a bromine-based perovskite solar cell (PSC), the role of a self-assembled monolayer in enhancing the stability and open circuit potential of a PSC, and insights into the electroformation process of a perovskite-based memristor. These examples illustrate how impedance spectroscopy can offer crucial insights to untangle the complexities of the perovskite puzzle and address its technological challenges.
2.2-I2
The conceptual idea of the integration of energy photovoltaic (PV) generation and storage systems in a single unit, the photocapacitor, offers an innovative approach to manage energy supply for IoT systems that typically requite low power and work under discontinuous illumination, improving the system performance, reducing device size and weight. Several photovoltaic technologies (Silicon, Dye Sensitized, Organic and Perovskite solar cless) and type of supercapacitor have been integrated in four, four, three and two terminal photocapacitor, leading so far to a maximum device efficiency of 25% for a perovskite based photocapacitor measured under 1 sun illumination [1].
In this presentation I will introduce the concept of photocapacitor, highlighting the working principles, the characteristic features and potentiality of this type of device. I will then show our work on the realization of perovskite based photocapacitors both on rigid and on flexible substrates.
In particular I will report on the all printed three-terminal photocapacitor based on carbon perovskite solar cell (CPSC) integrated with an MXene-based in plane microsupercapacitor (MSC) realized on glass substrate. The device was characterized both under 1 sun and at 1000 lux illumination achieving an overall efficiency of 3.8% with a striking 99% coulombic efficiency and 87% capacitance retention over 6000 cycles for the supercapacitor unit.
I will then show the integrated two terminal flexible photocapacitor, where a flexible perovskite solar module was integrated with flexible interdigitated supercapacitors interconnected in parallel and series with peak overall and storage efficiencies of 2.8% and 23% respectively. Finally I will show some applications of those devices [2].
2.2-O2
Dr. M. E. Calvo is Doctor in Chemistry by the University of Buenos Aires, Argentina. He is member of the permanent staff of the Spanish National Research Council (CSIC). He is part of the Multifunctional Optical Materials Group at the Institute of Materials Science of Seville where he has been working for 15 years. Nowadays, his main research interest is focused on the solution process of optical porous hybrid nanostructured materials for different applications like solar protection, optoelectronic devices and sensors. He is author of more than 70 publications and 7 patents and he is/was supervisor in several PhD theses. More information at http://mom.icmse.csic.es/group-members/mauricio-calvo/
The synthesis of ABX3 quantum dots (QD) using a mesoporous material with a narrow pore size distribution is a preparation method that has gained interest in recent years due to the ease of processing and the excellent optical properties of the QDs obtained in the pore network.[1] These ligand-free QDs also present a pristine surface that is suitable for interacting with the surrounding atmosphere. In this work, we have synthesized CsPbBr3 QDs within the pore network of high optical quality SiO2 porous films. We analyzed the variation of the QDs luminescence response under the gradual exposure to vapors, since the mesostructure of the matrix determines the rate at which vapor molecules will adsorb onto the pore walls and eventually condensate, filling the void space.[2] These results open the route to apply ABX3 QDs in base materials for sensing. On the other hand, the aforementioned control of the water adsorption allowed us to obtain an intense and enduring blue emission (470-480 nm), with a photoluminescence quantum yield (PLQY) of 40% when Cs4PbBr6 was previously embedded in SiO2 porous matrix.[3] This blue emission originates from very small CsPbBr3 nanocrystals (diameter<3nm) formed by the controlled exposure of Cs4PbBr6 to humidity.
2.2-O1
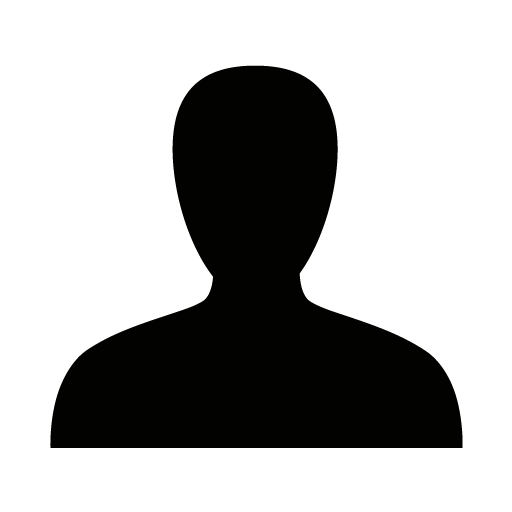
The power conversion efficiency (PCE) of silicon-based solar cells is rapidly approaching its practical limit of 29%,[1] highlighting the need for alternative absorber materials. Emerging photovoltaic materials such as hybrid halide perovskites (HHPs) have shown to be a good alternative to silicon due to their high PCE (26.7%) as well as low-cost solution-based processing methods.[2], [3]
In order to understand the impact of the precursor solution in a device processed from solution, it is necessary to gain an insight into how the precursors affect the early stage of crystallisation. To have a better understanding of the role of the solvent in HHPs precursor solutions, we investigated MAPbI3 precursor solution in γ-butyrolactone (GBL), dimethylformamide (DMF), dimethyl sulfoxide (DMSO), N-methyl-2-pyrrolidone (NMP) and mixtures thereof using small angle X-ray scattering (SAXS).
SAXS is a non-destructive technique based on the scattering length density difference between the scattering objects and the matrix. SAXS allows us to determine the average distance between the scattering objects, their size and shape as well as the interaction with each other.[4] We performed SAXS experiments at HZB synchrotron radiation source BESSY II at the four-crystal monochromator beamline[5] of the Physikalisch-Technische Bundesanstalt using the ASAXS endstation.[6]
All the SAXS patterns obtained from the measurements show a clear maximum in the scattered intensity at q-values between 2.7 and 3.8 nm-1 (values for GBL and DMSO respectively), a peak in the SAXS pattern is an indication of the presence of agglomerates in solution. The average distance between the scattering objects (dexp) varies depending on the solvent used, showing that the solvent must be part of the scattering objects. In a previous study[7] we showed that the scattering objects in solution have a core-shell configuration, with a solvent shell surrounding a [PbI6] core, which can be arranged as a single octahedron or as corner-sharing octahedra. In this study, we show that solvents with high donor number (DN), such as NMP or DMSO, present a lower dexp compared to DMF and GBL. This indicates that solvents with high DN favour the single octahedron arrangement in the core. The results obtained from the analysed SAXS data (using SASfit[8]) indicate that solvents with high DN favour monodisperse solutions, i.e. all the scattering objects have the same size. DMF and GBL favour polydispersity, which follows a lognormal size distribution. This is in agreement with the proposed core-shell model since having only a single octahedron in the core can explain the monodispersity in high DN solvents.
We will discuss how the nature of the solvent influences the arrangement of the precursors in the precursor solution since it has the potential to impact the crystallisation process of the HP and, therefore, the performance of a device produced from solution processing.
2.3-I1
Metal halide perovskites are at the forefront of next-generation optoelectronics due to their exceptional optoelectronic properties and ease of processing. However, challenges in scalability, stability, and process reproducibility remain critical barriers to their commercial adoption. In this work, we present the baseline we established for slot-die coated perovskite solar cells in the HySPRINT lab of HZB for small area test cells reaching up to 22% PCE collaboratively. We recently established a digital workflow collecting most relevant data regarding the fabrication of solar cells as well as their performance in the research data management platform NOMAD. We demonstrate how digital tools can greatly enhance intra-lab collaboration and will showcase how these kind of platforms can also be used for research data sharing by the wider research community.
We demonstrate the integration of in-situ process monitoring techniques, such as photoluminescence, during slot-die coating. These tools provide immediate feedback on critical parameters, including film thickness, drying kinetics, and phase evolution, enabling precise control over film quality and reproducibility. Data is complemented by in-situ process monitoring experiments using GIWAXS at the BESSY synchrotron.
Finally, we present an example of the fabrication of perovskite solar cell mini-modules using scalable methods, achieving efficiencies exceeding 20% with enhanced stability under operational conditions. The insights gained from these studies pave the way for the large-scale manufacturing of stable and efficient perovskite devices, addressing key technological challenges and supporting the field’s transition from laboratory to market.
2.3-O1
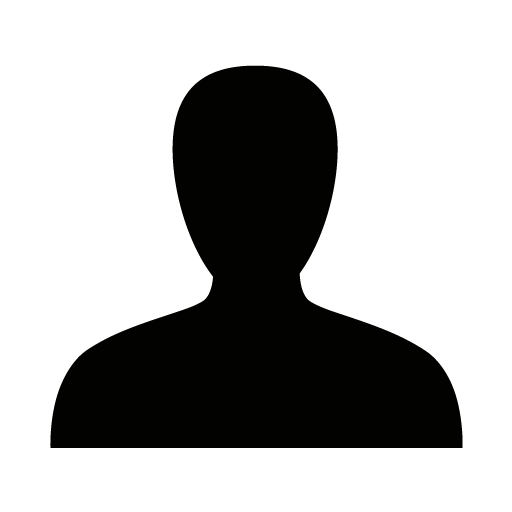
Electrochromic materials are garnering attention for applications in smart windows, displays, and augmented reality, enabling energy-efficient solutions by modulating light and heat transmission.[1]-[6] Hybrid organic-inorganic halide perovskites, renowned for their exceptional optoelectronic properties, have recently been explored for their electrochromic behavior. This study investigates perovskite precursor solutions as electrochromic materials, offering a promising alternative to conventional approaches that integrate perovskite solar cells with separate electrochromic layers. The perovskite precursor solution exhibits a voltage-dependent color change from yellow (0 V) to reddish-brown (2.5 V). The device achieves a 22% transmittance drop at 500 nm, with an average visible transmittance variation of ~30%, ideal for smart windows and display technologies. Raman and UV-Visible spectroscopy provides an insight into the mechanism and modifying A-site cations, B-site cations, or X-site anions in the perovskite structure further tunes the electrochromic properties. Demonstrations include a 4 cm² electrochromic window and a 24 cm² display incorporating a perovskite gel mixture, with response and recovery times of 7.65 s and 28.25 s, respectively. The system shows stable cyclic performance, a coloration efficiency of 1.084 cm²/C, and significant potential for energy-efficient applications. This study highlights the novel electrochromic behavior of perovskite solutions, eliminating the need for integrating solar cells with external electrochromic materials. The findings advance the development of scalable, sustainable devices for smart windows and displays, paving the way for innovative, energy-efficient technologies.
2.3-O2
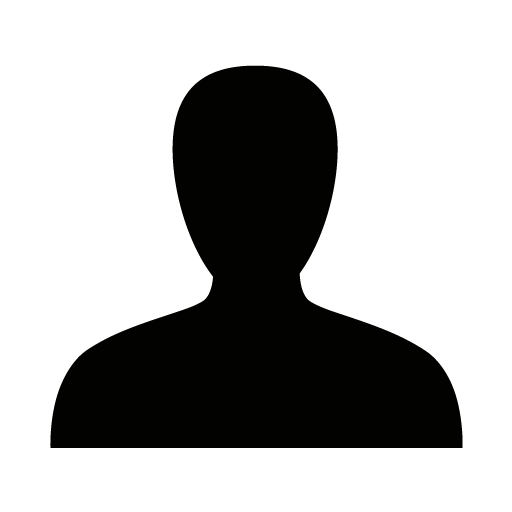
Over the past decades, three-dimensional hybrid perovskites (HPs) have gained prominence in optoelectronics, extending beyond traditional photovoltaic applications. Their versatile potential as light-emitting diodes, photocatalysts, and photodetectors positions them as key candidates for efficient, low-cost, and flexible photonic devices. Among HPs, methylammonium lead iodide (MAPI) has continued to attract interest due to its broad application potential, where defect engineering plays a crucial role in tuning its intrinsic properties. Notably, the synthesis method significantly influences the defect landscape and, consequently, the performance of MAPI.
In this work, we present a solvent-free mechanosynthesis approach to produce MAPI [1] in large quantities for electromagnetic wave absorption (EMWA) studies—a very seldom investigated application for HPs [2]-[4]. Our results reveal that 4 h-ball-milled MAPI (MAPI4h) powders with a powder size lower than 20 µm exhibits a significant improvement of dielectric loss at 11.4 GHz within the X-band frequency range (8–12 GHz) compared to powders ball-milled for only 30 minutes (MAPI30). The enhanced performance of MAPI4h is attributed to improved dipole polarization relaxation and reduced particle sizes.
Structural characterizations of MAPI4h confirm the preservation of the expected I4/mcm crystalline structure of MAPI with no apparent bulk defects but revealed smaller grain and crystallite sizes and increased strains in comparison with MAPI30. A fractal microstructure has been evidenced with aggregated grains constituted of nanograins and these aggregates form agglomerate of aggregates with different sizes. In addition, an orientation of nanograins inside grains of MAPI30 is evidenced when in MAPI4h, this oriented nanograins aggregation could be extended between the adjacent grains due to prolonged milling and localized temperature increases. Detailed defect analysis through static and time-resolved photoluminescence, Urbach energy calculations, X-ray photoelectron spectroscopy (XPS), high resolution transmission electron microscopy-energy-dispersive X-ray spectroscopy (HRTEM-EDXS), and positron annihilation lifetime spectroscopy (PALS) highlights significant surface modifications with a raise of surface vacancy-type (MA+ and I- vacancies) defect level in MAPI4h powders (<20µm). PALS, in particular, identifies the presence of specific open defects in MAPI that differ from those earlier observed in MAPI layers or single crystals prepared by solution or dry processes. A density peak in the defect distribution is located at a mean depth of about 55.9 nm from the surface of the first layer of monocrystalline grains. Its existence suggests that the defect population results from competitive reaction of generation and recombination where the grain surface plays a role of sink. The density peak tends to increase with longer grinding time.
These surface vacancies in MAPI4h behave as dielectric polarization centres by stabilizing methylammonium dipoles, which results in enhanced dipole polarization relaxations. The heterogeneous interface between the bulk MAPI and the modified surface could largely enhanced the interfacial polarization of EMWA material, improving dielectric loss [5]. Additionally, the improved dispersion of sub-20 µm powders within polymeric matrices and high specific surface area exhibiting more surface defects enable stronger particle-wave interactions during EMWA testing. These synergistic effects, driven by surface defects and structural optimization, resulted in promising EMWA properties.
This work emphasizes the potential of mechanosynthesis to tailor defects in hybrid perovskites, providing a pathway to explore defect-driven properties and expand their applications beyond optoelectronics.
1.1-I1
InCAEM will develop a singular infrastructure open to all the scientific community for research in advanced energy materials in order to address the scientific challenges of the European Green Deal. The project started in October 2022 and will have the infrastructure ready by end of 2025. It includes new equipment, infrastructure and staff.
New instruments to be installed in the ALBA premises are a high resolution (scanning) transmission electron microscope for in situ studies in gas and liquid environments and a scanning probe microscopies platform including tip-enhanced Raman spectroscopy capabilities and flexible sample environments. Advanced data infrastructures will be installed both at ALBA and at PIC-IFAE for in situ and ex situ data processing, respectively. In addition, we will carry out the adaptation of existing beamlines to perform correlative experiments, and develop methods and pipelines for multi-modal approaches.
Compatible operando sample environments for complementary characterization tools together with advanced data analysis will provide excellent opportunities for true multi-modal and multi-length scale characterization of functional materials aimed at developing new sustainable materials to be used in batteries, electric vehicles or solar cells, among others. While (S)TEM and scanning probe microscopies offer unsurpassed spatial resolution and a variety of contrast mechanisms, X-ray-based techniques provide highly specific chemical, structural, electronic and magnetic information with high efficiency so that larger fields of view, thicker samples and faster processes can be studied.
The possibility to perform experiments in situ during relevant processes such as reactions, sample growth, thermal treatment or electrochemical cycles, and the correlation of results from different instruments with identical experimental conditions will permit linking the structural, compositional and morphological aspects of the same (nano)structures and of their surroundings for a comprehensive understanding of their structure-function relationship.
We will present the project and the opportunities it will bring for the user community with the help of selected application examples.
1.1-I2
Carlo Marini studied at University of Roma “Sapienza” (Italy) where he defended the PhD in Physics in 2010. In the same year he joined the ESRF synchrotron of Grenoble (France) first as post-doc and then as junior scientist (2013) at the BM23 and ID24 beamlines. In February 2014 he joined ALBA synchrotron as second scientist at CLAESS beamline. Since 2023 he is beamline responsible of NOTOS. His scientific activity has been initially focused on the characterization of 3d metal functional materials and then it opened to the study of catalysis and in particular bimetallic nanoparticles, with a special interest to the interplay between structural and electronic properties shown by these systems. During his research, he developed a multi techniques approach which includes X ray absorption, X ray emission, X ray diffraction, Raman, Infrared, High Pressure and theoretical simulations. In 17 years of research, he produced more than 140 publications in international journals reaching a final H-index 30.
Battery technology has become increasingly important in recent years due to the growing demand for portable electronic devices and electric vehicles, and to improve the performance and lifespan of batteries it is essential to understand the complex processes that occur during their operation. NOTOS is a beamline from ALBA Synchrotron Light Source devoted to X-ray Absorption Spectroscopy (XAS), X-Ray Diffraction (XRD) which has been designed to study the electronic structure and the short- and long-range order in a wide range of scientific disciplines: chemistry, catalysis/electrocatalysis, energy science, nanomaterials, condensed matter, and environmental science. The available energy range is now 4.5-30 keV and the photon source is a bending magnet, but it will be extended up to 35 keV thanks to a partial refurbishment of the optics and the change of the bending magnet with a SuperBend source in the framework of the ALBA-II upgrade. In addition to the capability to perform XAS and XRD investigations separately, the beamline allows quasi-simultaneous XAS-XRD experiments. Examples of XAS and XRD measurements on commercial electrodes (phospho-olivine LiFePO4 and NMC111) are presented showing the performances of the beamline in operando. Finally, as real research case, we discuss the structural stability of Bimetallic Cu-doped Gold Nanoclusters in electrochemical CO2 reduction.
1.1-O1
The characterization of the electronic structure of nanomaterials, ideally in operando in an electrolyte, is essential for electrochemical applications such as in energy storage and conversion. To this aim, soft X-ray spectroscopies are particularly relevant as they enable the selective characterization of either the electrolyte or the material of interest thanks to their element specificity.1 Furthermore, the high chemical sensitivity of X-ray absorption spectroscopy coupled to the high spatial resolution (<30 nm) offered by soft X-ray spectromicroscopy enables the chemical imaging of nanomaterials, which can provide precious information about local inhomogeneities at the nanoscale.2 In this talk, I will introduce synchrotron-based in situ soft X-ray spectromicroscopy techniques and explain how they can be applied to investigate carbon nanomaterials. A special emphasis will be provided on in situ cells allowing characterization under controlled atmosphere or in liquid in the soft X-ray range. These techniques will be illustrated by our recent work on the characterization of two class of nanomaterials: (i) nanoporous carbon materials in which the addition of nitrogen can modify the interaction with water molecules,3 and (ii) 2D titanium carbides MXenes, for which the chemical bonding between the Ti and C atoms remains poorly investigated. The role of hydration will be particularly discussed along future perspectives of X-ray spectromicroscopy.
1.1-O2
Jordi Fraxedas (Tarragona,1962), PhD in Physics (1990) from the University of Stuttgart (Germany), is a CSIC Scientific Researcher at the Catalan Institute of Nanoscience and Nanotechnology (ICN2) in Barcelona (Catalonia). His thesis work was performed at the Max Planck Institut für Festkörperforschung (Stuttgart) and at BESSY (Berlin, Germany), under the supervision of Prof. M. Cardona, and worked at ESRF (Grenoble, France), CERN (Geneva, Switzerland), Materials Science Institute of Barcelona (ICMAB) and at CNRS (Angers, France). His research activity is focused on interfacial phenomena and surface science of inorganic and molecular materials using synchrotron radiation-based spectroscopies and scanning probe microscopies. He has co-authored 155 peer-reviewed scientific articles (3900 citations, H=32) and published the books Molecular Organic Materials: From Molecules to Crystalline Solids (Cambridge University Press, 2006), Water at Interfaces: A Molecular Approach (Taylor&Francis/CRC, 2014) and Molecular Materials: Preparation, Characterization and Applications (Taylor&Francis/CRC 2017). SCOPUS ID 7003376529, ORCID: 0000-0002-2821-4831.
We have developed new environmental liquid cells for Hard X-ray Photoelectron Spectroscopy (HAXPES) experiments at the HAXPES endstation of the GALAXIES beamline at the SOLEIL synchrotron radiation facility using on-purpose designed thin (15-25 nm) low-stress silicon nitride membranes. The membranes were fabricated at IMB-CNM-CSIC from 100 mm silicon wafers using standard lithography techniques and in order to facilitate fast positioning of the X-ray beam on the membrane, platinum alignment marks have been added to the chips hosting the membranes. We have selected rectangular membranes in order to achieve an optimal balance between producing large enough membranes ensuring mechanical stability as well as conforming to the elliptical shape of the synchrotron radiation beam, about 30 µm (V) × 100 µm (H), since in this configuration the strain depends mainly on the short dimension of the rectangle. Optimizing such dimensions, we have fabricated membranes with dimensions of about 20-90 um (V) x 530-600 um (H). The geometry of the HAXPES endstation, with horizontal polarization parallel to the analyzer axis and the intrinsic 54.7 degrees angle arising from the chemical etching of silicon for the fabrication of the membranes, imposes incidence angles of about 45 degrees.
Two types of liquid cells have been built: (i) a static one mounted on an Omicron-type sample holder with the liquid confined in the cell container, and (ii) a circulating liquid cell, inspired on an existing electrochemical cell installed at the LUCIA beamline [1]. The cells have been successfully tested in first exploratory experiments with aqueous solutions of salts (Na+, Cs2+, Cl-), with dispersions of gold nanoparticles and in electrochemical experiments using a coin-cell configuration [2]. The membranes are mechanically robust and withstand the 1 bar pressure difference between the liquid inside the cell and vacuum and the intense synchrotron radiation beam during data acquisition if correctly handled. The lifetime of the membranes is beyond the time scale of the performed experiments in our tests (more than 6 hours). Our results open the door to regular HAXPES studies of liquids under circulation and potentially to other techniques using synchrotron radiation such as e. g., X-ray absorption and transmission.
1.2-I1
In-situ techniques are key to elucidate the structure of the electrochemical interface, the active sites, and the reaction mechanisms of electrocatalytic reactions for sustainable energy conversion and storage. This talk will focus on the combination of electrochemical methods and different in-situ and operando surface-sensitive techniques including vibrational spectroscopy and synchrotron-based characterisation to understand and tune the structure-activity and structure-selectivity relationships for different electrocatalytic reactions of interest to produce renewable fuels and chemicals. These reactions include oxygen electrocatalysis for green hydrogen production and utilisation and the electrosynthesis of green fuels and value-added chemicals using electrochemical carbon dioxide, nitrate, and methane conversion.
First, I will present our work toward understanding and tuning the structure-activity relations on well-defined Pt-based surfaces for oxygen electrocatalysis by combining electrochemical methods, ex-situ surface science characterisation and in-situ grazing incidence X-ray diffraction [1,2]. Then, I will show our model studies on well-defined Cu-based electrocatalysts to assess the interfacial properties of the electrochemical CO2 and CO reduction reactions [3]. We have investigated new methods to evaluate and tailor the facet distribution on Cu-based catalysts [4]. Finally, I will discuss some approaches toward the in-situ investigations of the reaction mechanisms for the electrochemical nitrate reduction to produce ammonia and the co-reduction of CO2 and nitrates to produce sustainable fertilisers such as urea.
1.2-I2
Rosa Arrigo (WoS Researcher ID L-6676-2016) is lecturer in Inorganic Chemistry at the University of Salford in Manchester (UK) and honorary research scientist at the UK’ s synchrotron facility Diamond Light Source. Her research interests are focused on the design of innovative processes and nanostructured systems for decarbonization technologies in green chemistry and energy storage and conversion. Her research strategy consists of establishing molecular level structure-function relationships through the controlled synthesis of tailored materials, testing and thorough structural characterisation, including but not limited to the extensive use of innovative in situ synchrotron-based techniques such as X-ray photoelectron spectroscopy and X-ray absorption fine structure spectroscopy. Current projects focus the conversion of carbon dioxide and H2 production. Recently, she is investigating the host/guest chemistry in metal-organic frameworks for the delivery of Aspergillus derived drugs and in CO2 capture.
Selected Publications of Relevance to Catalysis Science.
Dynamics at Polarized Carbon Dioxide–Iron Oxyhydroxide Interfaces Unveil the Origin of Multicarbon Product Formation, R. Arrigo, R. Blume, V. Streibel, C. Genovese, A. Roldan, M. E. Schuster, C. Ampelli, S. Perathoner, J. J. Velasco Vélez, M. Hävecker, A. Knop-Gericke, R. Schlögl, G. Centi , ACS Catal. 2022, 12, 1, 411–430
Elucidating the mechanism of the CO2 methanation reaction over Ni/hydrotalcite-derived catalysts via surface sensitive in situ XPS and NEXAFS, G. Giorgianni, C. Mebrahtu, M. E. Schuster, A. I. Large, G. Held, P. Ferrer, F. Venturini, D. Grinter, R. Palkovits, S. Perathoner, G. Centi, S. Abate, R. Arrigo, Phys. Chem. Chem. Phys. 2020, DOI: 10.1039/D0CP00622J.
Operando X-ray absorption fine structure study of the electrocatalytic reduction of carbon dioxide over Ferrihydrite on nitrogen-doped carbon, C. Genovese, M. E. Schuster, E. K. Gibson, D. Gianolio, V. Posligua, R. Grau-Crespo, G. Cibin, P. P. Wells, D. Garai, V. Solokha, S. Krick Calderon, J. Velasco Velez, C. Ampelli, S. Perathoner, G. Held, G. Centi, R. Arrigo, Nat. Comms. 9, 2018, 935. doi:10.1038/s41467-018-03138-7.
In situ observation of reactive oxygen species forming on oxygen-evolving iridium surfaces, V. Pfeifer, T. E. Jones, J. J. Velasco Vélez, R. Arrigo, S. Piccinin, M. Hävecker, A. Knop-Gericke, R. Schlögl, Chem. Sci. 8, 2017, 2143-2149. DOI: 10.1039/C6SC04622C.
Recent Press Releases
“Take a Tour of the Diamond Light Source” in Chemistry world,
“Carbon Dioxide Conversion to Hydrocarbon: Thinking Big to See Small Things”, Nature Blog and "Beyond the Paper".
Copper-based nanostructures are active catalysts for the electrochemical reduction of CO₂ (CO₂RR) to energy-rich products, with morphology often correlated to the catalytic performance. This study investigates the synthesis, structural evolution, and catalytic behaviour of Cu₂O nanoparticles (NPs), integrating morphological control with electronic structure analysis to reveal the true role of morphology in CO₂RR. At the university of Salford, we have developed a surfactant-free synthesis method, which produces Cu₂O NPs with tuneable shapes, including sharp-edged nanocubes (NCs) and novel popcorn-like morphologies (nano-popcorns, NPCs), under varying pH and atmospheric conditions. In-situ techniques, such as X-ray absorption fine structure (XAFS) and UV-Vis spectroscopy, capture the dynamic interplay of dissolution and condensation equilibria, shedding light on the evolving chemical speciation of Cu during nanoparticle formation.
In-situ electrochemical liquid scanning transmission electron microscopy (EC-LSTEM) at Freiburg University, in collaboration with the group of Anna Fischer, has revealed distinct dynamic behaviours of the particles under CO₂RR, depending on their starting morphology. Herein, I will establish parallels between the chemistry involved during synthesis as a function of macroscopic parameters, as revealed by in-situ and ex-situ XAFS and TEM analysis, and the behaviour of the particles under CO₂RR. By bridging these observations, I aim to explain how the particle morphology evolution during CO₂RR is linked to the electronic structure of the electrocatalysts, and how these changes explain catalytic selectivity and stability.
1.2-I3
Molecular electrochemistry has been complemented for several decades by spectroscopic measurements performed under in situ conditions, a method known as spectroelectrochemistry. Several spectroscopic techniques have been used in this configuration to provide structural insight in the understanding of molecular reactions mechanisms. In this talk, we will present how X-ray absorption spectroscopy, a synchrotron-based technique, can be instrumental in determining the local and electronic structure of electrochemically generated molecular species.
We will focus on the particular case of iron porphyrins, which are very efficient catalysts for the electrochemical reduction of CO2 into CO.1 We will first present a spectroelectrochemical cell specifically designed for the study of homogeneous molecular species generated electrochemically, together with a dedicated chamber for X-ray spectroscopic experiments. We will then present and analyze the X-ray absorption spectra collected on the starting Fe(III) species and its counterparts reduced by one, two or three electrons. Comparing the data collected under argon or CO2 will provide clues on the interactions between this substrate and the metal center, as well as on the CO2 reduction mechanism.2 Finally, we will discuss the possibility to perform similar experiments with time-resolution on electrochemically generated molecular species.
1.2-O1
In the last decades, copper has attracted considerable attention over other pure metal catalysts for its exceptional performance in the electrocatalytic reduction of CO2 into valuable hydrocarbons and alcohols [1]. However, the selectivity of this reaction remains a key challenge for industrial applications. It is well-established that the selectivity is influenced by the oxidation state of the catalytic material [2], highlighting the importance of understanding and controlling the dynamics of electronic properties at the solid/liquid interface during CO2 reduction reaction (CO2RR). In-situ / operando spectroscopic techniques, such as X-ray absorption (XAS), are essential as they can provide important information about the chemical state of the elements of interest, though it is technically challenging in the soft X-ray regime.
To overcome these limitations, a strategy increasingly adopted to study solid/liquid interfaces under ultra-high vacuum conditions involves using electrochemical cells equipped with X-ray transparent Si3N4 membranes. These membranes allow the separation of the liquid phase from the vacuum while enabling X-ray spectroscopy. Such cells facilitate the investigation of changes in the oxidation state of catalytic material during electrochemical reaction by measuring XAS spectra at the core level edges, typically using total fluoresce yield (TFY) mode.
This strategy has been successfully implemented at BACH beamline, in collaboration with the technical service group of IOM-CNR [3]. The microfluidic electrochemical cell (ME-cell) features inlet and outlet channels, which allow for the renewal of the electrolyte, and a three-electrode system, comprising an Ag/AgCl leakless as reference electrode (RE), a Pt wire as counter electrode (CE) and a working electrode (WE) made of an Au-coated Si3N4 membrane onto which the catalytic material is deposited.
Stability studies of electrodeposited copper nanoparticles (CuNPs) were carried out in alkaline media, where dissolution and re-deposition phenomena were observed. Our results revealed that electrodeposited CuNPs on graphene substrates present higher stability after re-deposition, showing to be a more promising catalyst for CO2RR. Additionally, a significant effect on the chemical state of pristine CuNPs was identified after incorporating the well-known Nafion polymer as a binder. In-situ XAS measurements demonstrated that Nafion induces a persistent copper (II) oxidation state under near-catalytic conditions during CO2RR, which disappears after the first cycle of activation, suggesting a dynamic transformation of the catalytic surface. Furthermore, in collaboration with the University of Trieste, we observed that the Nafion deposition methodology (e.g., spin coating vs. drop-casting) impacts the product selectivity during the reduction mechanism of CO2.
Our results emphasize the importance of in situ XAS measurements to understand the stability of copper-based catalysts in alkaline media. Moreover our results highlight the critical role of polymer incorporation in tailoring the performance of Cu-based catalysts for CO2 reduction reaction.
1.3-I2
Peter Strasser is the chaired professor of �Electrochemistry for energy conversion and storage� at the Chemical Engineering Division of the Department of Chemistry at the Technical University of Berlin. Prior to his appointment, he was Professor at the Department of Chemical and Biomolecular Engineering at the University of Houston. Before moving to Houston, Prof. Strasser served as Senior Member of staff at Symyx Technologies, Inc., Santa Clara, USA. In 1999, Prof. Strasser earned his doctoral degree in Physical Chemistry and Electrochemistry from the �Fritz-Haber-Institute� of the Max-Planck-Society, Berlin, Germany, under the direction of the 2007 Chemistry Nobel Laureate, Professor Gerhard Ertl. In the same year, he was awarded the �Otto-Hahn Research Medal� by the Max-Planck Society. In 1996, Dr. Strasser was visiting scientist with Sony Central Research, Yokohama, Japan. He studied chemistry at Stanford University, the University of Tuebingen, and the University of Pisa, Italy. Professor Strasser is interested in the fundamental Materials Science and Catalysis of electrified liquid solid interfaces, in particular for renewable energy conversion, energy storage, production of fuels and chemicals.
The electrolytic splitting of water into its elements is Reaction No.1 in modern electrochemistry. Discovered by van Troostwijk, Nicolson, Carlisle, and Ritter, water splitting and its half-cell constituents - hydrogen evolution (HER) and oxygen evolution (OER) – later became the most widely explored electrochemical model reactions in ground-breaking works by Bockris, Parsons, Conway, and Gerischer. Over the past 2 decades, advanced characterization methods coupled to high performance computations have offered new molecular insights into the reactive interface of catalyst and electrolyte. Today, water splitting using renewable power is an emerging industrial process technology to generate “green” hydrogen, a versatile energy vector for the decarbonization of power generation, heat, mobility, and industry.
In this presentation, I will share some of our recent work on the design and ex/in-situ characterization of electrocatalytic materials, interfaces and mechanisms for the electrochemical oxygen evolution reaction (OER). Catalytic materials such as Iridium oxide and Nickel oxides along with similarities and differences in their mechanisms in acid and alkaline environments will be discussed.
1.3-I1
Transport electrification has resulted in an expansion of the Li-ion battery application from Wh to kWh storage. This has brought up additional requirements to improve performance (e.g. power, cycle life) enhance of sustainability and decrease of cost. Blending different active materials at the same cell electrode, an empirical approach commonly used for primary cells, has been readily applied to commercial EV Li-ion batteries mostly on the positive side. The global aim is to promote positive synergetic effects between the different electrode components, which have unfortunately received limited attention at the fundamental research level.
Materials with fast reaction kinetics, such as LiMn2O4 (LMO) can sustain significantly higher effective rates than the nominal rate applied to the electrode. This has motivated the widespread commercial use of NMC:LMO blends as LiNixMnyCozO2 (x+y+z=1, NMC) despite having slow kinetics, exhibits high capacity (especially for large amounts of Ni) and the addition of LMO lowers overall costs while enhancing power performance. Olivine LiMPO4 (M=Fe, Mn) based blends have a lower presence in commercial cells to date but they have also deserved attention at the laboratory scale as they can as well exhibit fast kinetics and are based on low cost abundant transition metals.
Results will be presented related to different blends, consisting of combinations of LMO, NMC, LiFePO4 (LFP) and LiFe0.35Mn0.65PO4 (LFMP). Electrochemical experiments coupled to time-resolved synchrotron operando X-ray diffraction experiments enable to capture charge transfer events between blend components which are dependent on both voltage profile and kinetics of individual blend components. The results demonstrate the significant impact of the voltage profiles of individual materials on the current distribution, with the effective C-rate of each component varying throughout the state of charge (SoC).
Finally, systematic operando synchrotron X-ray diffraction and absorption experiments will be presented for NMC and LFP with diverse experimental conditions (cell type, radiation energy etc.) to illustrate the existence of beam-induced effects which may bias interpretation of results. These range from total reaction inhibition to partial hindrance and negligible deviations from the expected mechanism, and have been consistently observed across various experimental conditions, photon energies, photon fluxes and exposure times. The total radiation dose per cycle seems to be a useful predictor of the magnitude of beam-induced electrochemical delay and could serve as a guideline to define reliable measurement protocols.
1.3-O1
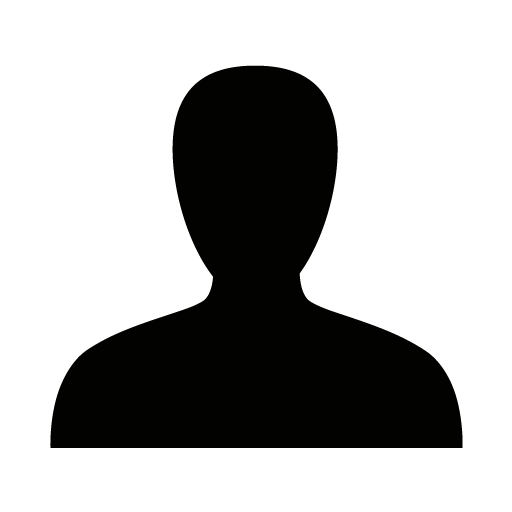
Thanks to the operating high voltages and delivered capacities, Li-rich transition metal (TM) oxide cathodes are among the most promising materials for next-generation lithium-ion-batteries, where Co-free Li-rich cathodes join reduced costs with competitive performances. However, their cycle-life remains limited, and the individual role of TMs is still not fully understood. The investigation of the TM chemical species evolution along the first charge for the Li1.2Mn0.56Ni0.16Co0.08O2 and Li1.2Mn0.6Ni0.2O2 systems have been accessed by means of operando multi-edge XAS. The charge compensation mechanism has been studied and the effect induced by removing Co has been revealed. Statistical data analysis methods reveal that the absence of Co results to accelerate and complete the Ni oxidation along the first charge and to inhibit the undesired spinel formation and oxygen release at the end of the first voltage plateau. The reasons of the oxygen release in the Co-containing electrode and its relation to structural modifications will be presented.
1.3-O2
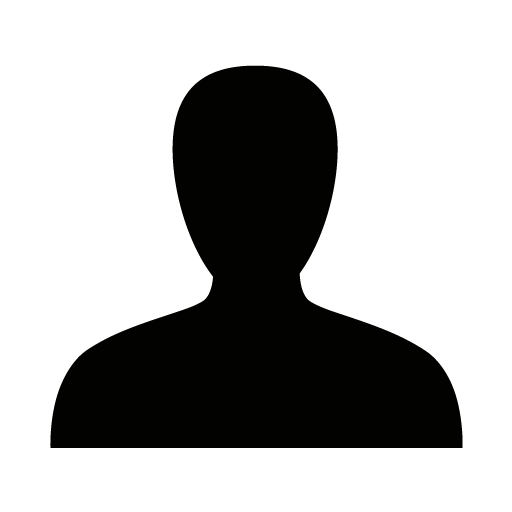
Aqueous Zn-MnO2 batteries with mildly acidic electrolytes are a promising alternative to Li-ion batteries for large-scale energy storage due to their low cost and high safety and still remarkable energy density. These advantages arise from the high capacity of the Zn metal anode and its potential allowing plating in aqueous electrolytes. Zn is in addition widespread, cost-effective and easily recyclable. Among possible cathode materials, MnO2 stands out due to its abundance, environmental advantages, and long-standing use, in Zn/MnO2 alkaline batteries. The demonstration of rechargeability in mildly acidic electrolytes has expanded its potential for energy storage.
Despite their promise, the mechanism of mildly acidic Zn/ MnO2 batteries is complex and remains debated. Early studies ruled out Zn²⁺ intercalation, instead suggesting proton intercalation or a dissolution-precipitation process involving MnO2 dissolving into Mn²⁺ and the simultaneous formation of Zinc Hydroxide Sulfate (ZHS, ZnSO4[Zn(OH2)]3·xH2O) during discharge. This dissolution-precipitation mechanism, capable of a two-electron transfer, offers a higher theoretical capacity compared to intercalation. However, practical capacities are often closer to those of a one-electron process. Furthermore, during charging, the process is not simply reversed; the electrochemical profile reveals distinct stages, including at least two plateaus and a pseudocapacitive region, indicating a more intricate mechanism. Similar multistage profiles are observed in subsequent discharges.
Clarifying the overall reaction mechanism and the factors limiting practical capacity is challenging due to the poorly crystalline nature of many involved Mn compounds, often hygroscopic, which limits structural information from diffraction and ex-situ studies.
X-ray absorption (XAS), which is capable of detecting bulk speciation of most elements regardless of crystalline state even when embedded in heterogeneous matrix is therefore a very powerful technique for such investigation, and we applied it in several different modalities. We studied the mechanism by operando XAS at the Mn and Zn K-edges to follow speciation simultaneously and quantitatively in the cathode and in the electrolyte, essentially confirming the nowadays mostly accepted dissolution/deposition mechanism, however showing some deviations in the ZHS formation rate, and quantifying parasitic H2 evolution reaction. In addition, the significant evolution of the absorption fine structure region (EXAFS) of the Mn K-edge suggested existence of Mn(III) intermediate. Further evidence of Mn(III) was provided by soft X-ray microscopy, providing access to the Mn-L edge with higher sensitivity to the oxidation state. Operando conditions were in this case consistent with more detailed ex-situ data. Finally, magnetic moment derived from Mn Kβ X-ray emission also confirm presence of Mn(III). Principal component analysis suggests that actually several kinds of reduced Mn species do exist, which can be associated with MnO2 initial defects, reaction intermediates and newly formed species.
The complementarity of operando and ex-situ techniques and the beam effects present in this system will be also discussed. Overall, such pieces of information provide a more complete overview of the reaction complexity. Nonetheless, understanding these processes is key to optimizing Zn-MnO2 batteries for large-scale, sustainable energy storage applications.
1.3-O3
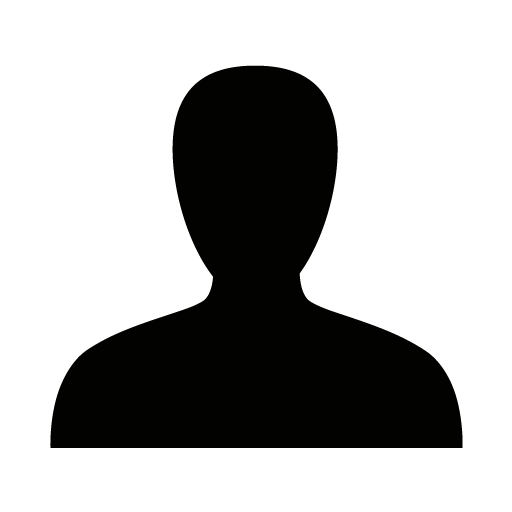
Redox Flow Batteries (RFBs) are among the most promising and innovative solutions to face the intermittency issues come from the renewable energy. Specifically, their peculiar design physically separates the power-generating stack from the energy capacity stored in the electrolyte. This feature allows for flexibility, modularity, and easy upgrades according with the necessity/application. Indeed, the RFBs can be designed from kW to MW with sufficient extended duration (> 10 hours), assessing superior safety in comparison with Li-ion batteries.
Nowadays, Vanadium Redox Flow Batteries (VRFB) dominates the market, thought their widespread application is hindered for economic, low energy/power density values and sustainability drawbacks. In this fashion, an emerging strategy from scientific community is the replacement of the vanadium by water-soluble molecules. Herein, polyoxometalates (POMs) offer exceptional advantages, enabling unusual capability to accept/donor a large number of electrons under reversible and stable manner. Additionally, their solubility in water is remarkable and the synthesis process is quite straightforward and suitable for large production.
Herein, the POM based on K6[Fe4(H2O)2(PW9O34)2]·20H2O (hereafter Fe4(PW9)2) has been demonstrated for the first time in RFB as redox active specie for the negative half-cell. The synthesis of Fe4(PW9)2 has been carried out following the steps published previously [1]. After that, the POM was stabilized in a buffer electrolyte at pH 5, using 1 M concentration of (lithium acetate and acetic acid) as supporting electrolyte. This POM is able to transfer up to 10 e-, operating at low potentials (ca. to -1 V vs Ag/AgCl) with excellent reversibility. Particularly, the Fe3+/Fe2+ redox processes appeared between 0 to 0.2V vs Ag/AgCl, involving 4 electrons; and the W5+/W6+ redox processes started below -0.5 V vs Ag/AgCl, comprising two plateaus of 3 electrons each one, the second one limited by competing H2 evolution, and possibly involving structural rearrangements affecting the corresponding oxidation potential. The long-term stability of the Fe4(PW9)2 operating in the protentional range between -0.5 to -1 vs. Ag/AgCl has been demonstrated, showing the feasibility of the Fe4(PW9)2 electrolyte operates up to 44 h achieving excellent reversibility. Finally, in operando measurements using X-ray absorption/fluorescence radiation with the individual monitoring of the Fe K-edge and W L-edge energies confirm the dynamic structure, reversibility and oxidation states at several current densities applied.
1.2-I1
Ifan is a Professor in Electrochemistry at the Department of Materials at Imperial College: he leads the Interfacial Electrochemistry Group there and is also Atoms to Devices Research Area Lead at the Henry Royce Institute.
Ifan joined Imperial College in July 2017. Prior to Imperial, he was at the Department of Physics at the Technical University of Denmark (DTU); he was first employed as a postdoctoral researcher, then as assistant professor and finally as associate professor and leader of the Electrocatalysis Group there. In 2015, Massachusetts Institute of Technology (MIT) appointed Ifan as the Peabody Visiting Associate Professor. He taught and conducted research at the Department of Mechanical Engineering at MIT for a whole semester.
Ifan’s research aims to enable the large-scale electrochemical conversion of renewable energy to fuels and valuable chemicals and vice versa. Such processes will be critical in order to allow the increased uptake of renewable energy. His focus is on the catalyst at the electrode, i.e. the electrocatalyst. It turns out that the electrocatalyst material defines the efficiency of several important electrochemical processes, including:(i) electrolysis for the storage of renewable electricity — which is inherently intermittent — in the form of fuels, such as hydrogen or alcohols.(ii) fuel cells as a potentially zero emission source of power for automotive vehicles. (iii) the green synthesis of valuable chemicals, such as ammonia and H2O2. (iv) batteries, which tend to degrade by gas evolution at the electrode-electrolyte interface. Hence the reactions that need to be accelerated in electrolysers and fuel cells — such as CO2, CO, O2 and H2 evolution — are precisely those that need to be inhibited in batteries.
Ifan has discovered or co-discovered several new catalysts for the oxygen reduction reaction, which exhibited significant improvements in performance over the prior state-of-the-art. In particular, his research on hydrogen peroxide production led to the establishment of the spinout company, HP Now.
Ifan is the recipient of RSC's Geoffrey Barker Medal (2024), the RSC's John Jeyes Award (2021). He also currently holds an European Resarch Council Consolidator Grant (2021-2025). Since 2022, he has been a Clarivate Highly Cited Researcher.
Ammonia is not only an essential component in fertilisers for agriculture but is also emerging as a promising carbon-free fuel and energy carrier for the future. However, its production remains heavily reliant on the Haber-Bosch process, which is environmentally damaging and only works well in large scale centralsied facilities. Despite extensive research, a viable alternative process has yet to be realised. An electrochemical approach, operating under ambient conditions, holds great promise by enabling decentralised, on-demand ammonia production.
To date, among solid electrodes, only lithium- and calcium-based systems in organic electrolytes have been unequivocally demonstrated to reduce nitrogen to ammonia. However, the current performance of these systems leaves considerable room for improvement.
To address this challenge, our research employs a multidisciplinary approach combining electrochemical methods, cryo-electron microscopy, infrared spectroscopy, electrochemical mass spectrometry, time-of-flight secondary ion mass spectrometry (TOF-SIMS), X-ray photoelectron spectroscopy (XPS), and density functional theory (DFT). We aim to construct a comprehensive understanding of the reaction mechanisms and factors influencing electrochemical nitrogen reduction.
We focus on elucidating the roles of various components in the process, including the choice of cations, salts, electrolytes, and proton donors. Building on this understanding, we propose pathways to achieve higher reaction rates and improved efficiencies for nitrogen reduction, paving the way for scalable and environmentally friendly ammonia production.
1.2-I2
Ammonia is a key fertilizer ingredient and, if synthesized in a sustainable way, a carbon-free alternative to liquid fuel. An alluring prospect is the production of ammonia via the electrochemical reduction of atmospheric nitrogen and water, driven by renewable electricity. However, a catalyst that facilitates the formation of ammonia while simultaneously hindering the parasitic side reaction of hydrogen evolution in aqueous electrolytes, has yet to be identified. Moreover, the persistent disagreement between theoretical predictions and experimental results hinders the further development of the field. Herein, we scrutinize the methodology and assumptions used in the bulk of theoretical studies on nitrogen reduction, suggesting improvements where applicable.
Over the last decade we have been searching, using density functional theory (DFT) calculations, for alternative materials that can catalyze nitrogen reduction reaction (NRR) while suppressing hydrogen evolution reaction (HER). The class of materials we have investigated are transition metal ceramics of e.g. nitrides, oxides, sulfides, carbides, oxynitrides and carbonitrides. Several promising candidates are predicted within each class of materials and we have tested several of them experimentally. There, we grow the catalysts in thin-films using magnetron sputtering, which are then tested in a micro reactor for electrocatalytic performance. The electrochemical micro reactor is connected in-line with the ammonia detection unit, preventing any possible contamination which makes the results reliable and robust. Experiments are done both in N2 saturated electrolyte and in Ar saturated electrolyte and isotope labelled 15N2 is used to proof catalysis. In this presentation, we discuss both the theoretical predictions and the experimental performance of several candidates for NRR. Finally, we explore the extent to which deep neural networks can be used to aid in the search for a novel catalyst, highlighting the pitfalls that must be avoided.
1.2-O1
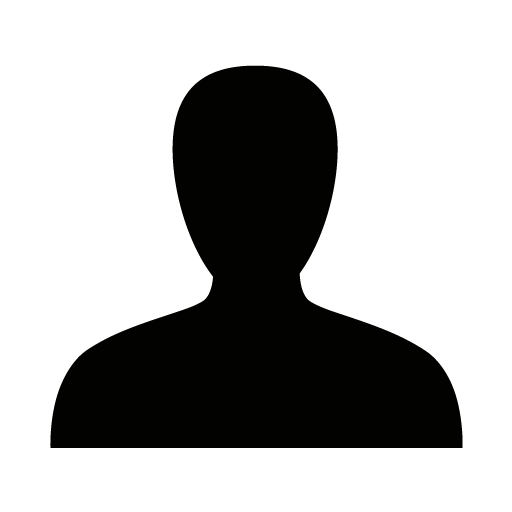
Ammonium nitrate is a key chemical feedstock which is produced by two energy intense processes, that is nitrogen reduction (Haber-Bosch Process) and catalytic ammonia oxidation (Ostwald Process). To-date, in industry, both processes use fossil fuels as energy input and raw materials. In this presentation, we propose the development of (photo-)electrochemically driven reactors capable of coupling the nitrogen-to-ammonium reduction reaction (NRR) and the nitrogen-to nitrate oxidation reaction (NOR), leading to autonomous flow photoreactors for ammonium nitrate production from nitrogen, water, sunlight, and sustainable electricity.
Specifically, we weill report on the development of noble-metal-free single atom catalysts (SACs, 1 metal reaction center) and single-site catalysts (SSCs 2 metal reaction centers) anchored to electrically conductive carbon supports.[1,2] Variation of the SAC/SSC metal sites will allow control of NRR/NOR performance, while metal stabilization in a well-defined all-oxo coordination environment will allow us to tune reactivity. Deposition of these molecular precursors on high-porosity is used to facilitate stable mechanical and electrical linkage between catalyst and electrode. In situ/operando (photo-)electrochemical studies and theoretical modelling will be reported to gain insights into the system performance, selectivity and stability. This will provide insights from the atomic to the reactor-level on the catalytic performance, its limitations, and enable us to identify key optimization parameters.
1.2-O2
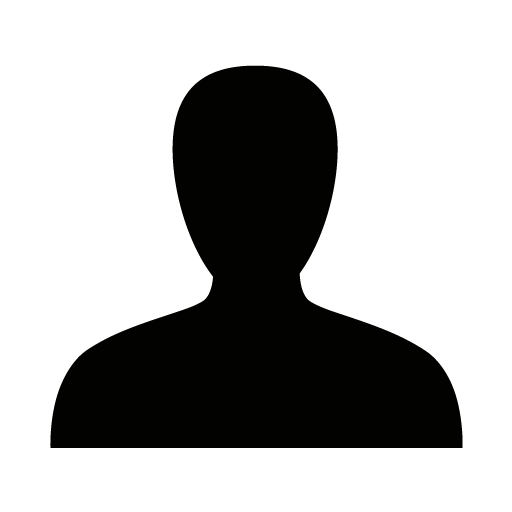
The development of the Haber-Bosch process in the early 20th century paved the way for a significant increase in ammonia production, primarily for agricultural and industrial applications. The traditional Haber-Bosch process operates under harsh conditions (high temperature and pressure) with the use of Ru- or Fe-containing catalysts [1]. Despite of the high importance of this process, its harmful impact on the environment requires to find greener alternatives.
The 2D-structures, particularly graphene-based derivatives, attract much attention in the electrochemical catalysis. It was demonstrated that nitrogen-doped graphene C2N materials exhibits remarkable catalytic activity for N2 reduction to NH3 under ambient conditions attributed to their high surface area and tunable electronic structure [2]. Additionally, the presence of the nitrogen-containing functional groups in C2N materials may serve as active sites for catalyzing nitrogen reduction and hydrogenation reactions [3]. Despite these advances, a deep theoretical understanding of the underlying catalytic mechanisms in C₂N for ammonia synthesis remains underdeveloped, highlighting the need for further exploration in this area.
This work aims to elucidate the most favorable reaction pathway for NH₃ synthesis from N2 on C₂N catalysts using quantum-chemical calculations. Both pristine and defective C₂N structures are investigated as isolated molecule model systems (in both vacuum and solvent environments) and as extended materials using periodic boundary conditions. We have analyzed associative distal and associative alternative pathways with the different adsorption sides of N2 molecule with the implementation of computational hydrogen electrode schema [4]. Our findings indicate that the distal reaction pathway is energetically favorable on defected C₂N, requiring only an applied potential of < 1 eV. We believe that our results provide valuable insights into the catalytic mechanisms of C₂N and contribute to the development of efficient and sustainable strategies for ammonia synthesis.
1.2-O3
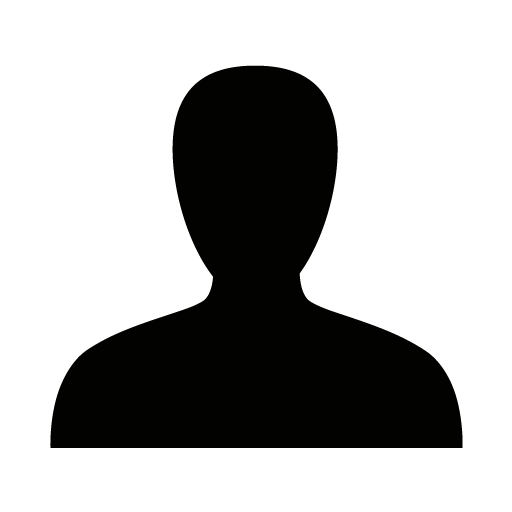
The electrochemical conversion of nitrogen (N₂) to ammonia (NH₃) represents a crucial advancement toward sustainable ammonia synthesis. With ammonia being essential for fertilizers and numerous industrial processes, the development of efficient and environmentally friendly production methods is of global importance. Traditional Haber-Bosch synthesis is energy-intensive and carbon-emitting, highlighting the need for green alternatives such as electrochemical methods.
Efficient nitroconversion requires a carefully optimized interplay between the electrocatalyst, electrode design, and electrochemical reactor configuration. This study introduces an innovative approach that integrates solid proton-conducting materials into a temperature-controlled electrochemical cell, designed to operate under moderate thermal conditions for enhanced reaction kinetics and stability. The cell features heated end plates for precise temperature regulation, supporting a variety of catalytic processes.
The modular design of the cell allows flexibility and adaptability across different configurations, enabling its use not only for electrochemical nitroconversion but also for other electrocatalysis applications involving gaseous substrates, such as CO2 electrolysis. The cell can accommodate a range of membranes and solid electrolytes with varying thicknesses and can be operated in both catalyst-coated membrane (CCM) and catalyst-coated substrate (CCS) modes.
The performance of the cell was evaluated in both N2R and CO2 reduction (CO2R) modes. This benchmarking approach was used to verify the functionality of the anode and assess overall cell performance. Ammonia formation was observed in small quantities during nitroconversion using metallic and organic catalyst systems, confirming the system’s capabilities. This work establishes a versatile platform for advancing N₂R research, with insights for optimizing cell design and operational strategies.
1.3-I1
Electrocatalytic systems are essential for various renewable energy conversion and storage technologies, serving as a cornerstone for a sustainable future. Unlocking their full potential requires advancements in catalytic materials to enhance efficiency, stability, and cost-effectiveness. Achieving these goals demands an atomic-level understanding of electrocatalytic systems, particularly the complex interface between the electrocatalyst and electrolyte, which involves numerous interacting components and processes.
The properties of this interface can vary significantly depending on factors such as the solvent and electrode potential, and these variations can directly influence electrocatalytic behavior. Theoretical and computational methods play a pivotal role in unraveling these complexities, as they provide atomic-level insights into interface chemistry under realistic reaction conditions. However, further development of these methods is crucial to fully address the challenges.
Constant potentialm grand canonical ensemble (GCE) DFT calculations [1] offer a powerful framework for modeling electrochemical interfaces and reactions at the atomic level while maintaining fixed electrode potentials. In my presentation, I will discuss recent advancements in GCE-DFT [2], which extend its applicability to systems beyond the capabilities of standard approaches.
As an example, I will present our work on the electrocatalytic nitrogen reduction reaction (NRR) to ammonia using a graphene-based single-atom catalyst. This includes an exploration of the potential-dependent reaction thermodynamics and kinetics, as well as the critical role of explicit water molecules in the calculations [3]. The competition between the hydrogen evolution reaction (HER) and NRR is analyzed in detail, with a focus on the effects of co-adsorbates and innocent ligands.
Finally, I will outline the advantages and limitations of this method, comparing it with standard DFT calculations to highlight its potential and areas for further improvement.
1.3-O1
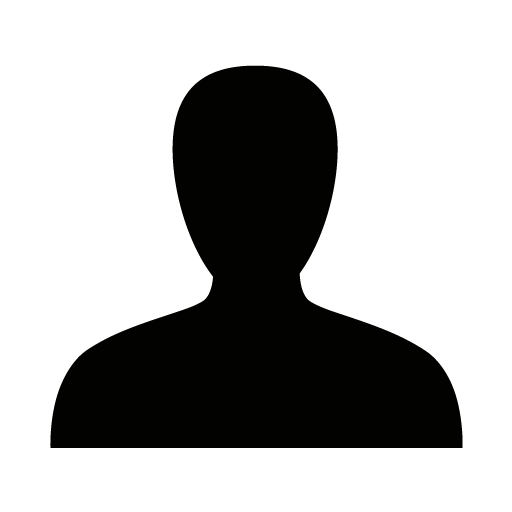
Aerogels are a unique class of 3-dimensional porous materials with outstanding properties like ultra-low density, a high surface area, and excellent thermal and acoustic insulation. Over the last century, aerogels have evolved significantly, with advancements in synthesis, aging, drying, and post-synthesis treatment techniques, enabling the production of aerogels with various properties and from various materials, including silica, carbon, metal oxides, and (bio-)polymers.
Within this work we will present the fascinating properties and possible applications of various aerogels in aviation, transportation and energy. We will then lay a focus on the design of semiconducting aerogels to be used as photocatalysts. Their open porous structure of interconnected particles provides interesting advantages compared to nanoparticle-based systems, such as advanced charge carrier transport, larger surface area and not being prone to agglomeration.
We will present a novel sol-gel based synthesis routes for (semi-)crystalline Titania aerogels and show that depending on the synthesis procedure the material parameters such as surface area, the polymorph present in the sample and the degree of crystallinity can be controlled and tuned. We thereby provide an alternative synthesis route for highly porous, highly crystalline Titania samples with specific surface areas of up to 600 m² g-1 can be obtained without calcination steps and thus obtain the open porous 3D structure.
The aerogel properties can be selectively controlled by the different synthesis parameters. Especially, the direct influence of acid concentration was investigated. Due to the dependence on the steric hindrance of the alkoxy ligands, the applied chloride ions coordinate in different ways to the used Titania precursor which changes the hydrolysis and condensation reaction kinetics. Also, the usage of different solvents leads to a ligand exchange of the Titania precursor which causes the formation of different intermediate complexes with coordinated chloride ions that act as templates for the formation of mixtures of anatase and brookite polymorphs with tunable ratios. Furthermore, the addition of denaturing agents typically used for lab-purpose ethanol significantly alters the material properties.
1.3-O2
Ammonia, a widely produced chemical, contributes to more than 1% of fossil energy consumption and plays a significant role in global greenhouse gas emissions. Therefore, exploring alternative processes to the Haber-Bosch process is of considerable scientific interest.[1] This contribution presents a two-reactor system designed for the systematic investigation of process parameters in photocatalytic ammonia synthesis, using TiO2 aerogels. TiO2 aerogels are able to store large amounts of photogenerated electrons in surface trap states upon illumination in water−methanol mixtures.[2] The designed setup splits the processes of photocharging and nitrogen reduction into two different reactors. The first reactor is dedicated to photocharging TiO2 aerogels, preparing it for N2 reduction. In this reactor, TiO2 aerogels are exposed to controlled UV-LED irradiation, focusing on maximizing the storage of photogenerated charge carriers within the TiO2 structure. The second reactor is dedicated to reducing nitrogen with the electrons in the aerogel stored by utilizing Taylor-flow conditions for enhanced mass transfer and nitrogen reduction efficiency. This reactor is engineered to maximize the contact between nitrogen and the photocharged aerogels, ensuring a more efficient and controlled reduction of nitrogen to ammonia. This study includes characterization of the photocharging reactor and an analysis of the operational parameters of both reactors. The effects of photocharging conditions and flow rates on the overall efficiency of ammonia production were investigated. Concluding this contribution, the potential of this two-reactor system in ammonia production will be discussed. The separation of photocharging and nitrogen reduction processes, combined with the use of Taylor flow, presents a significant advancement in photocatalytic ammonia synthesis. This system offers a more flexible approach to ammonia production, and fundamental understanding of both photocharging and N2 reduction process.
Quantification of ammonium at very low concentrations represents an enormous challenge and generally conventional methods are time-consuming. The indophenol method that utilizes salicylic acid, sodium hypochlorite and sodium nitroferricyanide, usually requires about 80 to 120 minutes per sample which is not only time-consuming but can be limiting for studies with a large number of samples. To minimize the effort of ammonia detection, an automated yet simple and cheap setup was established utilizing syringe pumps and a temperature-controlled reactor, finally providing the possibility for online monitoring of experiments.[3] This setup will be presented as well.
1.3-O3
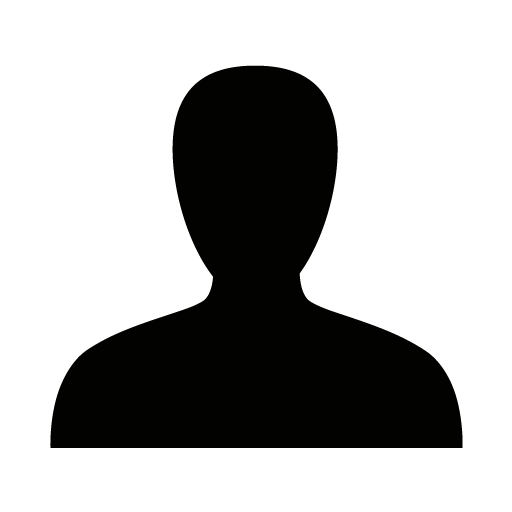
Ammonia is the most important component in fertilizers, as 80% of the worldwide production goes into fertilizers. Currently, the energetically expensive Haber–Bosch process is used to break the triple bond of N2. In the last years, the photoelectrochemical (PEC) reduction of nitrogen has gained attention, providing an alternative route to produce ammonia more affordable and sustainable. Despite beneficial properties of binary copper oxides as photocathodes for catalytic reactions such as the nitrogen reduction reaction (NRR), binary copper oxides show degradation processes, limiting their stability as electrode materials.[1,2]
To this end, the ternary copper oxides CuFeO2 and CuBi2O4 are investigated as materials for photocathodes as promising alternatives for the conversion of solar energy into chemical fuels.[3] In this work we performed Density Functional Theory calculations (DFT+U) to analyze their electronic properties, thermodynamic stability of different surfaces and adsorption energy trends for NRR intermediates. Beyond that, we investigated diverse defects in thermodynamically stable surfaces for both materials and their impact on the NRR mechanism. Moreover, the influence of an aqueous surrounding on the stability of the surfaces and reaction intermediates thereon will be investigated using the hybrid QMMM simulation approach (SAFIRES [4]) implemented in ASE and GPAW, which to this end will be extended to be compatible with periodic surface models.
1.3-O4
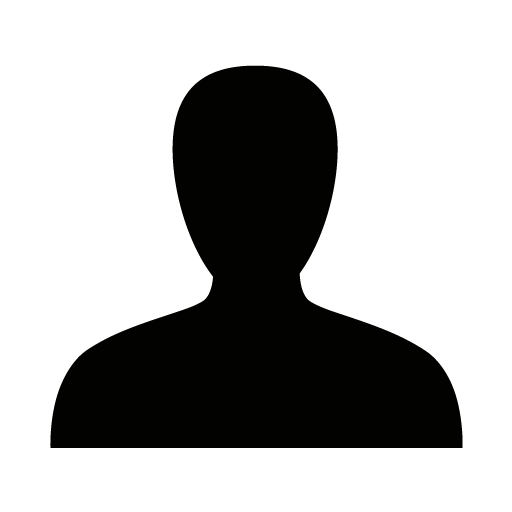
Electrochemical and photoelectrochemical reduction of nitrogenous small molecules, including N2 and NO3-, represent appealing routes for the production of NH3 under ambient conditions. However, major challenges include the activation of highly stable reactants, avoidance of spurious artifacts introduced by contamination, control of product selectivity, and suppression of the competing H2 evolution reaction (HER) in aqueous environments. In this work, we comparatively investigate the activities of Cu-based oxide semiconductors, CuO, Cu2O, and CuBi2O4, for the photo-assisted N2 and NO3 reduction reactions (N2RR and NO3RR, respectively), taking advantage of their p-type characters, moderate bandgaps, and suitable band edge positions. The ternary compound CuBi2O4 is specifically selected for its improved photoelectrochemical stability compared to the binaries. The Cu-oxide electrodes were synthesized via electrodeposition methods and the photoelectrochemical investigations were carried out in alkaline aqueous electrolytes. Notably, all photoelectrochemical investigations were performed in an optimized workstation, where background contamination (NH3, N2H4, and NOx) are carefully evaluated and high purity is ensured. Although all three Cu-based semiconductors were found to be photoactive, we observed no production of NH3 when tested for N2RR. Moreover, during photoelectrochemical NO3RR, Cu-oxide photocathodes showed major selectivity for NO2- rather than NH3. Although the selectivity for NH3 increased for Cu2O at decreased positive potentials, NO2- remained the major product of this reaction. Furthermore, photocorrosion leads to a rapid loss of photoactivity for the Cu-oxide photoelectrodes. While CuBi2O4 showed improved stability, photoelectrochemical activity was found to be reduced. However, complementary electrochemical measurements of Cu single-atom catalysts hosted on carbon (Cu@C) show appreciable activity and stability for NO3RR, yielding the two major products NH3 and NO2-, fully suppressed HER, and an NH3:NO2- ratio of 3:1 at an applied potential of -0.53 V vs. RHE. Overall, these results indicate that Cu-based oxides are poorly suited for NH3 synthesis via N2RR and NO3RR under aqueous photoelectrochemical conditions but that Cu@C may provide promise for dark electrochemical NO3RR under otherwise similar conditions.
1.3-O5
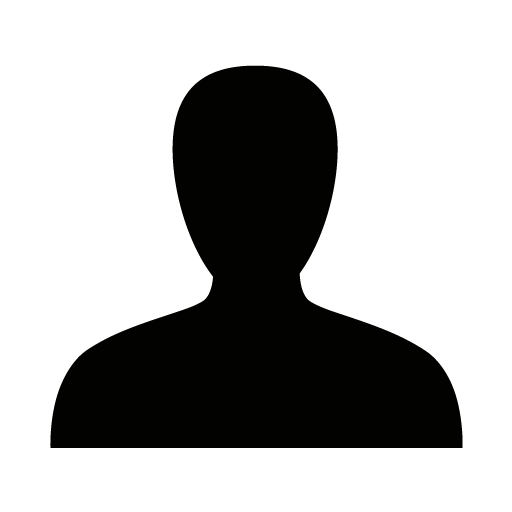
Photoelectrochemical (PEC) energy storage is a promising technology for our sustainable future. Many photoelectrodes have been developed to drive the hydrogen evolution reaction (HER) and oxygen evolution reaction (OER). Optimizing photoelectrodes that are highly active and selective to the reactions is a challenging task, as well as ensuring their stability during operation in harsh PEC environments.
In this talk, we present an illuminated scanning flow cell (iSFC) setup that enables the performance of operando PEC characterization on the activity of photoelectrodes, and simultaneously on their stability against aqueous dissolution. Selected examples of the operando setup will be presented, for instance the photo-corrosion of BiVO4 photoanodes [1], [2], and the photo-stability range of ZnFe2O4 photoanodes [3].
Then, studies on a promising photocathode material, CuBi2O4, will be presented. The photocathode has been investigated not only for HER, but also nitrogen reduction reaction (NRR) as a PEC route to fixate N2. Dissolution of Bi has been observed in an alkaline environment, and the PEC corrosion was further revealed using scanning transmission electron microscopy (STEM).
2.1-I1
The synthetic tunability of electronic structure and surface chemistry of semiconductor nanocrystals make them attractive light absorbers for light-driven chemistry. In this talk, I will describe our collaborative work on coupling nanocrystals with the MoFe protein of nitrogenase for light-driven N2 reduction to ammonia. The focus will be on our efforts to understand how nanocrystal properties determine the binding interactions with the enzyme, how electron transfer from the nanocrystals to the enzyme to drive ammonia formation, and how this platform can be used for studies of MoFe catalysis mechanisms.
We have functionalized nanocrystals to guide electrostatic attachment to the MoFe protein. Studies of experimental determinants of photochemical formation of H2 and NH3 suggest that the high excitation rates achieved with nanocrystals allow for accumulation of sufficient electrons on the enzyme to drive NH3 production. Transient absorption studies of electron transfer kinetics suggest that electron transfer efficiency is limited at single particle level by the competition between relatively slower electron transfer and relatively faster electron-hole recombination. At the ensemble level, electron transfer efficiency is also limited by the strength of the binding interactions. Microscale thermophoresis studies reveal that the nanocrystal-enzyme binding is highly sensitive to the nanocrystal dimensions. Light-driven injection of electrons into MoFe protein has enabled electron paramagnetic studies of the enzyme catalytic cycle in these systems and the key results of those studies will be highlighted.
2.1-I2
Victor Mougel completed his Bachelor's and Master's degree in Chemistry at the ENS of Lyon, and obtained his PhD at the University of Grenoble under the supervision of Prof. Marinella Mazzanti. He then joined ETH Zürich as an ETH/Marie Skłodowska-Curie Fellow before starting his independent career as a CNRS associate researcher at Collège de France in 2016. Since December 2018, he is a tenure track assistant professor at the Department of Chemistry and Applied Biosciences at ETH Zürich.
Inspired by the active sites of the nitrate and nitrite reductase enzymes, we will introduce in this talk bio‑inspired strategies to design heterogeneous catalysts for NO3RR with enhanced selectivity, activity, and stability.
Thanks to the development of an Fe-substituted molybdenum carbide MXene catalyst, Mo2CTx:Fe, we demonstrated via a combination of in situ X-ray absorption spectroscopy and density functional theory calculations that the Fe sites facilitates the formation of oxygen vacancies, which served as active sites for nitrate reduction, mimicking the mechanism of enzymatic nitrate reductase.[1] Building on this result, which highlights the importance of oxygen vacancies to provide binding sites for nitrate, we developed a reduced molybdenum oxide shell grown on dendritic nickel foam (MoOx/NiNF).[2] This catalyst displays a substantial presence of oxygen vacancies, as confirmed by X-ray photoelectron spectroscopy and in situ Raman spectroscopy, and exhibits very high NO3RR performance, achieving an FE of 99% and an ammonia yield rate of 4.29 mmol h-1 cm-2 at −1.0 V vs. RHE in neutral media, while maintaining operational stability for over 3100 hours at high current densities.
Finally, we will present novel strategies for ammonia recovery in nitrate and NOx reduction, enhancing the efficiency and practicality of NO3RR and paving the way for more scalable and applicable ammonia production methods.[3]
2.1-O1
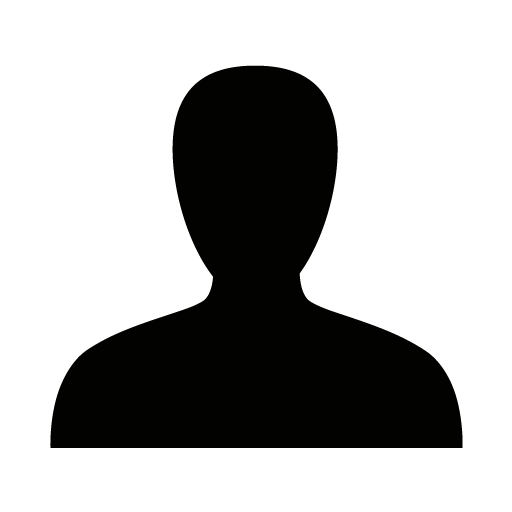
The Haber-Bosch (HB) process continues to dominate the ammonia (NH3) production market despite its significant carbon footprint. This process heavily relies on the steam reforming of methane (CH4) to generate hydrogen (H2) and requires substantial energy to allow the reaction between nitrogen (N2) and H2. Additionally, HB plants are unevenly distributed globally, meaning the transportation of NH₃ and its derivatives to end users constitutes a considerable share of the overall environmental impact. [1]
In recent years, research has increasingly explored alternative methods for NH3 production. One approach is the direct electrochemical reduction of N2 (NRR) in aqueous electrolytes under ambient conditions, utilizing renewable energy. However, this method faces challenges such as low selectivity at high current densities and limited productivity. These issues stem from the high dissociation energy of the N2 triple bond and the competing H2 evolution reaction (HER). [2] Instead, the possibility to exploit NO3− present in groundwaters and wastewaters and electrochemically convert them into NH3 results more feasible. [3] Indeed, this process has lower activation energy, which makes the reaction thermodynamically favoured compared to NRR. [4]
Our work aims to find the best operational parameters using a commercial MoS2 catalyst deposited on a gas-diffusion electrode. The tests are carried out in a flow cell of 10 cm2 geometrical area. Such a setup has the advantage of guaranteeing a better mass transport of the active species and of being scaled up. Design of experiments and surface response methodology (DoE/RSM) have been chosen to gain further insight into the influence of some operating conditions (i.e., potential, catalyst loading, and supporting salt concentration) on the Faradaic efficiency (FE) and NH3 production rate. To assess wastewater treatment, it has been decided to maintain NO3− concentration at 500 mg L−1. The model suggests the presence of two optimal conditions: one for the FE (76.9%) at -1.2 V vs Ag/AgCl potential and K2SO4 0.3 M as supporting salt and one for the NH3 production rate at -1.6 V vs Ag/AgCl and K2SO4 0.36 M (77.67 µg h-1 cm−2). Catalyst loading did not show any effect on the system responses. Setup stability has been tested for over 100 h. Additionally, other parameters such as carbon-based electrode support, flow rate, cations in the electrolyte and type of binder have been explored, at the optimal conditions obtained in the DoE, to determine if further maximization of the response is possible. Moreover, the reaction mechanism is elucidated using pulsed chronoamperometry.
2.1-O2
The growing risk of a potential energy crisis and escalating environmental challenges highlight the inminet need for research focused on alternative, sustainable energy sources capable of substituting fossil fuels. Ammonia (NH3) has been extensively produced and utilised as a fertiliser for over a century. Currently, due to its significant hydrogen content (17.6% wt), high energy density (4.32 kW h L−1 for liquid NH3), easy liquefaction for handling, storage and transportation, it is gaining momentum as a promising alternative renewable energy carrier and storage intermediate for global use in the future. [1-3] NH3 is produced via the well-established Haber-Bosch process (HBP) [1], which is well known for its high energy demands that consume 1-2% of fossil fuels worldwide, contributing to the greenhouse effect by releasing the equivalent of ca. 2% of total CO2 global emissions. [4-6] Therefore, the development of greener and more sustainable technologies to replace the century-old Haber-Bosch process is imperative. The electrochemical reduction reaction of nitrogenous spices (E-NRR) is a suitable technology widely recognised as an alternative option to the traditional HBP. E-NRR offers several key benefits:: (i) it is thermodynamically predicted to be more energy efficient than the HBP by 20%; (ii) it permits the elimination of fossil fuels as H2 source; (iii) it can be integrated with renewables energy resources (e.g. solar panel, windmill, etc.); (4) it is scalable and can lead to on-demand & on-site NH3 production. [4] Thus far, research on E-NRR has mostly focused on the electrocatalyst; however, it is well known that the electrolyte plays a crucial role, potentially having an impact of several orders of magnitude on the process outcome, particularly affecting selectivity and efficiency. In E-NRR the optimal electrolyte should enhance N2 solubility, limit the H+ to minimize the parasitic hydrogen evolution reaction (HER), maintain the catalyst stability, and thus improve the Faradic efficiency (FE). The types of proton donors, solvents and additives, such as alkali metal ions, are critical in enhancing the selectivity of the E-NRR process.
In this context, the present work aims to explore the use of glycerol-water mixture in different proportions as a solvent in the electrolyte to study the potential beneficial effect of glycerol in terms of N2 solubility increment and HER mitigation. Furthermore, it has been demonstrated that Nafion membranes, usually used as separators on E-NRR systems, represent an important source of NH3 impurities, leading to false positives or overestimation of the NH3 production. Taking that into account, a set of experiments has been performed to elucidate the membrane-ammonia interactions and quantify the impurities in our system.
2.2-O4
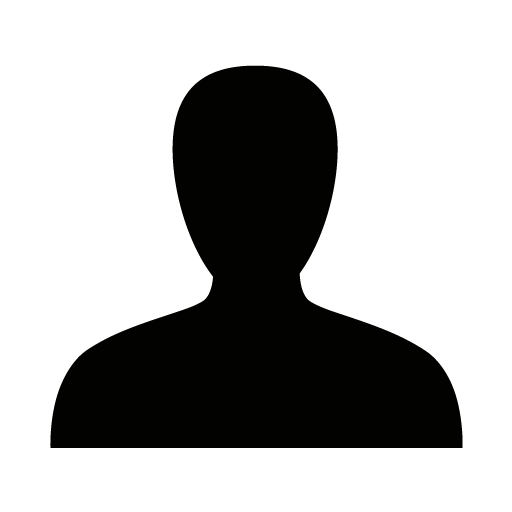
To realize the electrocatalytic nitrogen reduction reaction (NRR) numerous material systems have been experimentally investigated but their NRR activity and selectivity are far from satisfactory and thus very challenging. There is potential to approach this challenge with catalyst nanoengineering including facet-controlled growth, vacancy engineering, heteroatom doping and the fabrication of composite catalyst structures.[1] Gas phase synthesis routes like chemical vapor deposition (CVD) are promising as they allow the fabrication different materials including oxides, carbides, nitrides and sulfides on the nanoscale, while also offering the potential to tune the material properties by variation of the deposition parameters.
ZrN has been identified as a promising catalyst material for NRR,[2] as it owns electronic properties comparable to noble metals, while being cheap and abundant.
Since theoretical investigations suggest the highest selectivity and stability under typical electrochemical conditions of the (100) facets of rock salt structured ZrN,[3] we followed a metal organic chemical vapor deposition (MOCVD) approach to synthesize facet-controlled ZrN along the (100) plane. Through variation of the process parameters including the precursor choice, the substrate and the deposition temperature, crystalline ZrN (100) layers were grown on Si and glassy carbon substrates. Complementary analysis of the films by X-ray diffraction (XRD) , Rutherford backscattering spectrometry in combination with nuclear reaction analysis (RBS/NRA), X-ray photoelectron spectroscopy (XPS), scanning electron microscopy (SEM) and transmission electron microscopy (TEM) were conducted to gain more insights into the catalyst material. Interestingly, ZrN rapidly oxidizes when exposed to ambient conditions and forms amorphous ZrOxNy species on the surface. This was further substantiated by ab initio molecular dynamics simulations (aiMD), while first proof of principle electrochemical experiments hint towards a potential activity of this material.[4] While further electrochemical experiments need to be conducted in an attempt to prove the genuine NRR of this material, alternative composite catalyst materials based on the reported MoS2/Ru[5] structure are currently developed by MOCVD approach.
2.2-O5
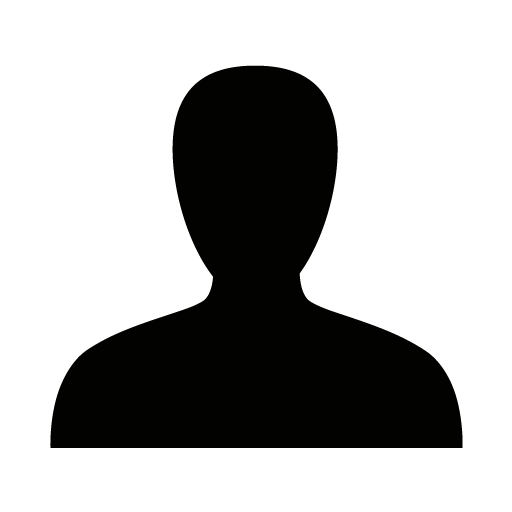
The direct electrochemical ammonia synthesis (EAS) from water and nitrogen using renewable energy shows great potential for future decentralized production of indispensable green ammonia [1]. However, identification of catalyst materials that genuinely catalyze the conversion of nitrogen to ammonia via the nitrogen reduction reaction (NRR) in aqueous electrolyte is the bottleneck for the successful development of EAS technology. To date, only Lithium-mediated NRR has been demonstrated to show sufficient production rate and faradaic efficiency for a prospective application [2], while the reaction in aqueous electrolyte, independent of the studied material class, suffers from false-positive results due to low productions rates, low Faradaic efficiencies and complex contamination issues [3].
Several catalyst systems are currently investigated for NRR in aqueous electrolytes. Transition metal nitrides (TMN) may offer an energetic advantage as electrocatalyst, because the NRR is expected to be catalyzed via the Mars-van-Krevelen mechanism (MvK) on TMNs. Here the protonation of a lattice nitrogen atom forms the first ammonia molecule. The resulting N-vacancy is filled by molecular nitrogen where one N adatom is subsequently protonated to form the second ammonia molecule. Thus, the N2 activation step occurs at the N-vacancy site and avoids the surface adsorption and dissociation of N2. Specific facets of Zr, Cr, V and Nb were described as stable, active and selective TMN catalysts for the NRR based on theoretical catalyst screening [4]. However, current literature studies state contradictory results and require the differentiation of genuine activity and non-catalytic decomposition of TMN catalysts [5], which is currently lacking.
In our work, we focus on the electrochemical evaluation of the NRR dependent on different electrode morphologies of Zr-based TMNs with trace analysis of ammonia established by ion chromatography technique [6]. Comparison of nanoparticulate ZrN catalysts [7] processed as gas diffusion electrode (GDE) to surface engineered model thin film catalyst [8] as well as insights from first principles simulation delivers a comprehensive analysis of material properties and experimental conditions for the electrosynthesis of ammonia. Detailed structural characterization after NRR experiments by x-ray photoelectron spectroscopy and dedicated chemical dissolution experiments elucidate the stability of the material as an important prerequisite of electrocatalytic NRR.
2.2-O1
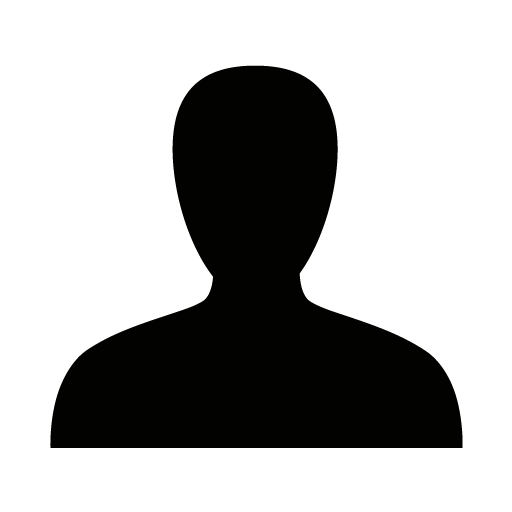
The conversion of dinitrogen into nitrous compounds like amino acids is essential for sustaining life on Earth. In addition to natural nitrogen fixation, industrial methods are indispensable to fulfil the immense global requirements, particularly for fertilizer manufacturing, mostly dependent on ammonia. Presently, ammonia synthesis relies on the energy-intensive Haber-Bosch process. In consideration of the current climate and energy challenges, investigating alternative methods for ammonia production is imperative. Our focus lies in investigating the photocatalytic reduction of nitrogen to ammonia using various bismuth oxyhalides as catalysts.
The main challenge for the photocatalytic nitrogen reduction is the low quantum yield due to the difficulty of breaking the very strong N-N triple bond. Enhancements in this process can be pursued through two approaches: identifying more suitable catalysts and refining the reaction conditions to promote the desired outcomes. Bismuth based catalysts showed the most promising results in the last years of research into photocatalytic ammonia generation. Especially bismuth oxyhalides (BiOX, X=Cl, Br, I) are an interesting material class due to their well-fitting band positions and high variability in composition, which allows to tune their reactivity. Therefore, different bismuth oxyhalides were synthesized, which we have been tested for reactivity, as well as ammonia yields. Preliminary findings have revealed that BiOBr demonstrated the highest ammonia yields. Consequently, we have shifted our focus towards the modification and enhancement of this catalyst.
Furthermore, we investigated the influence of temperature, pressure, and light intensity on the reaction. Temperature holds particular interest due to its well-documented enhancement of reaction kinetics, while pressure augments nitrogen availability, and light intensity accelerates the generation of electron-hole pairs. For the photoreactions, we employed closed vessels under a nitrogen atmosphere containing ultrapure water and the corresponding photocatalyst. To control temperature and light intensity, we positioned the samples in an oil bath and illuminated them with a high-power 365 nm LED. To assess ammonia yield, we utilized two distinct methods: an optical assay based on the common indophenol blue method, and gradient flow ion chromatography.
To define reaction parameters for the comparison, we will show results of using different temperatures, pressure, light intensity, and reaction time by using the example of titanium dioxide. Endowed with these defined parameters, we will then proceed to compare the performance of different bismuth oxyhalides alongside titanium dioxide.
2.2-O2
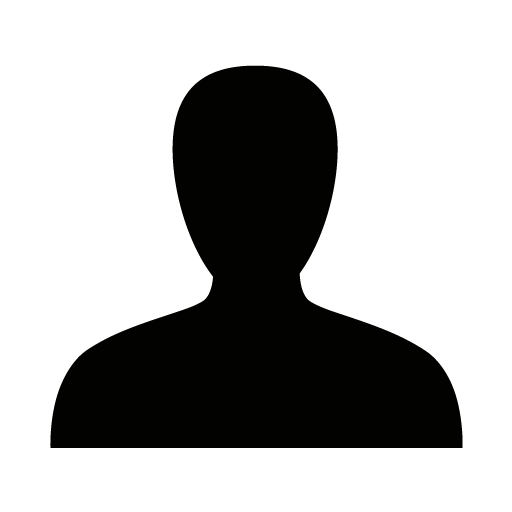
Transition metal nitrides and oxynitrides were tested as electrochemical nitrogen reduction reaction (eNRR) catalysts for ammonia synthesis under ambient conditions. There have been several promising experiments on thin film electrodes in μ-reactor (Antec Scientific) (e.g.[1]). We have synthesized these catalysts as nanoparticles and incorporated to gas diffusion electrodes (GDE) to optimize the catalytic environment. One of the optimization steps needed is to understand the effect of pressure on the system. Gas pressure has been demonstrated to significantly affect the triple phase boundary and catalytic activity in CO2 reduction and is therefore anticipated also to have an effect in NRR [2], [3], [4], [5]. To increase the pressure in the flow cell and study the effect of the gas pressure on eNRR we have constructed a system for adjustable backpressure. To this end, a pressure-relief valve is used at the gas outlet of the flowcell. We will present studies focusing on the effect of the pressure increase on the catalytic behavior in a flow electrocell with gas diffusion electrodes for eNRR.
[1] F. Hanifpour, et al., Electrochimica Acta 2022, 403, 139551
[2] K. Hara et al., Journal of the Electrochemical Society 1995, 142, L57
[3] N. Sonoyama et al., Electrochemistry Communications 1999, 1, 213
[4] E.J. Dufek et al., Journal of The Electrochemical Society 2012, 159, F514
[5] B. Sahin, Energy Technology 2022, 10, 2200972
2.2-O3
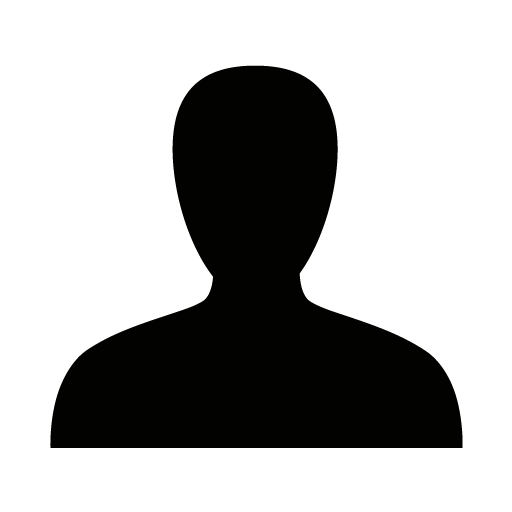
Ammonia, produced via the Haber-Bosch process for more than 150 106 t per year, is responsible of around 1.5% of the global greenhouse gas emissions. [1] It is a fundamental building-block for fertilizers and could represent a future H2 carrier, but it is still dependent from fossil fuels. To find a delocalized electrochemical process complementary to Haber-Bosch could be a key solution to move towards a renewable-driven NH3 production.
Nowadays, the lithium-mediated pathway represents the most promising solution in the N2 reduction reaction challenging field, achieving the highest Faradaic efficiency and NH3 production rate. [2], [3] Different strategies are under evaluation in literature, all exploiting the ability of this metal to bind N2 even in standard conditions. They could be divided into continuous or step-by-step strategies. In the first, Li+ ions from the aprotic electrolyte are electrodeposited on the cathode, where N2 is reduced and protonated into NH3, directly and in the same environment. [4] In the second case, the electroreduction of N2 at the cathode has been proposed, and the formation of an intermediate product containing fixated nitrogen is the key step. Only in a second separate step, this intermediate should be protonated into NH3. To achieve the first step of N2 activation, the exploitation of a Li-N2 galvanic cell, inspired by lithium-air batteries, has been proposed in literature to optimize the process efficiency and allow the direct protonation with H2O [5].
In both cases, is crucial to study the phenomena at the cathodic electrode-electrolyte interphase, as the aprotic electrolyte inevitably reacts on the active sites, forming a solid electrolyte interphase. This component of the system determines both the selectivity towards NH3 formation and the stability of the process. Due to the dynamicity of this layer, a critical eye should be adopted for a correct electrochemical characterization and NH3 quantification of the systems. Our laboratory is currently addressing these challenges within the SuN2rise project, applying also statistical methods as the design of experiment to optimize the studied factor with a restricted number of experiments.
An overview of electrochemical and engineering-related crucial aspects will be presented in this contribution. In particular, the challenges related to the NH3 quantification in highly concentrated aprotic electrolyte will be discussed, as well as the reliability of electrochemical characterization aimed to prove the N2 electrochemical reduction at the cathode. The advantages and issue related to the strategy based on the Li-N2 cell concept will be critically addressed, highlighting the precautions needed to avoid misleading result in presence of a metallic lithium anode.
2.2-O6
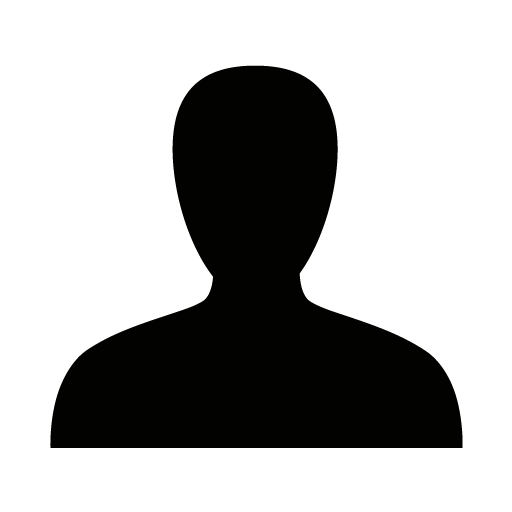
Multiphase electrochemical reactions at the gas/liquid/solid phase boundary are often limited by the low solubility of the gaseous component in the electrolyte, leading to slow reaction rates. A particularly striking example is the electrochemical synthesis of ammonia from atmospheric dinitrogen and a proton source, which occurs at 0.148 V vs. NHE and hence strongly competes with the hydrogen evolution reaction (HER) occurring at 0 V vs. NHE.[1] Since the kinetics of the nitrogen reduction reaction (NRR) are intrinsically sluggish as compared to that of the HER, high overpotentials must be applied to yield ammonia in measurable quantities so that achieving a selectivity towards NRR becomes a key challenge in the field.[2] On top, solubility of nitrogen in the commonly used aqueous electrolytes is low, while protons are ubiquitous, rendering the HER the dominating reaction in such systems. On the other hand, due to their unique structure, ionic liquids exhibit high gas solubilities, typically by an order of magnitude higher than water.[3] Hence, the utilization of composites between microporous carbons and room-temperature ionic liquids (RTILs) as electrocatalysts is an efficient way to tailor the electrode/electrolyte interface in a way that protons are prevented from accessing the electrode surface and at the same time increased amounts of nitrogen can accumulate near the catalytically active centers to enable electrochemical NRR.
This approach was performed using a literature-known iron oxide-based catalyst, deposited on activated ultramicroporous carbon via a wet impregnation technique and employing it on a gas-diffusion electrode cell. This setup ensures the direct contact between gaseous N2 molecules, solid electrocatalyst and the protons in the electrolyte, creating an ideal environment for e-NRR under ambient conditions. Ion Chromatography was used to measure the amount of produced ammonia. The resulting NH3 yield rate and Faradaic Efficiency (FE) confirm the compatibility and the synergetic effect of nanoporous AC and iron oxide towards e-NRR, paving the way for further investigation for example of the effects of pore size, hydrophilicity of the RTIL.
References:
[1] F. Qu et al., J. Mater. Chem. A, 2019, 7, 3531,
[2] J. K. Nørskov et al., ACS Catal. 2017, 7 (1), 706–709.
[3] D. R. MacFarlane et al., Energy Environ. Sci. 2017, 10 (12), 2516–2520.
2.2-O7
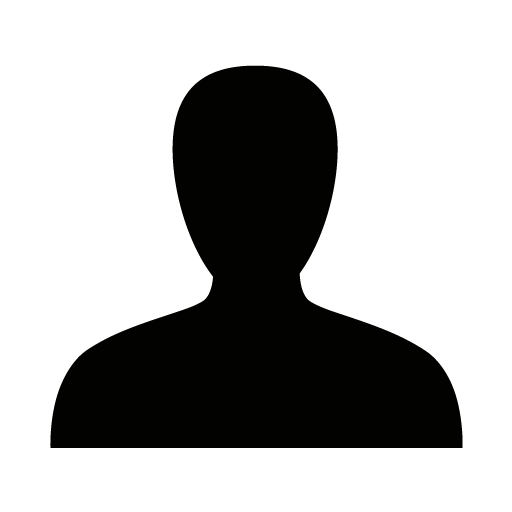
Ammonia (NH3) is an important chemical raw material, playing an integral role in the agricultural, industrial and pharmaceutical fields. Industrially, NH3 is still produced using the conventional Haber-Bosch process (developed at the start of the 20th century): N2 is reduced to NH3 by H2 using a metal-based catalyst, temperatures between 400 and 500°C and pressures between 150-250 atm. These harsh conditions make the process energetically expensive and environmentally polluting: H2 mainly comes from the reforming of hydrocarbons (usually supplied through natural gas), and it produces large emissions of CO2.[1,2]
Considering the global energy crisis and the devastating effect large emissions of greenhouse gasses have on the environment, it is of utmost importance to replace this production method with one that is greener and more sustainable, in both materials and conditions. Among many catalytic methods, photocatalytic nitrogen fixation (PNF) has been considered one of the best alternative candidates, even if it is less efficient than other photocatalytic process, because it’s powered by solar energy and both nitrogen fixation reactions (NRR and NOR) are carried out in mild conditions. [1,2]
In the search of novel and efficient photocatalysts, we investigated the potential use of heterojunctions based on graphitic carbon nitride nanosheets (gC3N4) coupled with BiOX (X=Br and I). By mapping the photocatalytic behavior of the heterojunction as a function of the weight relation between the two compounds, we found that the composite with a ratio equal to gCN(90)-BiOBr(10) produces the higher yield of NH3 (~19 μmol/g/h).
We decided to further investigate these types of heterojunctions by keeping similar ratios between the two semiconductors, but changing the components: in tandem, we mapped the behavior of the same heterojunctions, swapping gCN with rice-husk Biochar (rBC) to explore sustainable materials. In this case too, we found that the higher yield (~10 μmol/g/h) is produced by rBC(90)-BiOBr(10) and that the heterojunction maintains its structure after it has been used in the PNF reaction.
2.3-I1
The catalytic activation of small molecules/ions such as N2, CO2 or nitrate on the surface of porous electrocatalysts at their interface with electrolytes belongs to the most fundamental phenomena on the way to a more sustainable and decentralized production of commodity chemicals such as ammonia or methanol. Nanoporous carbon materials play an ever increasing role in this regards because adsorption phenomena on the carbon surface are crucial for the working principles of the respective devices. It is well known that the adsorption properties of such materials are a function of their pore architecture. Pore size, pore geometry, pore connectivity, and pore hierarchy determine important factors like mass transport and the strength of interaction with different guest species. Another (and possibly even more powerful) “regulation screw” to control the adsorption properties of nanoporous carbon materials is their atomic construction. The controlled integration of heteroatoms (most often nonmetallic group III or group V and VI elements with nitrogen being the most widely studied heteroatom) into porous sp2-based carbon networks can significantly change their physicochemical properties [1]. This includes but is not limited to their acidity/basicity, oxidation resistance, electric conductivity, and surface polarity. In order to make use of these effects it is important that the heteroatoms are significant in number, that they are uniformly distributed over the bulk of the material, and that the local atomic construction motives are as defined as possible. The synthesis of nitrogen-rich carbon materials by controlled condensation of well-defined nitrogen-rich molecular precursors is a particularly elegant way to synthesize porous carbon materials with large concentrations and precisely incorporated heteroatoms [2].
My presentation will give an overview of current attempts to develop synthetic methods for the precise tailoring of the chemical architecture and pore structure of functional nanoporous carbon materials [2],[3]. Special focus will be on the fabrication of all-carbon hybrid materials which combine a rather heteroatom-rich carbon phase and a pristine porous carbon on the nanoscale to combine, for instance, a demanded chemical property with high electrical conductivity. The structure-property-relationships of these materials in the electrochemical conversion of N2 and nitrate will also be presented [3],[4]. In addition, attempts to modify the adsorption properties of the carbon pores by immobilization of ionic liquids will be presented.
2.3-O1
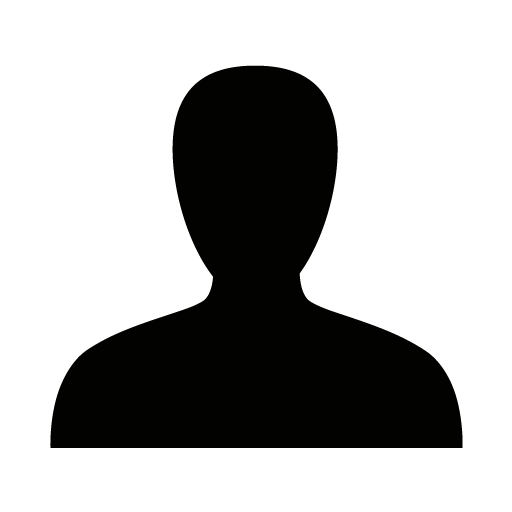
We recently summarized the strategies to improve the selectivity and faradaic efficiency of the photochemical and electrochemical reduction of nitrogen to ammonia (NRR).[1] One of the possibilities is to follow a Mars-van-Krevelen mechanism (MvK) on transition metal nitrides (TMN).[2] Moreover, TMNs possess a good chemical stability, high conductivity, flexible electronic structure and avoid the use of precious metals.[3] Skúlason and co-workers have published theoretical, density functional theory (DFT) based catalyst screening of TMNs as novel catalysts for NRR; vanadium nitride (VN) was found among the most promising active, selective, and vacancy regenerating mononitrides; for VN films especially the (100) facet is stable.[4] Also experimentally, VN has been reported to be an active electrochemical NRR catalyst.[5] However, commercial VN suffers from severe instability, both chemical and electrochemical experiments lead to significant vanadium and nitrogen leaching.[6] Thus, Pan and coworkers established an alternative NRR pathway over vanadium oxynitride; they proposed that mixed anion arrangement improve the adsorption of N2, the stability of active surface states, and their activity and selectivity over the unwanted hydrogen evolution.[7]
We have synthesized VN powders, VN nanotubes and VN sheets starting from various vanadium precursors (VOCl3, V2O5, VOx nanotubes, NH4VO3) and comparing different routes (microwave assisted hydrothermal, urea glass, carbothermal). Pure VN phases were obtained with the urea glass route operating at 800°C and the carbothermal route heated up to 1200°C. Best morphology stability of nanotubes and nanosheets was found with the urea glass route. Linear sweep analysis in N2 atmosphere indicated NRR activity somewhat preferred over hydrogen evolution, most pronounced for VN nanotubes prepared by the urea glass route.
Ongoing work includes ammonolysis to obtain stable VN morphologies and to combine VN with titanium nitride (TiN) as a stabilizing substrate. Based on DFT calculations enhanced catalytic performance due to optimized proton adsorption was proposed for VN/TiN alloys.[8] The synthesis of such alloys with TiN or other TMNs should be possible with slight modifications of our VN synthesis routes. Actual synthesis results and electrochemical NRR data employing indophenol test and 15N experiments will be presented in the talk.
2.3-O2
The interest in covalent materials has been rising recently, and structures with specifically designed properties and chemical features have widened the application spectrum of this class of materials.[1] Exemplarily, the
introduction of lightweight heteroatoms, such as nitrogen, into a sp2-bonded carbon expands the spectrum of possibilities and enables tailoring the electronic and optical properties of carbon materials. Among those, carbon nitride materials, a class of 2D covalent semiconductor with ideal formula C3N4, have recently attracted much attention especially in photocatalysis. However, up to now, their application as thin films was hindered due to the low homogeneity of the coatings available. Recently, we developed an innovative method to produce carbon nitride thin films with tunable thickness by means of chemical vapor deposition. The CVD method enables to deposit carbon nitride thin film with tunable thickness over large substrates and regardless their shape, from flat silicon wafers to fibers and even bulky irregular materials.The as prepared thin films are highly stable, homogeneous, and flat with a very high refractive index, even in the range of diamond.[2,3] The high homogeneity and conformal deposition of the carbon nitride thin films prepared enabled to use them to develop innovative batch and microfluidic photoreactors, by coating the reactors’ walls, achieving high selectivity and conversion in shorter time, and enabling overcoming problems typically associated with the use of bulk materials.[4,5] Furthermore, the utilization of carbon nitride thin films in photocatalysis enabled the development of in-operando spectroscopic techniques, such as atmospheric pressure-XPS and -XAS, combined with TOF-MS under controlled precursor vapor pressure and using a solar simulator as light stimulus. This revealed fundamental mechanistic insights and the critical role of surface interactions in key reactions, such as water splitting. The utilization of covalent semiconductor thin films, such as carbon nitrides, is still in its infancy, however, it sets the premises for significant improvements in energy conversion, energy storage, selective ion separation, and beyond.
2.3-O3
Two principal pathways exist for the photocatalytic valorization of nitrogen. The reductive route targets the formation of ammonia and the replacement of the energy-intensive Haber-Bosch process. As an alternative, nitrogen can be oxidized to nitrous oxide and nitrate, as precursors to nitric acid. Since the traditional synthesis of nitric acid via the Ostwald process is based on ammonia as feedstock, photocatalytic nitric acid formation “out of thin air” is clearly also a more sustainable solution. It has already been demonstrated that titania is able to oxidize molecular nitrogen, but the yields of nitrate were low [1]. Since titania is known to allow much higher quantum efficiencies in other challenging photosynthetic reactions [2], we attempted to improve the catalytic functionality of titania for nitrogen and oxygen activation.
In previous works we have shown for the cases of methanol and isopropanol oxidation that well-known active sites from classical catalysis are capable to carry out the same reaction light induced [3,4]. Based on this concept, we modified TiO2 with well-known nitrogen activation catalysts, based on Fe and Mo, or sites for oxygen activation, based on V and W. As a third category, based on the fundamental rule that catalysts always facilitate both forward and reverse reaction, active sites from DeNOx catalysis, based on Pd, were added to TiO2. Using an extensive set of synthesis methods, such as grafting organometallic precursors or impregnation procedures, the size range of the active sites was varied, studying both single sites and nanoparticles.
Photocatalytic activity studies revealed that Pd, in particular, can improve significantly the formation of nitrogen oxides on TiO2. Ongoing studies using in situ vibrational spectroscopy attempt to clarify the influence of the deposited active sites on the formation of reactive intermediates on the photocatalyst surface, to eventually understand the reaction pathway and to identify persistent kinetic barriers.
2.3-O4
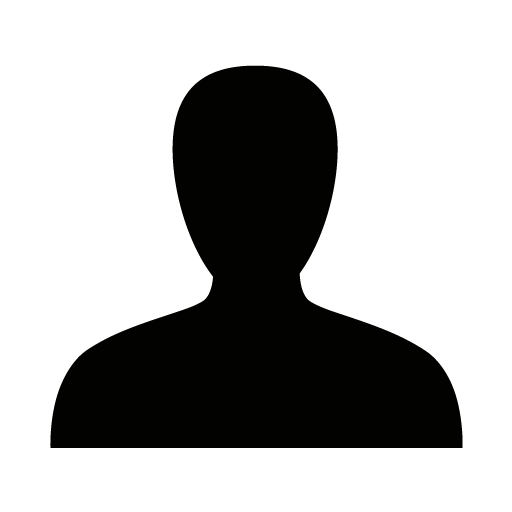
Gas-solid photocatalytic oxidation of dinitrogen to nitrogen oxides
Abdelkarim Zaim,a Jonathan Z. Bloha
a DECHEMA Research Institute, Frankfurt am Main, Germany
In recent years, there has been a notable increase in the demand for sustainable fertilizer production, driven primarily by rising energy costs and concerns about climate change. Additionally, as the global population continues to expand, there is a greater demand for agricultural commodities. While nitrogen is the most abundant element in the atmosphere, only a tiny fraction is fixed in the soil as bioavailable nitrogen, highlighting the necessity of nitrogen-based fertilizers in modern agriculture [1].
In the present era, nitric acid utilized in fertilizer manufacturing predominantly originates from the Ostwald process, wherein ammonia is oxidized. In this highly exothermic process, most of the energy contained in ammonia is converted to heat. The primary source of ammonia is derived from the Haber-Bosch process, which is responsible for approximately 1-2 percent of global CO2 emissions and is regarded as one of the largest global energy consumers [2]. A more efficient solution to this problem is the direct oxidation of nitrogen to nitric acid, as this process does not require the enormous energy input associated with the formation of NH3 (ΔRH = 383 kJ mol-1), but instead only necessitates the energy required for the formation of NO (ΔRH = 91 kJ mol-1) from the elements [3].
Photocatalysis represents a viable method for the direct generation of nitric acid from its elemental constituents. Under mild conditions, the process requires only light, water, and air. As a preliminary demonstration, the production of nitrates was shown in a gas phase reaction from water and air with the use of titanium dioxide (TiO2) as a photocatalyst. It was determined that water is an essential component of the reaction, and the primary product is NO2; this can be converted to nitric acid in water [4].
Although the mechanism of this reaction remains to be fully elucidated, we present an initial investigation of the influences of the reaction conditions on the kinetics and product composition. For example, the effects of the reactant ratio, temperature, and light intensity are considered, and all products of the reaction are analyzed both qualitatively and quantitatively. This contributes to a more comprehensive understanding of the mechanism and kinetics of the reaction.
In conclusion, a methodology for enhancing the photocatalytic oxidation of nitrogen will be presented, with the objective of achieving higher product concentrations and selectivities. This will contribute to a comprehensive understanding of the photocatalytic synthesis of nitric acid from dinitrogen.
2.3-O5
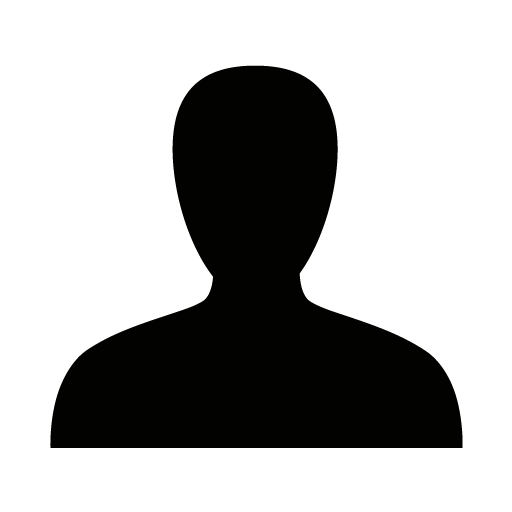
Transition metal nitrides have been discussed and tested for the purpose as catalysts for electrochemical nitrogen reduction reaction and demonstrated to be promising candidates for this reaction in aqueous electrolytes and ambient environment [1], [2]. In order to take the step from the sub-optimal environment for eNRR using thin film catalysts on solid electrodes to a more optimal environment with gas diffusion electrodes (GDE) for enhanced triple phase boundary and using nanoparticles for increased surface area aspect ratio, we have synthesized the selected transition metal nitrides using the urea gel route [3], [4], [5].
A significant amount of carbon is added to the catalysts through the catalyst synthesis method and due to sintering in N2(g) environment it may not all be removed as gaseous compounds. Therefore, the total catalyst (transition metal nitride) proportion in the powder needs to be known. We have used TGA-MS to identify the carbon and quantify the total metal proportion in the catalyst powder material. We have used XRD to analyze the crystal structure, SEM-EDS to analyse the particle morphology and C&N analysis to quantify N and C, this way allowing for a complete understanding of the powder chemical composition.