The program is in CEST time
Phases-I1
Hybrid materials are functional platforms that combine the synthetic control of molecular chemistry with the robust structural and electronic properties of crystalline inorganic solids. We utilize pressure to explore correlations between compression-induced structural changes and electronic properties in hybrid materials to direct our efforts in tuning these materials and synthesizing new variants. We examine these structure-property relationships using high-pressure X-ray diffraction, dc resistivity measurements, and absorption, vibrational, and photoluminescence spectroscopies. Two- and three-dimensional hybrid halide perovskites have emerged as versatile materials for energy storage and conversion, such as solar-cell absorbers, white-light phosphors, and battery electrodes. Through application of gigapascal-scale pressure to 2D copper chloride perovskites, we recently demonstrated the first instance of appreciable conductivity in a copper-chloride-based material. I will discuss how the pressure-driven structural evolution of copper halide perovskites results in dramatic piezochromism, increased conductivity, and the emergence of new electronic structures. In addition, I will demonstrate how we can then utilize chemical modification to bring this compression-induced conductivity within more technologically accessible pressures. I will end with a brief discussion of future pressure-related exploration within our new research group.
Phases-I2
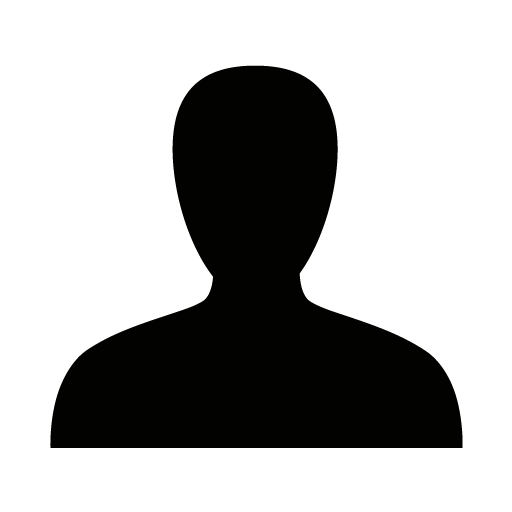
The emission of white light from a single material is atypical and is of interest for solid-state lighting applications. Broadband light emission has been observed in some layered perovskite derivatives, A2PbBr4 (A = R-NH3+), and correlates with static structural distortions corresponding to out-of-plane tilting of the lead bromide octahedra. While materials with different organic cations can yield distinct out-of-plane tilts, the underlying origin of the octahedral tilting remains poorly understood. Using high energy resolution (e.g., quasi-elastic) neutron scattering, this contribution details the rotational dynamics of the organic cations in A2PbBr4 materials where A = n-butylammonium (nBA), 1,8-diaminooctammonium (ODA), and 4-aminobutyric acid (GABA). The organic cation dynamics differentiate (nBA)2PbBr4 from (ODA)PbBr4 or (GABA)2PbBr4 in that the larger spatial extent of dynamics of nBA yields a larger effective cation radius. The larger effective volume of the nBA cation in (nBA)2PbBr4 yields a closer to ideal A-site geometry, preventing the out-of-plane tilt and broadband luminescence. In all three compounds, we observe hydrogen dynamics attributed to rotation of the ammonium headgroup and at a time scale faster than the white light photoluminescence studied by time-correlated single photon counting spectroscopy. This supports a previous assignment of the broadband emission as resulting from a single ensemble, such that the emissive excited state experiences many local structures faster than the emissive decay. The findings presented here highlight the role of the organic cation and its dynamics in hybrid organic–inorganic perovskites and white light emission.
Phases-I3
In 3D halide perovskites, the choice of the A-site cage cation is governed by the so-called Goldschmidt tolerance factor. When a large A-site cation exceeding the proper tolerance factor is incorporated, it will cause significant expansive strain in the structure and usually the corner-sharing perovskite structure is disrupted. However in 2D perovskites, the expansion of the perovskite cage can be compensated by the compressive strain induced by the spacer cations to reduce the accumulated strain energy. Expanding the cage is equivalent to exerting “negative pressure” on the perovskite structure, which cannot be achieved by applying physical pressure. We have found that large A-site cations such as formamidinium (FA), methylhydrazinium (MHy), dimethylammonium (DMA), ethylammonium (EA) and guanidinium (GA) can all fit into the cages of 2D perovskites. Increasing the size of the A-site cation can lead to Pb−I bond elongation, bandgap blueshift, lattice softening and PL quenching, providing a perfect model system to study the effect of A-site cations on the photophysical properties of perovskites.
films-I1
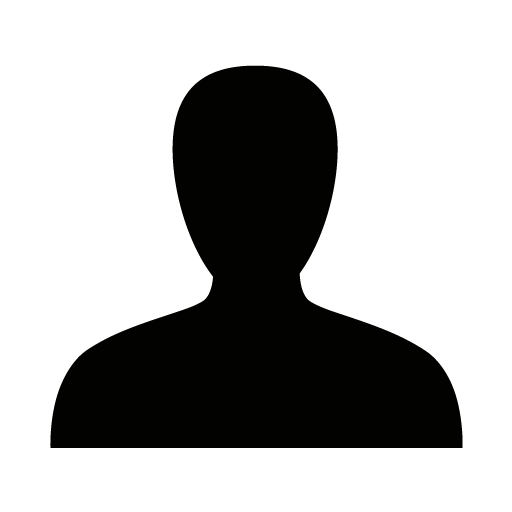
The layered nature of two-dimensional metal halide perovskites (2D HaPs) gives rise to a large exciton binding energy that often leads to efficient radiative recombination of charge carriers. 2D HaPs thus often offer bright luminescence that can be tuned over a large part of the visible and ultraviolet spectral regions - rendering them attractive candidates for applications of light emission and for studying fundamental aspects of exciton physics.
In many cases, such studies are conducted on polycrystalline thin films through techniques that provide a signal averaged over a large sample area, i.e including many different grains and grain boundaries. At the same time data analysis often relies on simplified models, such as a bulk semiconductor picture that neglects the impact of surfaces, interfaces, and the presence of impurity phases.
As a straightforward and non-invasive set of techniques, optical microscopy is both capable of offering a wide array of photophysical insights of 2D HaPs, and at the same time providing a high spatial resolution.
In this talk, I shall give examples of where simplified pictures of bulk semiconductors fail to explain the properties of 2D HaPs, and how their behaviour can be unravelled through a concerted effort of optical microscopy techniques. A combination of hyperspectral PL imaging and Raman spectro-microscopy is especially powerful. The presented findings will underline the important role of local defects, grain boundaries, impurity phases, and the general microstructure of 2D HaPs for both Ruddlesden Popper and Dion Jacobson phases, and how they need to be accounted for to understand macroscopically accessible information.
films-I2
Quasi-2D perovskites are promising candidates for stable and efficient optoelectronic devices, such as light-emitting diodes, solar cells, and photodiodes. On top of superior environmental stability compared to their 3D counterpart, they possess versatile optoelectronic properties. In fact, by varying the number of inorganic layers sandwiched between the organic spacers (n), bandgap and exciton binding energy can be tuned. During film deposition, however, a variety of structural phases with different n-values are formed, usually making a 2D-3D gradient where lower dimensional phases are located at the bottom of the film and 3D at the top.
Chasing phase purity has been a challenging task for researchers, as both kinetics and thermodynamics play an important role in determining which phases are formed. While solvent engineering has been used to tune phase purity, no reports have investigated the effect of co-solvents on the crystallization mechanism of quasi-2D perovskites and their impact on phase distribution. To fill this gap, we first investigated the kinetics of film formation of quasi-2D perovskites in presence of co-solvents [1]. By using such a solvent engineering approach and in-situ techniques, we explained the mechanism behind 2D-3D gradient formation and how to selectively tune it.
We then employed 2D-3D graded perovskites for a variety of applications. First, to investigate the dependence of halide segregation in mixed-halide perovskites on their dimensionality. By forming such a multidimensional film, we could in fact study the photostability under illumination of both 2D, quasi-2D, and 3D perovskites. Then, to enhance the performances of perovskite photodiodes, which we found to be strongly dependent on a thermal charge generation mechanism taking place at the interface between the electron-blocking and the active layer.
films-I3
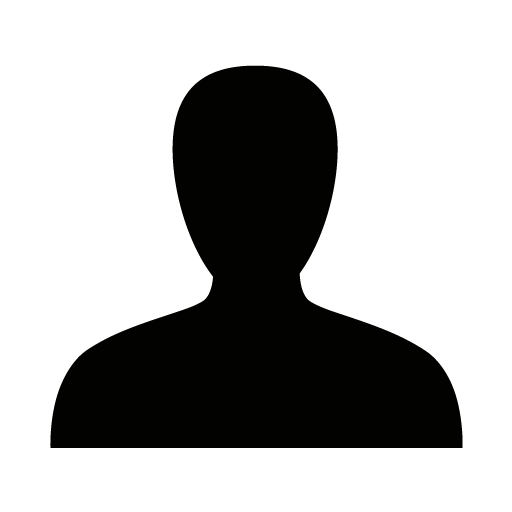
De-mixing is an important phenomenon in material science referring to the phase separation occurring in mixtures, including metallic alloys and ionic solids. Under dark at equilibrium, thermodynamic variables, such as temperature and composition, play an important role on determining the phase stability of a mixture. Interestingly, in 2015 Hoke et al. reported that reversible phase separation in a mixed-conducting mixture of metal halide perovskites, (e.g. MAPb(BrxI1-x)3) can be induced with illumination[1], opening important questions on the thermodynamics of energetically pumped system. As the light induced evolution of different phases involves significant ion transport, improved understanding of the underlying defect chemical mechanisms involved in this photo-induced phase separation (photo de-mixing) and in the re-mixing occurring under dark(dark re-mixing) is necessary for both understanding and controlling this effect. However, to date, there are few reports devoted to investigating thermodynamic parameters in these energetically pumped systems, including the evaluation of the composition for the photo de-mixed phases. This is further complicated by the light induced degradation affecting halide perovskites. The improved stability of 2D perovskites[2] makes them suitable model systems for investigating photo de-mixing. Furthermore, the suppressed ion transport in 2D halide perovskites compared with classic 3D hybrid perovskites observed experimentally raises questions on the influence of dimensionality on the defect chemistry and the phase properties of these mixtures.
Here, we consider Dion-Jacobson mixtures based on (PDMA)Pb(Br0.5I0.5)4 (PDMA: 1,4-phenylenedimethanammonium spacer) as a model system for our study. We demonstrate that 2D mixed halide perovskites undergo photo de-mixing with direct transformation from the pristine phase to the de-mixed phases. Almost complete re-mixing of these phases occurs when the sample is left in the dark, showing that the process is reversible in terms of optical and structural properties. We have further conducted temperature-dependent absorption measurements under light to extract the photo de-mixed compositions and to map the photo-miscibility-gap with three different methods.[3] Lastly, we propose a model that describes photo de-mixing in mixed lead halide perovskites where we emphasize that, in addition electronic effects, the energetics related with potential ionic defect formation should be considered for the overall energetic balance that drive photo de-mixing.[4, 5]
Films-I1
Organic-inorganic metal halide perovskite semiconductors have demonstrated real promise as a next-generation thin film photovoltaic technology based on unprecedented gains in device efficiencies and electronic tunability. However, the lack of device reliability remains a key drawback. We show that there are large, tensile stresses that form during perovskite processing, which is a direct consequence of the large mismatch in coefficients of thermal expansion (CTE) between stiff substrates (i.e., silicon and glass) and the perovskite. Perovskites already suffer from a number of instabilities—both chemically and thermomechanically—and the discovery of large tensile stress values in films is a property that, if ignored, could inhibit the long-term success of perovskite solar cells as an industrially relevant, commercialized technology. The stress is independent of any underlying charge transport layers and agnostic to fabrication methodology other than the temperature at which the perovskite is formed. The aim of this work was to understand how processing and operating conditions affect residual stress in perovskite films and to determine methods for controlling the amount of stress in the final film to engineer more reliable perovskite devices. We develop three simple approaches for reducing stress in perovskite films that involve the use of lower formation temperature, higher CTE substrates (i.e., PET and polycarbonate), and halide exchange—each of which is shown to improve stability. Our work also has important implications for device design and characterization. Specifically, reducing film stress is critical to enable reliable, module-scale perovskite devices that would otherwise be prone to delamination from the build-up of stresses.
Films-I2
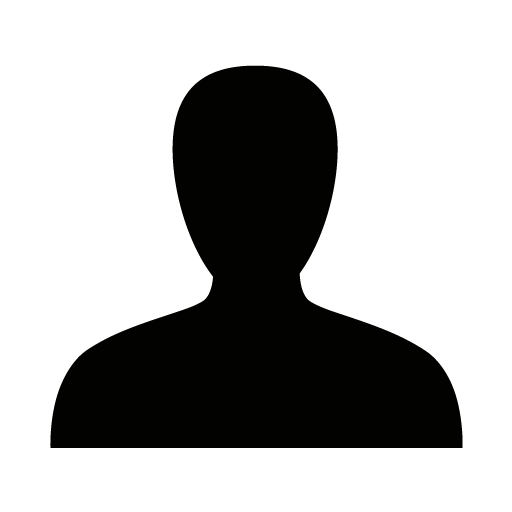
Hybrid organic-inorganic halide perovskite materials are promising for light emitting applications. We have established a general protocol to prepare ultrathin, smooth, passivated, and pinhole free films of metal halide perovskites with various compositions, by incorporating bulky organoammonium halide additives to the stoichiometric 3D perovskite precursors. In this way, we are able to preserve the benefits of a non-excitonic material but utilize the optoelectronic and mechanical benefits of bulky organic molecules. LEDs produced in this way are capable of exceeding 17% external quantum efficiency, exhibit significantly improved stability, and are capable of being as flexible as organic electronic thin films. Finally, they allow for stabilizing mixed halide (I and Br) and mixed Pb-Sn stoichiometries such that we can tune emission from the green to near infrared.