The program is in CET Time.
1A-I1
Conjugated polymers provide a unique toolbox for establishing electrical communication with biological systems. In this talk, I will show how modulating the chemistry of these materials can lead to interfaces that maximize interactions with biological systems while maintaining electronic transport properties. These materials are then used in organic electrochemical transistors (OECTs) to detect biological species in physiological media. I will introduce two types of OECT based sensors; one that detects metabolites with performance exceeding the state-of-the-art, and the other that detects coronavirus spike proteins at the physical limit. Having challenged these sensors with patient samples and cellular media, I will discuss areas where proof-of-concept platforms may fail and how to prolong the operation in biological environments. By tackling each of these problems, we improve device performance to a level that marks a considerable step toward label-free diagnostics.
1A-I2
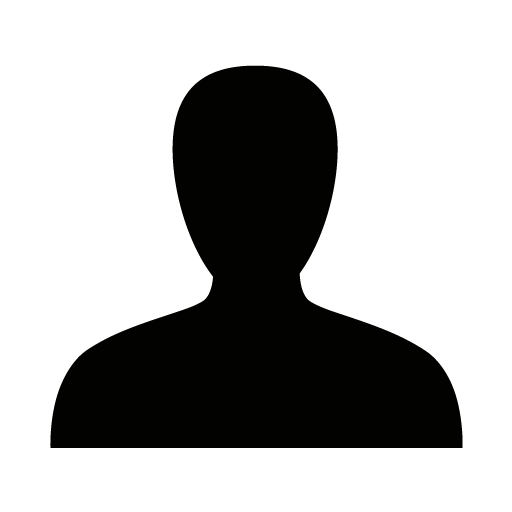
The interface between biological cells and non-biological materials has profound influences on cellular activities, chronic tissue responses, and ultimately the success of medical implants and bioelectronic devices. The optimal coupling between cells and materials is mainly based on surface interaction, electrical communication and sensing1.
In the last years, many efforts have been devoted to engineer materials to recapitulate both the environment (i.e., dimensionality, curvature, dynamicity)2 and the functionalities (i.e., long and short term synaptic plasticity)3 of the neuronal tissue to ensure a better integration of the bioelectronic platform and cells. In this scenario, resembling the operation and the composition of the neuronal membrane might be beneficial to reconstitute synaptic proteins’ arrangement (i.e. synaptic receptors) and electronic functionalities to further optimize the communication between neuronal cells and in vitro bioelectronic platforms2.
Here, we explore how organic neuromorphic devices and supported lipid bilayers (SLBs) can recapitulate short and long term plasticity in biohybrid synapses.
Through the neurotransmitters’ oxidation (i.e. catecholamines) first, we were capable of modulating the synaptic potentiation and ultimately the coupling with biological cells to form a functional synapse.
and artificial membranes we were capable of tuning the response of a neuromorphic platform to modulate its conductance over time. Then, we functionalized the organic neuromorphic transistor with a supported lipid bilayer and investigated how the PEDOT:PSS – artificial membrane interface can affect the ion flow and thus the device’s conductance over time and the short term plasticity of the biohybrid synapse.
In turn, this could represent a first step toward in vitro adaptive neurohybrid interfaces to engineering neuronal networks with biomimetic structural and functional connections at synaptic level.
References
1. Lubrano, C. et al. Towards biomimetic electronics that emulate cells. MRS Commun. 10, 398–412 (2020).
2. Mariano, A. et al. Advances in cell-conductive polymer biointerfaces and role of the plasma membrane (2021).
3. Keene, S. T. A biohybrid synapse with neurotransmitter-mediated plasticity. Nat. Mater. 19, 16 (2020).
1A-I3
Magnus Berggren received his MSc in Physics in 1991 and graduated as PhD (Thesis: Organic Light Emitting Diodes) in Applied Physics in 1996, both degrees from Linköping University. He then joined Bell Laboratories in Murray Hill, NJ in the USA, for a one-year post doc period focusing on the development of organic lasers and novel optical resonator structures.
In 1997 he teamed up with Opticom ASA, from Norway, and former colleagues of Linköping University to establish the company Thin Film Electronics AB (ThinFilm). From 1997 to 1999 he served Thin Film as its founding managing director and initiated the development of printed electronic memories based on ferroelectric polymers.
After this, he returned to Linköping University and also to a part time manager at RISE Acreo. In 1999, he initiated the research and development of paper electronics, in part supported by several paper- and packaging companies. Since 2002, he is the professor in Organic Electronics at Linköping University and the director of the Laboratory of Organic Electronics, today including close to 90 researchers.
Magnus Berggren is one of the pioneers of the Organic Bioelectronics and Electronic Plants research areas and currently he is the acting director of the Strategic Research Area (SFO) of Advanced Functional Materials (AFM) at LiU. In 2012 Magnus Berggren was elected member of the Royal Swedish Academy of Sciences and in 2014 he received the Marcus Wallenberg Price. He is also the co-founder of 7 companies: ThinFilm, Invisense, DP Patterning, Consensum Prodcution, OBOE IPR, OBOE Players and Ligna Energy.
Thiophene-based trimers is a new class of organic electronic molecular units, which enable the organization and polymerization of conjugated structures within living biological systems and inside operating electronic devices and systems. The trimers can be equipped with side groups that targets and promotes coupling to specific surfaces and (bio-)chemical cues making self-organization and -assembly of organic bioelectronics possible in a novel manner. The chemical and physical fundamentals of the trimers, the route of polymerization, and their performance while operating in neuromorphic and in vivo-manufactured bioelectronics will be reported. Specifically, neuromorphic systems based on organic electrochemical transistors including the trimers will be described along with bioelectronic systems formed inside living plants, cells and animal models. Our findings promise for radically new ways of forming bioelectronic systems in living systems, which mimicks the structures and functions of the signaling of biology.
1A-T1
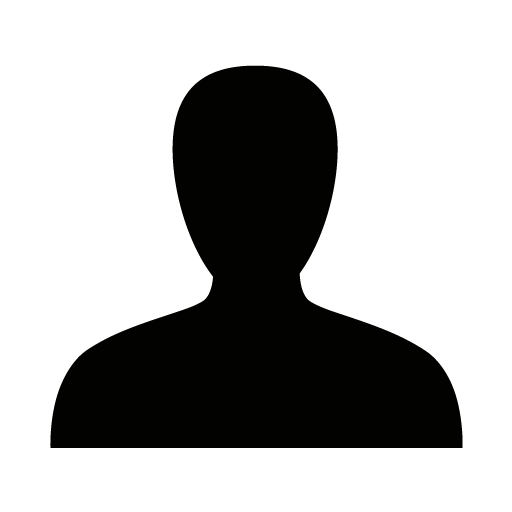
Organic electrochemical transistors have garnered strong interest due to ability to efficiently transduce biological signals into electronic ones thanks to the mixed ion and electron conduction of the semiconducting film in the channel. However, the lack of understanding of these mechanisms during the operation of OECTs hinders the use of OECT-based bioelectronic technologies in circuits. Here we investigate how polymer hydration in aqueous biological media affects mixed conduction properties of n-type (electron transporting) semiconductor transistors. We use electrochemical quartz crystal microbalance with dissipation monitoring (eQCM-D) coupled with UV-Vis and impedance spectroscopy to observe the hydration of n-type polymers with varying lengths of ethylene glycol side-chains attached to NDI-T2 backbone. By varying the hydrophilic content as well as conformation of the n-type polymer, we modulate the hydration which in turn governs the in situ (dynamic) morphology of the film and hence the electronic and ionic mobilities. Our study suggests that hydration is not de facto beneficial for the n-type OECT performance, as has recently been observed for the p-type counterparts.1
1A-T2
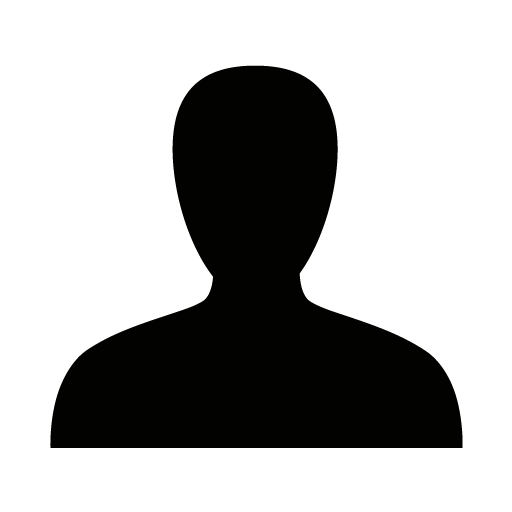
Among the existing two-dimensional materials, MXenes, i.e. transition metal carbides, nitrides and/or carbonitrides, stand out for their excellent electrochemical properties. Due to their high charge storage capacity, metal-like conductivity, biocompatibility as well as hydrophilicity, Ti3C2Tx MXene-based inks hold great potential for scalable production of skin conformable electronics via direct printing methods. Herein, we develop an aqueous MXene ink and inkjet-print MXene films on freestanding, flexible, and conducting polymer-based substrates. These skin-adherent MXene electrodes detect electrocardiography signals with high signal-to-noise ratio while exhibiting preserved electrical performance after 1000 cycles of bending with a 50 d long shelf life in ambient conditions. We show that printed MXene films can be further functionalized to perform as multifunctional biosensing units. When integrated with a sodium (Na+) ion selective membrane, MXene electrodes detect Na+ in artificial sweat with a sensitivity of 40 mV per decade. When the films are functionalized with antibodies, they generate an electrical signal in response to a pro-inflammatory cytokine protein (interferon gamma) with a sensitivity of 3.9 mV per decade. Our findings demonstrate how inkjet-printed MXene films simplify the fabrication of next-generation wearable electronic platforms that comprise multimodal sensors.
1A-T3
C. Tortiglione is researcher at CNR since 2001, heading the Nanobiomolecular group at Istituto di Scienze Applicate e Sistemi Intelligenti "E.Caianiello" (ISASI-CNR, Pozzuoli) of National Research Council since 2007. After completing her graduate research at Istituto di Genetica e Biofisica (IGB-CNR, Naples), she spent two years at University of Edinburgh, in the laboratory of Developmental Biology (Prof. M. Bownes). Back in Italy she received her PhD at University of Naples, developing new skills in Plant Genetics and Biotechnology, followed by several postdoctoral appointments. At ISASI she launched new research lines merging Biology to Nanoscience. Beside basic investigations on key pathways controlling development and cell differentiation using cell and molecular biology tools, the novelty of her research is the development and the use of nanoparticle-based methods for analysis of gene and cell function, manipulation of intracellular pathway, optical and magnetic hyperthermia, controlled drug delivery. Recently, she is exploiting the possibility to use organic semiconducting polymers to control cell function. She demonstrated the possibility to modulate animal behaviour and light sensitivity by using photovoltaic nanoparticles, and is currently using these materials for therapeutic purposes. More recently she exploited the possibility to use the biocatalytic machinery of living organisms for fabricating functional hybrid bioelectronic interfaces, fully integrated into the tissues, using semiconducting oligomers as build blocks
The unmatchable capability of living cells to fabricate complex structure starting from simple building blocks offers new paradigms to seamlessly integrate new electronic structures into the living matter, creating new hybrid devices. We exploited this potential in a simple tissue-like organism, the freshwater polyp Hydra vulgaris, and here we provide an overview of recent and on-going results obtained with this model together with our methodological approaches ranging from behavioural to optical, spectroscopical, and electrical characterization. By simple exposure to conjugated oligomers we show the capability of Hydra to fabricate new electronically conducting and electrochemically active microstructures in specific cell types [1]. In another case, fluorescent and conductive microfibers embedded into the tentacle tissues were produced starting from oligothiophene compounds, showing the feasibility to use these organisms as biofactories to fabricate and to test novel bioelectronic interface [2]. Tissues with integrated biocompatible electronics, manufactured in vivo in localized regions, may inspire new devices to manipulate biological functions by adding or augmenting conductivity in physiological or pathological contexts with spatiotemporal control, paving the way to new bioengineering concepts.
References
[1] G. Tommasini, G. Dufil, F. Fardella, X. Strakosas, E. Fergola, T. Abrahamsson, D. Bliman, R. Olsson, M. Berggren, A. Tino, E. Stavrinidou, C. Tortiglione, Seamless integration of bioelectronic interface in an animal model via in vivo polymerization of conjugated oligomers, Bioactive Materials 10 (2022) 107-116.
[2] M. Moros, F. Di Maria, P. Dardano, G. Tommasini, H. Castillo-Michel, A. Kovtun, M. Zangoli, M. Blasio, L. De Stefano, A. Tino, G. Barbarella, C. Tortiglione, In Vivo Bioengineering of Fluorescent Conductive Protein-Dye Microfibers, iScience 23(4) (2020) 101022.
1A-I4
Eleni Stavrinidou is an Associate Professor and leader of the Electronic Plants group at Linköping University. She received a PhD in Microelectronics from EMSE (France) in 2014. She then did her postdoctoral training at Linköping University (Sweden) during which she was awarded a Marie Curie fellowship. In 2017 Eleni Stavrinidou became Assistant Professor in Organic Electronics at Linköping University and established the Electronic Plants group. She received several grants including a Swedish Research Council Starting Grant and she is the Coordinator of the HyPhOE-FET-OPEN project. In 2019 she received the L’ORÉAL-UNESCO For Women in Science prize in Sweden. In 2020 she became Associate Professor and Docent in Applied Physics. The same year she was awarded the Future Research Leaders grant of the Swedish Foundation for Strategic Research. Her research interests focus on organic electronics for plant monitoring and optimization, energy applications and bio-hybrid systems.
Leveraging the biocatalytic machinery of living organisms for fabricating functional bioelectronic interfaces in-vivo not only enables seamless integration of microdevices into the tissue but also a greener solution for advanced microfabrication. Previously we have demonstrated that plants can polymerize conjugated oligomers in-vivo forming conductors within their structure. We showed that the polymerization is enzymatically catalyzed by endogenous peroxidases and we developed a series of conjugated oligomers that can be enzymatically polymerized in physiological conditions. The conjugated polymers integrate within the plant cell wall structure adding electronic functionality into the plant that is then explored for energy storage. Recently we demonstrated intact plants with electronic roots that continue to grow and develop enabling plant-biohybrid systems that maintain fully their biological processes. Furthermore, we have extended this concept into an animal model system. We demonstrated that Hydra, an invertebrate animal, can polymerize intracellularly conjugated oligomers in cells that expresses peroxidase activity. The conjugated polymer forms electronically conducting and electrochemically active domains in the µm range integrated within the hydra tissue. Our work paves the way for self-organized electronics in plant and animal tissue for modulating biological functions and in-vivo bio-fabrication of hybrid functional materials and devices.
1A-I5
Skin is the body’s largest organ, and is responsible for the transduction of a vast amount of information. This conformable, stretchable, self-healable and biodegradable material simultaneously collects signals from external stimuli that translate into information such as pressure, pain, and temperature. The development of electronic materials, inspired by the complexity of this organ is a tremendous, unrealized materials challenge. However, the advent of organic-based electronic materials may offer a potential solution to this longstanding problem. Over the past decade, we have developed materials design concepts to add skin-like functions to organic electronic materials without compromising their electronic properties. These new materials and new devices enabled arrange of new applications in medical devices, robotics and wearable electronics. In this talk, I will discuss basic material design concepts for realizing stretchable, self-healable and biodegradable conductive or semiconductive materials. I will show our methods for scalable fabrication of stretchable electronic circuit blocks. Finally, I will show a few examples of applications we are pursuing uniquely enabled by skin-like organic electronics when interfacing with biological systems, such as low-voltage electrical stimulation, high-resolution large area electrophysiology, “morphing electronics” that grows with biological system and genetically targeted chemical assembly - GTCA.
2A-I1
Darren is an Associate Professor and the Director of Research at the School of Pharmacy, University of Auckland. His main research drive is the use of materials to communicate with and influence the body through microelectrode arrays and the intelligent delivery of drugs.
The team’s research has demonstrated the ability of conducting polymer coatings to improve the performance of sensing and stimulating bio-electrodes. Darren has expertise in releasing different drugs in a controlled fashion from a range of injectable and implantable platforms. His translational and multidisciplinary research aims to address currently unmet health demands. The technologies my team design and test are used in implantable medical devices to answer fundamental research questions and to treat spinal cord injury. In the future these implants could be used for diagnostic purposes and as a platform for novel treatment strategies in tissues responsive to chemical and electrical cues.
As an academic pharmacist he work with scientists, health professionals and patients. Darren has built productive collaborations across different disciplines around the world. He is committed to achieving real world translation of research to make a difference to people’s lives.
Bioelectronic devices have found use at the interface with neural tissue to investigate and treat nervous system disorders. We have developed and characterized a very thin flexible polyimide-based bioelectronic implant that is inserted along the thoracic spinal cord in rats directly in contact with the dorsal surface of the spinal cord. There was no negative impact on hind-limb functionality nor any change in the volume or shape of the spinal cord. The bioelectronic implant was maintained in rats for a period of 3 months. We have obtained the first subdural recordings of spinal cord activity in freely moving animals. Recordings contained multiple distinct voltage waveforms spatially localized to individual electrodes. A clinically relevant spinal contusion injury can be achieved with the implant in place. This device has great potential to monitor electrical signaling in the spinal cord after an injury, and in the future, this implant will facilitate the identification of biomarkers in spinal cord injury and recovery. We are exploring further development of this implant to deliver localized treatments to the spinal cord towards regeneration of damaged tissues to recover lost function. Localized therapies are being developed in the form of pharmaceutical treatment through the local delivery of neurotrophic factors, and electroceutical treatment through precise electrical field stimulation.
2A-I2
Thomas Stieglitz was born in Goslar in 1965. He received a Diploma degree in electrical Engineering from Technische Hochschule Karlsruhe (now: KIT), Germany, in 1993, and a PhD and habilitation degree in 1998 and 2003 from the University of Saarland, Germany, respectively. In 1993, he joined the Fraunhofer Institute for Biomedical Engineering in St. Ingbert, Germany, where he established the Neural Prosthetics Group. Since 2004, he is a full professor for Biomedical Microtechnology at the Albert-Ludwig-University Freiburg, Germany, in the Department of Microsystems Engineering (IMTEK) at the Faculty of Engineering and serves as deputy spokesperson of the Cluster BrainLinks-BrainTools, board member of the Intelligent Machine Brain Interfacing Technology (IMBIT) Center and spokesperson of the profile neuroscience / neurotechnology of the university. His research interests include neural interfaces and implants, biocompatible assembling and packaging and brain machine interfaces. Dr. Stieglitz has co-authored about 170 peer reviewed journal publications, 330 conference proceedings and holds 25 patents. He is co-founder and scientific consultant of CorTec GmbH and neuroloop GmbH, two spin-off companies which focus on neural implant technology and neuromodulation, respectively. Dr. Stieglitz is Fellow of the IEEE and serves the EMBS in the neuroethics group and the technical committee of neural engineering, the German Biomedical Engineering Society (DGBMT im VDE) where he is chair of the Neural Prostheses and Intelligent Implants section, the Materials Research Society. He is also founding member of the International Functional Electrical Stimulation Society (IFESS).
Miniaturized neural implants cover the wet interface between electronic and biological circuits and systems. They need to establish stable and reliable functional interfaces to the target structure in chronic application in neuroscientific experiments but especially in clinical applications in humans. Proper selection of substrate, insulation and electrode materials is of utmost importance to bring the interface in close contact with the neural target structures, minimize foreign body reaction after implantation and maintain functionality over the complete implantation period. Our work has focused on polymer substrates with integrated thin-film metallization as core of our flexible neural interfaces approach and silicone rubber with metal sheets. Micromachining and laser structuring are the main technologies for electrode array manufacturing. Different design and development aspects from the first idea to first-in-human studies are presented and challenges in translational research are discussed. Reliability data from long-term ageing studies and chronic experiments show the applicability of thin-film implants for stimulation and recording and ceramic packages for electronics protection. Examples of sensory feedback after amputation trauma, vagal nerve stimulation to treat hypertension and chronic recordings from the brain surface display opportunities and challenges of these miniaturized implants. System assembly and interfacing microsystems to robust cables and connectors still is a major challenge in translational research and transition of research results into medical products.
2A-I3
Education
2000: Ph.D. from Johann Wolfgang Goethe-University, Frankfurt, Medical School. Supervisor: Prof. Wolf Singer, Max Planck Institute for Brain Research, Frankfurt, Germany.
1998: M.D. from Johann Wolfgang Goethe-University, Frankfurt, Medical School, Frankfurt, Germany.
Professional experience
Since 2009: Director of the Ernst Strüngmann Institute (ESI) for Neuroscience in Cooperation with Max Planck Society, Frankfurt, Germany.
Since 2008: Scientific Member of the Max Planck Society. Since 2008 Professor of Systems Neuroscience, Radboud University Nijmegen, Nijmegen, The Netherlands.
2001 - 2009: Principal Investigator, F.C. Donders Centre for Cognitive Neuroimaging, Radboud University Nijmegen, Nijmegen, The Netherlands.
1999 - 2001: Postdoctoral Research Fellow with Dr. Robert Desimone, Laboratory of Neuropsychology, National Institute of Mental Health, Bethesda, Maryland.
1998 - 1999: Postdoctoral Research Fellow with Prof. Wolf Singer, Max Planck Institute for Brain Research, Department of Neurophysiology, Frankfurt, Germany.
1998 - 1999: Residency at the Johann Wolfgang Goethe-University, Frankfurt, Medical School, Department of Psychiatry, Frankfurt, Germany.
Neuroscience in non-human primates, as well as neurotherapy in patients, can benefit tremendously from recent developments in engineering flexible interfaces to record neuronal activity. In particular thin-film electrodes offer the potential for electrophysiological recordings over very long periods of time, accessing any part and potentially covering large parts of the brain. I will present some of the work that we have performed, in collaboration with engineers, to develop and use polyimide-based electrodes for recordings from the cortical surface and the cortical neuropil. I will then try to identify those potential future developments that are most urgently needed and those that will enable the largest further steps.
2A-T1
A materials chemist (M.Sc. in “Photochemistry and Molecular Materials”), deeply interested in biological systems (Ph.D, in “Molecular and Regenerative Medicine”). From the very beginning of his research career he has been intrigued by the development of novel ways to translate text-book material properties and figures of merit into desirable real-life device performances, by governing process variables and device layout design. Since 2018 he is a post-doc at the Center of Translational Neurophysiology of Speech and Communication of the Italian Institute of Technology, his research activity is focused on organic bioelectronics, on its translational applications to biological and medical problems and on the development of organic neuromorphic devices. He co-authored 23 peer-reviewed papers in international journals of high impact, with >350 citations and h-index 10 (source: Scholar), and the “Organic Electronics” lemma of the Italian Encyclopedia “Treccani”. Since 2018, he holds the “Surface Science” course of the inter-university Professional Master's Programme on “Polymeric materials and products for the biomedical sector” of the University of Bologna and University of Modena and Reggio Emilia and he is passionately involved in diversified outreach and technology transfer activities.
Successful translation of electronic devices, operated as sensors and/or transducers, to clinical settings demands precise knowledge of their working principles as well as of the peculiar needs arising from the targeted application scenarios. This demands a tight mutual exchange between the scientific fields of device engineering and neurosciences, which metaphorically mirrors the most ambitious goal of Neuroelectronics: namely the bidirectional communication of electronic devices with the living matter, and, in particular, with the central nervous system.
The technological and scientific platform of organic electronics provides materials, tools and procedures to effectively tackle this goal, giving rise to the field of Organic Neuroelectronics.
Typically, operation of Organic Neuroelectronic devices demands driving voltages across the biotic/abiotic interface, which may result in undesired electrochemical reactions that are harmful both for the patient and for the device.
This talk reports on a novel device architecture which bypasses these drawbacks: the common-drain/grounded-source electrolyte-gated organic transistor. When implanted, this architecture allows one to maximize brain-signal amplification while applying null net bias, and consequently eliciting no parasitic currents, across the tissue. We demonstrate the viability of the proposed configuration by recording in vivo the somatosensory evoked activity from the barrel cortex of rats, achieving a superior signal-to-noise ratio which enables the detection of evoked activity at the single-trial level.
2A-T2
I'm currently PhD student in the Department of Bioelectronics (BEL) at the Centre de Microélectronique de Provence of the the Ecole Nationale Supérieure des Mines de Saint Etienne. I received a BS in applied physics a MS in Nanophysics at the University of Isfahan (Isfahan, Iran) and another MS in photonic at Univerity of Strathclyde (Glasgow, UK). During my past research, I worked on syntheses and characterization of metal nanoparticles as drug delivery systems and fluorescent biosensors.My PhD at Mines focuses on the design and microfabrication of neural electrodes for recording epileptic high frequency oscillations. I fabricate electrodes with new material for reducing the mechaanicl mistmach between neural implants and brain tisuue. I also work on local and controlled drug delivery on neural implants to reduce brain inflammortory response.
In drug-resistant partial epilepsies, resective surgery is the treatment of choice to suppress seizures, provided that the epileptogenic zone (EZ) is clearly identified and that it can be safely removed. In this context, the capacity to rely on objective biomarkers of the EZ is fundamental to define the optimal surgical approach in the specific context of each patient. One of the most important biomarkers are electrophysiological signals which represent local field potential variations generated by a network of neurons. Here our focus is a specific oscillation band knows as fast ripple (FR) with the frequency band between 250 Hz to 600 Hz. FRs are transit signals which mixed with ongoing activities and last only few tens of millisecond. There are fundings that show FRs are specific to epileptogenic zone. However, routine clinical use of FRs is a long path since we do not know which information is carried by FRs and how FRs correlates with epileptogenic seizure [1] .
The aim of this project is to optimize the recording electrodes properties to improve the detection of epileptic FRs. In first stage we benefited from the epileptic computational mode and tuned the electrode’s characteristics including size, impedance, geometry, and material. According to the simulated results, microelectrodes are fabricated. The impedance of electrodes were tuned by controlling the electrodeposition of PEDOT:PSS conductive polymer. Electrode’s recording sites are characterized in terms of morphology, impedance, electricidal conductivity, uv-visible and furrier transform infrared transmission. The double layer capacitance of the electrodes was measured experimentally and modeled with an equivalent circuit [2-3].
In the next step, microelectrodes are implanted in kainate mouse model of temporal lobe epilepsy for 28 days. The recorded signals are segmented and processed to extract the fast ripples. We found that the amplitude of the FRs signals to the background activity increases by lowering the impedance. In addition, the cut-off frequency at which electrodes the electrode transits from predominantly resistive to capacitive, highly influence the FRs resolvability.
2B-I1
Róisín M. Owens is Professor of Bioelectronics at the Dept. of Chemical Engineering and Biotechnology in the University of Cambridge and a Fellow of Newnham College. She received her BA in Natural Sciences (Mod. Biochemistry) at Trinity College Dublin, and her PhD in Biochemistry and Molecular Biology at Southampton University. She carried out two postdoc fellowships at Cornell University, on host-pathogen interactions of Mycobacterium tuberculosis in the dept. of Microbiology and Immunology with Prof. David Russell, and on rhinovirus therapeutics in the dept. of Biomedical Engineering with Prof. Moonsoo Jin. From 2009-2017 she was a group leader in the dept. of bioelectronics at Ecole des Mines de St. Etienne, on the microelectronics campus in Provence. Her current research centers on application of organic electronic materials for monitoring biological systems in vitro, with a specific interest in enhancing the biological complexity and adapting the electronics to be fit for purpose. She has received several awards including the European Research Council starting (2011), proof of concept grant (2014) and consolidator (2016) grants, a Marie Curie fellowship, and an EMBO fellowship. She currently serves as co-I and co-director for the EPSRC CDT in Sensor Technologies, renewed in 2019. She is a 2019 laureate of the Suffrage Science award. From 2014-2020, she was principle editor for biomaterials for MRS communications (Cambridge University Press), and she serves on the advisory board of Advanced BioSystems and Journal of Applied Polymer Science (Wiley). In 2020 she became Scientific Editor for Materials Horizons (RSC). She is author of 100+ publications and 2 patents and her work has been cited more than 6000 times.
In vitro models of biological systems are essential for our understanding of biological systems. In many cases where animal models have failed to translate to useful data for human diseases, physiologically relevant in vitro models can bridge the gap. Many difficulties exist in interfacing complex, 3D cell biology models with technology adapted for monitoring function. Polymeric electroactive materials and devices can bridge the gap between hard inflexible materials used for physical transducers and soft, compliant biological tissues. An additional advantage of these electronic materials is their flexibility for processing and fabrication in a wide range of formats. In this presentation, I will discuss our recent progress in pushing conducting polymer devices, to 3D, to simultaneously host and monitor complex multi-cellular models of tissues and organs. Building on our previous work that showcased a bioelectronic model of the human intestine, we are now incorporating elements of the microbiome and the immune system as well as the enteric nervous system. Coupling this model with our model of the neuro-vascular unit (including blood brain barrier) currently in progress, will bring us to our goal of a physiologically representative in vitro model of the gut-brain-microbiome axis.
2B-I2
Organic electrochemical transistors (OECTs) are electronic devices having organic mixed
ionic/electronic conductors as core components. The efficient ion-to-electron conversion of
organic materials allows for low operating voltages, high amplification, and adaptability to various
form factors. These characteristics, together with operation in physiological conditions make
OECTs promising devices for bioelectronics, including the recording of action potentials from
electrogenic cells.[1].
When interfacing with cells in vitro, the active material should satisfy several requirements
to allow both device performance and a suitable platform for cell culture. On the device side, the
conjugated polymer should provide a path for ionic and electronic conductivity as well as stability
in contact with aqueous electrolytes. Additionally, for in vitro cell culture, the polymer should be
resistant to sterilization, provide long-term stability in conditions needed for cell survival (i.e., 37
ºC in cell culture media), and offer a suitable substrate for cell attachment.
Conjugated polymers with ethylene glycol side chains provide films that are stable in
contact with water and that have the mixed ionic/electronic conductivity needed for efficient
OECT operation. However, ethylene glycol side chains, with their antifouling properties, offer a
poor substrate for cell adhesion.[2]
Here, we focus our attention to the cell-device interface. We develop polymer blends
combining efficient OECT operation and the functionalities needed for conjugation with specific
cues for cell attachment. Isoindigo donor-acceptor copolymers with hybrid alkyl/ethylene glycol
side chains provide a path for electronic and ionic conductivity.[3] PEDOT having carboxyl side
groups [3,4] is used to couple functional groups for click-chemistry with cues for cell attachment.
The blends enabled long-term device stability, for up to 6 months in cell culture media.
Covalent attachment of an extracellular matrix protein (i.e., fibronectin) provided a suitable
platform for attachment and recording of human embryonic stem cells-derived cardiomyocytes.
Overall, the blends offer a versatile strategy to attach cues that can be used to promote and/or
modulate cell-device interaction. As active materials for OECTs, the blends provide a suitable
platform to monitor the activity of electrogenic cells in vitro.