The program is in CEST Time.
A-I1
We live in an increasingly hyper-connected environment where humans, smart devices and robots live in synergy together. Flexible, wearable sensors and systems are accelerating this trend by generating ever greater amounts of data for AI algorithms to process and understand. Exciting developments in bio-integrable[1] and neuro-integrable sensory systems[2] will further augment human abilities and aid in applications as health diagnostics, surgery and predictive analytics.
We believe a multi-disciplinary approach especially in materials design and processing is essential to achieve near or even superhuman capabilities in robotics. In the area of manipulation tasks, robots have yet to match human abilities despite progress in various sensing and actuator systems. We apply a neuromorphic approach for sensory systems as a potential pathway towards greater tactile and machine intelligence[2]. I will discuss our approach and recent progress in developing new soft materials systems and bio-mimetic approaches for robotic intelligence.
A-I2
Tae-il Kim is currently working as an associate professor in School of Chemical Engineering at Sungkyunkwan University (SKKU), Korea. He earned his BS and Ph.D degree from the SKKU and Seoul National University, respectively. He moved to the University of Illinois at Urbana-Champaign (UIUC) for postdoctoral research with Prof. John A. Rogers, working on the bio-integrated optoelectronic devices and their optogenetic applications from 2009 to 2012. His current researches are based on flexible, bioinspired and bio-integrated electronics fabricated by unconventional lithographical method and advanced materials.
Arachnids are among the most sensitive creatures on the Earth. Especially, their mechano-sensory system embedded in the crack-shaped slit organ made of stiff exoskeleton over a cuticular pad near leg joints is known to sense a tiny variation of mechanical stress, thereby, serve as an ultra-sensitive vibration sensor. In this talk, we introduce spider inspired mechanosensors having an ultrasensitivity, durability with high signal-to-noise-ratio. [1] The device fabricated on a sheet of plastic is reproducible, mechanically flexible and shape-deformable so that they can be easily mounted on human skin as skin electronic with multi-pixel arrays. The spider inspired sensory system would provide versatile novel applications utilized in ultra-high sensitivity on displacements. Moreover, it will deal with crack geometry dependency [2], enhanced durability using self-healing polymer [3], strain visualization [4], and noise-damping properties.
A-O1
Dr. Hongki Kang is currently an assistant professor in the Department of Information and Communication Engineering at DGIST (Daegu Gyeongbuk Institute of Science and Technology), South Korea. He received his MS and PhD in Electrical Engineering from the University of California, Berkeley in 2010 and 2013, respectively. Prior to joining DGIST, he spent years as a postdoctoral researcher at Columbia University and at KAIST. With his experties in novel microelectronics, nanomaterials and microfabrication processes such as printing, his current research area is in neural interfaces, flexible electronics and biosensors.
Electro-optical neural interfacing technologies have been applied to offer both high temporal and high spatial resolution neural recording and stimulation. Optical neural stimulations such as optogenetics, photothermal stimulation have been successfully applied to various brain models. For the hybrid neural interface technologies, we must build optically transparent, mechanically flexible microelectrode arrays that can measure the neural signals without significant interference by light during the optical stimulations. While wide bandgap electrodes such as indium tin oxide have been traditionally used as the transparent electrodes, the mechanical brittleness of the material limits its application to in vivoor physically demanding environments. In this work, we developed ultrathin (<10 nm) metallic electrodes as the transparent and flexible microelectrode array. We used a biocompatible polyelectrolyte multilayer as the nucleation inducing seed layer for the ultrathin electrodes for enhanced electrical conductivity (<8 Ω/sq) while achieving high optical transmittance (>77%). In addition, we found that the strong electrostatic force formed by the charged polyelectrolytes altered the electrode-electrolyte interfaces of the electrode and significantly reduced the impedance and the baseline noise of the electrodes. Lastly, with the promising capability of the transparent neural electrodes, we also confirmed that the microelectrodes can be also used as reliable transparent temperature sensors.
A-O2
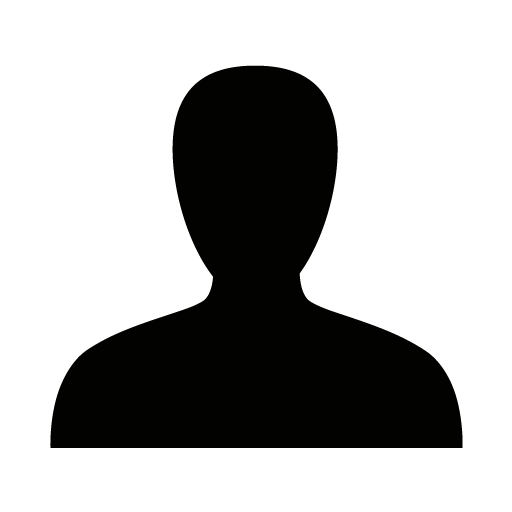
Retinal prostheses seek to create artificial vision by stimulating surviving inner retinal neurons of patients with profound vision impairment. Notwithstanding tremendous research efforts, the performance of all implants tested to date have remained rudimentary, incapable of overcoming the threshold for legal blindness. To maximize the perceptual efficacy of a retinal prostheses, a device must be capable of controlling retinal ganglion cells (RGCs), the output cells of the retina, with greater spatiotemporal precision. Most (if not all) studies of high resolution retinal stimulation were either derived from non-primate species or the peripheral primate retina, despite the uniqueness and high acuity of the central primate retina’s foveal region. In addressing this shortcoming, we are developing a more clinically relevant computational model capable of generating anatomically accurate RGC populations within the central human retina. The critical improvements of this model lie in its capacity to uphold location-dependent characteristics such as RGC density and dendritic diameter, whilst incorporating anatomically accurate features such as axon projection and three-dimensional RGC layering, features often forgone in favour of reduced computational complexity. Our model of epiretinal stimulation produced RGC excitation in the shape of wedges, halos, and elongated streaks, analogous to the perplexing and non-trivial percepts reported in clinical trials. Following this, multiple RGC populations across the central retina were stimulated to compare and assess neurostimulation configurations based on their ability to improve perceptual efficacy. Finally, these results were used to determine an upper-bound on artificial visual acuity achievable with retinal implants using today’s technologies. Our model and results could provide the means and new insights towards the development of neurostimulation protocols for next-generation, high-resolution retinal protheses.
A-O3
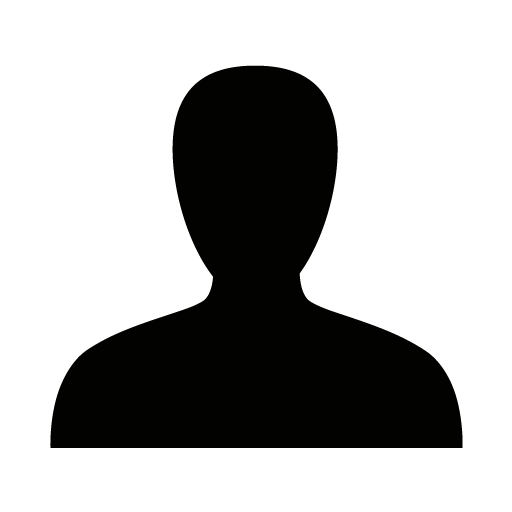
Recently developments of high data rate neural interfaces challenge the wireless communication protocol for energy efficiency, bandwidth, form factor, and scalability. Establishing an effective connection through biological tissue remains a difficult task due to its highly conductive nature. We proposed ionic communication(IC) that utilizing body ions for high-speed data communication. Here, we describe the working principles of IC and the effect of material and geometrical properties such as electrode area, material composition, ion type/concentration, and operating frequency based on the results of electrochemical impedance spectroscopy (EIS) and gain-bandwidth test. Using this approach, we were able to model the parameters and system performance including penetration depth, form factor, and power consumption. We then designed implantable transdermal IC electrodes with several MHz bandwidth. We used these devices to acquire high-quality in vivo electrophysiological data from freely moving rats. The proposed working theory of IC and the resulting devices set the foundation for advanced biomedical implantable systems, such as brain-computer interfaces, that require a low consumption, high bandwidth wireless communication system.
B-I1
Prof. Dr. Tobias Moser is a neuroscientist, otologist, and audiologist at the Göttingen Campus in Germany. He heads the Institute for Auditory Neuroscience at the University Medical Center Göttingen and leads research groups at the German Primate Center and the Max-Planck-Institutes for Biophysical Chemistry and Experimental Medicine. His main areas of research are synaptic coding and processing of auditory information as well as innovative approaches to the restoration of hearing in the deaf such as the optogenetic cochlear implant
When hearing fails, cochlear implants (CIs) provide open speech perception to most of the currently half a million CI users. The major bottleneck of current CIs is the poor coding of spectral information, which results from wide current spread from each electrode contact. As light can be more conveniently confined, optical stimulation of the auditory nerve presents a promising perspective for a fundamental advance of CIs. We have been developing cochlear gene therapy and optical cochlear implants for more than a decade and are now planning the first clinical trial to be started in few years. The presentation will provide an update on the development of optogenetic hearing restoration. Recent breakthroughs include achieving high temporal fidelity and frequency resolution, estimation of the dynamic range of optogenetic stimulation and establishing multichannel optical CIs.
B-I2
Professor Denison holds a joint appointment in Engineering Science and Clinical Neurosciences at Oxford, where he explores the fundamentals of physiologic closed-loop systems in collaboration with the MRC Brain Network Dynamics Unit. Tim also serves as an advisor to several governments and industry boards on the field of translational medical devices; in particular, helping define strategies for mapping scientific discovery to product development roadmaps within the regulatory and economic constraints of medical systems. Prior to Oxford, Tim was a Technical Fellow at Medtronic PLC and Vice President of Research & Core Technology for the Restorative Therapies Group, where he helped oversee the design of next generation neural interface and algorithm technologies for the treatment of chronic neurological disease. In 2015, he was elected to the College of Fellows for the American Institute of Medical and Biological Engineering (AIMBE). He has a PhD from MIT in electrical engineering, and an AB in Physics and MBA from the University of Chicago.
Biological rhythms permeate all living organisms at a variety of timescales. These rhythms are fundamental to physiological homeostasis, and their disruption is thought to play a key role in the progression of disease. In the last two decades, neuromodulation has been established as an effective adjunct therapy for medically refractory neurological disorders. To date, however, due to the limited sensing capabilities of neuromodulation devices exploring the influence of biological rhythms on therapy efficacy has not been feasible. However, with the development of new bioelectronic devices capable of long-term data recording and adaptive stimulation parameter adjustments, clinical neuroscience researchers are now gaining unprecedented insight into the longitudinal rhythmic behavior of patient data across a variety of neurological diseases. In this talk, we propose that future bioelectronic devices should integrate chronobiological considerations in their physiological control structure to maximize the benefits of therapy. We specifically highlight this need for deep brain stimulation (DBS) chronotherapy, where the DBS therapeutic dosage would be titrated based on the time-of-day and synchronized to each patient’s individual chronotype. This is motivated by preliminary longitudinal data recorded from both parkinsonian and epileptic patients, which show periodic symptom biomarkers synchronized to daily rhythms and other cycles. In addition, considering side effects, tonic stimulation can undermine diurnal patterns such as sleep-wake rhythms. Based on these observations, we suggest a control structure for future bioelectronic devices which incorporate anticipatory, time-based adaptation of stimulation control, locked to patient-specific biological rhythms, as an adjunct to classical feedforward and feedback methods. Initial results from two case studies using chronotherapy-enabled prototypes will illustrate the concept. The net control architecture of the bioelectronic implant mimics more closely the classical integration of adaptive, feedforward, and feedback control methods found in physiology, and could be useful as a general method for personalized therapy refinement.
B-I3
Lyes Khacef is a PostDoc in the Bio-Inspired Circuits and Systems group at University of Groningen. He received the M.Sc. in electrical engineering and embedded systems from the University of Nice Sophia Antipolis (France). He pursued a PhD at Université Côte d’Azur (France) in brain-inspired computing where he worked on understanding and modeling the local computations behind the self-organization capabilities of the brain. He proposed a new model for multimodal unsupervised learning based on local structural and synaptic plasticity rules, implemented then on FPGA-based cellular neuromorphic hardware. Dr. Lyes Khacef developed a multi-disciplinary expertise in computational neuroscience, machine learning and hardware design. He is currently working on the modeling and hardware prototyping of spike-based synaptic plasticity rules, for learning spatio-temporal patterns using neuromorphic vision, audio and tactile sensors.
Our brain-inspired computing approach attempts to simultaneously reconsider AI and von Neumann's architecture. Both are formidable tools responsible for digital and societal revolutions, but also intellectual bottlenecks linked to the ever-present desire to ensure the system is under control. The brain remains our only reference in terms of intelligence: we are still learning about its functioning, but it seems to be built on a very different paradigm in which its developmental autonomy gives it an efficiency that we haven’t yet attained in computing.
Our research focuses on the cortical plasticity that is the fundamental mechanism enabling the self-organization of the brain, which in turn leads to the emergence of consistent representations of the world. Indeed, the cerebral cortex self-organizes itself through local structural and synaptic plasticity mechanisms that are very likely at the basis of an extremely interesting characteristic of the human brain development: the multimodal association. In spite of the diversity of the sensory modalities, like sight, sound and touch, the brain arrives at the same concepts (convergence). Moreover, biological observations show that one modality can activate the internal representation of another modality when both are correlated (divergence). In this work, we propose the Reentrant Self-Organizing Map (ReSOM), a brain-inspired neural system based on the reentry theory using Self-Organizing Maps and Hebbian-like learning. We propose and compare different computational methods for unsupervised learning and inference, then quantify the gain of the ReSOM in a multimodal classification task. The divergence mechanism is used to label one modality based on the other, while the convergence mechanism is used to improve the overall accuracy of the system.
We perform our experiments on a constructed written/spoken digits database and a Dynamic Vision Sensor (DVS)/EletroMyoGraphy (EMG) hand gestures database. The proposed model is implemented on a cellular neuromorphic architecture that enables distributed computing with local connectivity. We show the gain of the so-called hardware plasticity induced by the ReSOM, where the system’s topology is not fixed by the user but learned along the system’s experience through self-organization.
B-I4
The auditory system contains a remarkable biological sensor that exhibits nanometer-scale sensitivity of mechanical detection. The first step in auditory processing is performed by hair cells, which act as transducers that convert minute mechanical vibrations into electrical signals that can be sent to the brain. The hair cells operate in a viscous environment, but can nevertheless sustain oscillations, amplify incoming signals, and even exhibit spontaneous motility. Theoretical models have proposed that a hair cell constitutes a nonlinear system, and allow us to describe and predict how they respond to incoming sound. Our experiments explore the physical mechanisms behind the detection of very weak signals, and describe them using models based on dynamical systems theory. We demonstrate the presence of chaos in the innate motility of active bundles, and show both theoretically and experimentally that it enhances the sensitivity of detection. We propose that these cells utilize weakly chaotic dynamics to combine sensitive response with high temporal resolution. Secondly, we explore the neural mechanisms that control the responsiveness of the cell. Specifically, we show that the sensitivity of the mechanical response in vitro is reduced by efferent activity, indicating that these neurons modulate the biological control parameters that fine-tune the dynamic state of the hair cell.
B-I5
The field of neuromorphic electronics is promising for local training and learning in energy restricted environments as well as in bioelectronics. Various neuromorphic devices will be presented that are based on organic-mixed conductors (ionic-electronic), materials that are traditionally used in organic bioelectronics. A prominent example of a device in bioelectronics that exploits mixed conductivity phenomena is the organic electrochemical transistor (OECT). Devices based on OECTs show volatile, non-volatile and tunable dynamics suitable for the emulation of synaptic plasticity functions. Finally, small-scale organic neuromorphic circuits allow for local learning in robotics and the realization of on-chip neuronal dynamics that are biophysically realistic.
B-I6
Right now, all of the tools that interface with our brains face a fundamental trade-off. We can either sample with low resolution, over large areas of the brain, or we can sample with fine resolution, over very small areas of the brain. This doesn’t fit with the way our brains are structured. With over 12 million neurons in each square cm of brain surface, we need to sample with high resolution over large areas in order to understand the way the brain works. The limitation is wiring. Every contact we put in the brain requires an individual wire and we can’t fit more than about 100 wires inside our heads. Using the same electronics that enable a digital camera to have millions of pixels without millions of wires, we can move some of the signal processing right to the sensors, allowing us to overcome the wiring bottleneck. The challenge is that traditional electronics are rigid and brittle. They are not compatible with the soft, curved surfaces of the brain. The solution is to make electronics that are flexible. Think of a piece of 2x4 lumber and a sheet of paper, they’re both made out of the same material, but have dramatically different physical properties. Leveraging that idea, we can make electronics that are extremely flexible, by making them very thin. Using these flexible electronics, we have developed high-density electrode arrays with thousands of electrodes that do not require thousands of external wires.
This technology has enabled extremely flexible arrays of 1,024 electrodes and soon, thousands of multiplexed and amplified sensors spaced as closely as 25 µm apart, which are connected using just a few wires. These devices yield an unprecedented level of spatial and temporal micro-electrocorticographic (µECoG) resolution for recording and stimulating distributed neural networks. I will present the development of this technology and data from in vivo recordings. I will also discuss how we are translating this technology for both research and human clinical use.
B-I7
Skin-inspired electronics is emerging as a new generation of soft electronics that may allow intimate contact with biological systems with minimal mechanical damage. Furthermore, they allow collection of sensing information with higher signal-to-noise ratio due to the lower impedance at electrode-tissue interface. In this talk, I will discuss several projects related to engineering conductive materials and developing fabrication methods to allow electronics with effective electrical interfaces with biological systems, through tuning their electrical as well as mechanical properties. The end result is a soft electrical interface that has both low interfacial impedance as well as match mechanical properties with biological tissue. Several applications of such electronics will be presented.
A-I1
Traditional neuromodulation, whether invasive or non-invasive, applies tonic stimulation in a single target to modulate brain activity in neurological and psychiatric disorders. When considering the brain as a complex adaptive Bayesian system, and applying network science approaches, symptoms and disorders are to be seen as emergent properties of (mal)adaptive network reconfigurations. This shifts neuromodulation from single target to network, i.e. connectivity-based, targeting, implementing multifocal stimulation.
But multifocal targeting also requires appropriate novel neurostimulation designs built for the purpose, either increasing connectivity in hypoconnectivity or breaking hyperconnectivity. This multifocal advanced neuromodulation approach is being developed and tested clinically using both transcranial multinetwork noise stimulation and infraslow network neurofeedback
A-I2
Large-scale neural interfacing is needed to provide better understanding of the brain at the cellular level and to develop more advanced prosthetic devices and brain-machine interfaces. Conventional neuroscience tools do not provide yet the level of density and functionality required to achieve such large-scale electrophysiology. This is because interfacing with biological tissues poses many challenges such as biocompatibility, the size scale of cellular features, the stability and longevity of the materials in a moist environment at body temperature, and the tiny amplitudes of neural signals. This talk will discuss the design and fabrication of CMOS-based neural probes, which integrate high-density micro-electrode arrays and high channel counts to enable large-scale neural recording in small animals.
A-I3
Angélica was born in Mexico City, Mexico. She received the M.S. degree in biomedical engineering from the Universidad Iberoamericana, Mexico City and the Ph.D. degree in neuroscience from the University of Geneva, Switzerland, in 1999 and 2006, respectively. She first worked as doctoral student and research assistant at the Ophthalmology Clinic of the Geneva University Hospitals. In 2011 she joined the team at the Western Switzerland University Cochlear Implants Center, and became its head of engineering in 2013. Currently, she is also leads the laboratory of Audiology of the Division of ENT and Head-and-Neck Surgery of the Geneva University Hospitals. Angélica became lecturer for the University of Geneva in 2015 and senior lecturer in 2018.
The focus of her research is sensory perception evoked by electrical neuroprostheses (retinal implants, cochlear implants, and vestibular implants). Her early scientific work was devoted to investigating the fundamental theoretical psychophysical aspects of visual prostheses and retinal implants. Later, she had the opportunity to investigate these aspects in humans, during one of the first human clinical trials of retinal prostheses. Currently, she leads the research group at the University of Geneva performing the first in-human trial of a vestibular implant to rehabilitate patients with bilateral vestibular deficits (www.vestibularimplant.org). The main focus of this research is the investigation of the effects of electrical stimulation of vestibular nerve afferents in human subjects and the clinical significance of the rehabilitation that a vestibular implant could provide to patients suffering from a bilateral vestibular loss.
Bilateral vestibulopathy is a heterogeneous disorder resulting in many disabling symptoms, including imbalance, oscillopsia, reduced mobility, and increased risk of falling. It has even been associated with cognitive impairments. Unfortunately, the prognosis is poor and currently available treatment options have very low efficacy.
Vestibular implants are implantable devices that attempt to partially restore vestibular function to patients with severe bilateral vestibulopathy of peripheral origin, using electrical currents. There have been substantial research efforts, first in animals and more recently in humans, towards the development of vestibular implants. Our group, the Geneva-Maastricht team, developed an original concept based on a modified cochlear implant. This device, developed in close collaboration with MED-EL (Innsbruck, Austria), provides 1 to 3 extra-cochlear electrodes which are implanted in the vicinity of vestibular afferents in addition to the “standard” cochlear implant array. We started implantations in humans in 2007 and, to date, 13 patients with severe bilateral vestibulopathy were implanted with these prototype devices without surgical or medical complications.
Special surgical techniques have been developed for safe implantation of these devices and their feasibility has been demonstrated in human subjects. Humans have demonstrated surprising adaptation capabilities to the artificial vestibular signal. Successful restoration of the vestibulo-ocular reflex in the mid- to high- frequency range has been demonstrated using standard clinical tests (rotatory chair and video-head impulse test). We also showed that it is possible to activate the vestibulo-collic reflex using measures of cervical myogenic vestibular evoked potentials. Controlled postural responses could also be obtained with our prototype vestibular implant device. Finally, visual abilities in dynamic settings were restored with the vestibular implant. The latter is a major step forward, providing the first ever demonstration of useful rehabilitation of this patient population.
Results obtained so far in humans are very encouraging. We hope that the increasing interest in this field and the substantial research efforts allocated lead to a clinical application in the near future. It should also be mentioned that the vestibular implant opens new possibilities for exploring several fundamental issues: balance function, the adaptive capacities of the brain, the processes of temporal integration of sensory information necessary for equilibrium, and probably for better understanding vestibular physiology and vestibular disorders. Therefore, the vestibular implant opens new perspectives, not only as an effective therapeutic tool, but also pushes us to go beyond current knowledge and well established clinical concepts.
A-O1
Currently I am working as Associate Research Professor at the 4BRAIN lab of Ghent University in Belgium. In 2002, I obtained a Masters degree in Biology at Ghent University. In 2007, I completed my PhD research at the Faculty of Medicine and Health Sciences of Ghent University. The topic of his PhD research was 'Cell Therapy for Epilepsy'. This work implicated isolation and culture of stem cells, biochemical characterization of cell clones, cell grafting in various epilepsy models, immunohistochemistry, etc... . As a postdoc, also at 4BRAIN, I studied the mechanism of action of neurostimulation therapies for epilepsy (vagus nerve stimulation and deep brain stimulation) both in animal models as in patients with epilepsy. This work involved techniques such as microdialysis and intracranial electrophysiology in rats and single-unit recording in humans. Currently my main interest is optogenetic, optopharmacological and chemogenetic modulation of epileptic brain circuits
Aim: Adenosine A1 receptors modulate neuronal activity by pre- and postsynaptic routes. The ubiquity of A1 receptors demands focal activation for specific therapeutic intervention. Here we describe how light-triggered release of the coumarin-caged A1 agonist N6-cyclopentyladenosine (cCPA) can be used to precisely control CA1 neurotransmission and excitability in acute rat hippocampal slices via a feedback control system.
Methods: Extracellular CA1 field potentials (fEPSPs) were evoked by Schaffer collateral stimulation and recorded with a 60-channel multielectrode array. First the dynamics of the response to CPA were determined using step and pulsed wash-in. Next slices were superfused with 3µM cCPA and 405 nm LED pulses (4mW) of 25/50 ms were used to release CPA. Repeated (10 sec interval) fEPSPs were recorded to monitor CA1 excitability. A light pulse was given each time its amplitude surpassed a preset level; thus constituting an ON/OFF feedback system
Results: CPA modulates fEPSPs with an ED50 of 37 nM and Hill coefficient of 3. Activation time is in the seconds range, while deactivation may take up to 10-20 minutes, reflecting the underlying G-protein signal transduction pathway. CPA effectively reduced excitability, defined as the amount of cell firing induced by synaptic input. The steep concentration dependence and asymmetric dynamics indicate a narrow and complex therapeutic range, but favors frequency modulation by short light pulses that deliver an almost all or none CPA dose. The feedback control kept the fEPSP amplitude stable for over 60 minutes in all slices (n=4) at 50% (or any other level) of its baseline value to within fEPSP variability. Doubling light puls duration reduced flash frequency but increased amplitude variation. Here is still room for refined optimization.
Conclusion: Flash-light uncaging of cCPA was used to precisely and focally control (reduce) CA1 excitability, making it a potential candidate to prevent epileptic seizures.
A-O2
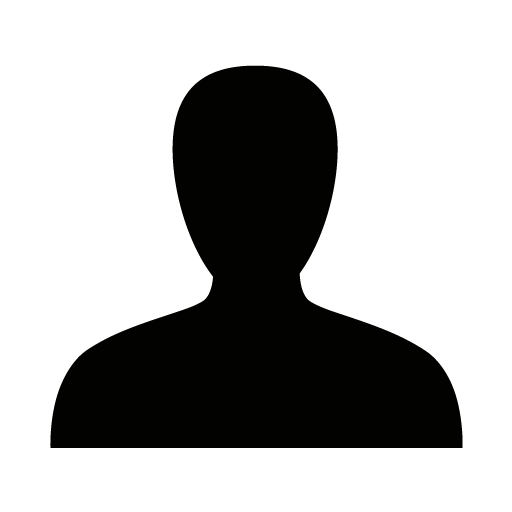
Artificial intelligence applications have demonstrated their enormous potential for complex processing over the last decade, however they still lack the efficiency and computing capacity of the brain. In living organisms, data signals are represented by sensory and motor processes that are distributed, locally merged and capable of forming dynamic sensorimotor associations through volatile and non-volatile connections. Using similar computational primitives, neuromorphic circuits offer a new way of intelligent information processing that makes it possible to adaptively oberserve, anaylze, operate and interact in real-world scenarios [1-6].
In this work we present a small-scale, locally-trained organic neuromorphic circuit for sensorimotor control and learning, on a robot navigating inside a maze. By connecting the neuromorphic circuit directly to environmental stimuli through sensor signals, the robot is able to respond adaptively to sensory cues and consequently forms a behavioral association to follow the way to the exit. The on-chip sensorimotor integration with low-voltage organic neuromorphic electronics opens the way towards stand-alone, brain-inspired circuitry in autonomous and intelligent robotics.
A-O3
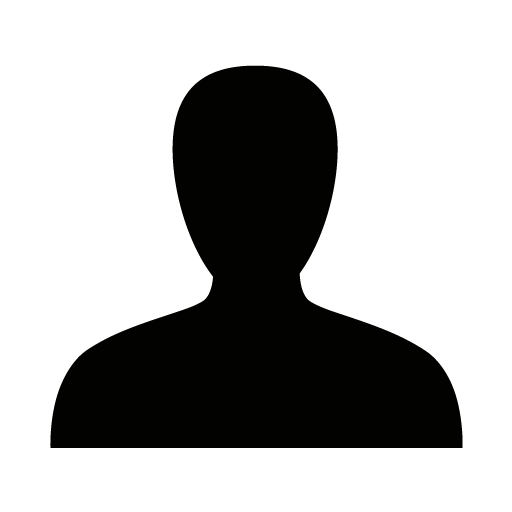
Optical techniques have greatly accelerated advances in modern medicine. Lasers and optical devices are now established in clinical practice to monitor and improve health condition of patients. Many degenerative diseases affecting the brain and the nervous system could be optically modulated at the cellular level with the right choice of light sensitive materials and devices. Organic semiconducting materials have recently emerged as promising candidates for controlling neuronal activity, both in-vitro and in-vivo. Here we show the development of light sensitive bioelectronic interfaces based on solution processed p- and n-type organic semiconductors that are used to optically stimulate neurons in vitro. These interfaces show good light absorption in a wide range of the visible spectrum (~400 nm – 700 nm) and large photo-electrochemical currents in the range of μA/cm2 when illuminated with low light intensity. Moreover, these interfaces are highly biocompatible as proved by healthy cells cultured directly on their surface as well as other cyto-toxicity assays. Primary cortical neurons extracted from rats are cultured on the surface of the devices for 21 days and form active, interconnected neuron networks. When exposed to low intensity, white light illumination, these neuron networks show a dramatic increase in activity as revealed by calcium imaging. Several individual neurons have shown action potential generation upon illumination – a sudden increase of the membrane potential as measured by patch clamp. By performing a number of photo-electrochemical measurements on the proposed semiconducting interfaces as well as other control substrates, we believe that photo-generated hydrogen peroxide plays a central role in the process of optical stimulation of the neuronal cultures. Despite that the exact mechanisms of photo-stimulation are not yet fully understood, the ability of these interfaces to operate without the need of external wires/bias is an advantage that can be leveraged to build high throughput in vitro models for neuronal network studies.
B-I1
A great demand exists for minimally-invasive neuromodulation technologies to enable next-generation bioelectronic medicine. We report on our developments of ultrathin (opto)electronic devices for neurostimulation. All of these devices rely on far red/near infrared irradiation in the tissue transparency window to actuate nanoscale organic semiconductor components. Our flagship technology is the organic electrolytic photocapacitor (OEPC) – a device that mimics biphasic current-pulse neurostimulation and thus transduces an optical signal into directly-evoked action potentials in neurons. These devices are not only wireless, but also 100-1000 times thinner than existing technologies. We will discuss chronic implants capable of stimulating peripheral nerves (sciatic and vagus) when actuated from outside of the body using diode lasers. Light power can be safely and effectively transmitted to implants up to 15 mm below the skin surface. We have observed stable operation in rodent models for at least 100 days. We believe that the combination of deep red light and ultrathin photovoltaic devices can account for a new paradigm in wireless bioelectronic medicine.
B-I2
Neural prostheses are devices integrated with the neural tissue for diagnostic or therapeutic purposes. Despite a large variety of devices exists, a shared future is the presence of cables connecting the electrode-tissue interface to implantable electronic circuits for signal management. The presence of wires and connectors is a significant disadvantage for neural prostheses often leading to failure; they exert mechanical forces and tractions on the implant and the tissue, and they are often transcranial or transcutaneous connections that might lead to post-surgical complications, such as infection. Also, the use of implantable electronic units is another disadvantage. Several aspects limit their operation, such as power consumption, excessive heat generation, and high risk of failure in a wet environment due to leakage.
In neural prostheses, wireless electrodes are desirable. Retinal prostheses are a particular category of neural prostheses, in which wireless neuronal stimulation was achieved thanks to photovoltaic technology, that also avoids the need for active implantable electronic units. In the case of retinal stimulation, photovoltaic technology is intuitive since the retina is made to absorb light entering from the pupil naturally. Although photovoltaic retinal prostheses do not work with ambient and natural light, electrical stimulation is triggered by artificial light projected into the pupil and absorbed by semiconductor elements embedded into the stimulating pixels. This solution allows retinal prostheses to avoid a transscleral flat cable which limits the maximum number of stimulating pixels on the device and induce post-operative complications such as eye inflammation or leakage through the incision.
In the first part of this talk, a wide-field, photovoltaic, and injectable retinal prosthesis will be reported. POLYRETINA is a wide-field epiretinal prosthesis which contains more than 10k photovoltaic electrodes distributed over a large area with high pixel density. The results obtained both in-vitro with retinal explants and in-vivo with large animal models will be reported.
In the second part of the talk, a novel visual prosthesis based on intra-neural stimulation of the optic nerve will be described. OpticSELINE is an intraneural 3D electrode array capable of selectively activating the optic nerve fibres to induce artificial vision. This technology is essential to overcome the exclusion criteria of retinal implants. The results from our in-vivo preclinical trial in large animals will be presented.
In conclusion, the use of photovoltaic technology to design novel neuroprostheses will be highlighted.
B-I3
A growing mechanistic understanding of the functional organization of immune-regulating reflexes has enabled use of electrical interfaces with the peripheral nervous system for the study and treatment of inflammatory diseases, and clinical studies are ongoing. Recent observations indicate that neural reflex regulation of inflammation is not limited to pro-inflammatory activity, but also plays a role in the important resolution phase of inflammation. Ongoing work aims to detail the functional anatomy of neural regulation of excessive inflammation in a range of diseases, including inflammatory bowel disease, rheumatoid arthritis, and atherosclerosis. Together, available data indicate that stimulating specific peripheral nerves is potentially an interesting approach for treatment of inflammatory diseases.
B-I4
Kelly Owens is the director of education and outreach at the Feinstein Institutes for Medical Research. In 2017, after fifteen years of active Crohn’s disease and inflammatory arthritis, and failing almost two dozen medications, she and her husband moved to Amsterdam for six months to participate in a revolutionary clinical trial run by SetPoint Medical using vagus nerve stimulation, a part of the emerging field of bioelectronic medicine. Within two months, she was deemed in clinical remission. Nowadays, her mission is to expand access to more patients suffering from inflammatory diseases and advance research.
Patients who are interested in learning more about bioelectronic medicine should email Kelly at kowens4@northwell.edu. She can share a variety of resources to learn about the science, clinical trials so far, and can add patients to the Feinstein Institutes’ patient database to be contacted for future trials.
Intro: Electricity throughout time: The Greeks, Whitman, and Tesla
My role: Director of Education at the Feinstein Institutes for Medical Research
How/why I came into this role: Diagnosed with Crohn’s and inflammatory arthritis in 2002
Experience as a patient over 15 years
Experience in the VNS clinical trial in 2017
What life looks like today: Mark Twain quote and The Benjamin Button Effect
What I’m working on now
Basic Principles of BEM
Jack Sparrow running from the villagers: What it feels like going up against the status quo
What patients are going through with current treatment options
The hurdles we face in translating from bench to bedside, including example of Kelvin & Einstein as an example of dogma vs. progress
Conclusion: where there is great need there is great opportunity.
B-I5
Dion Khodagholy is an assistant professor in the Department of Electrical Engineering at Columbia University in New York City.
He received his Master’s degree from the University of Birmingham (UK) in Electronics and Telecommunication Engineering. This was followed by a second Master’s degree in Microelectronics at the Ecole des Mines. He attained his PhD in Microelectronics at the Department of Bioelectronics (BEL) of the Ecole des Mines (France). His postdoctoral research at New York University, Langone Medical Center was focused on large-scale cortical acquisition and analysis.
His research explores the interface of electronics and the brain in the context of both applied and discovery sciences, with the ultimate goal of new innovations in device engineering and neuroscience methods to improve diagnosis and treatment of neuropsychiatric disease.
As our understanding of the brain’s physiology and pathology progresses, increasingly sophisticated technologies are required to advance discoveries in neuroscience and develop more effective approaches to treating neuropsychiatric disease. To facilitate clinical translation of advanced materials, devices, and technologies, all components of bioelectronic devices have to be considered. Organic electronics offer a unique approach to device design, due to their mixed ionic/electronic conduction, mechanical flexibility, enhanced biocompatibility, and capability for drug delivery. We design, develop, and characterize conformable organic electronic devices based on conducting polymer-based electrodes, particulate electronic composites, high-performance transistors, conformable integrated circuits, and ion-based data communication. These devices facilitate large-scale neurophysiology experiments and have led to discovery of a novel cortical oscillation involved in memory consolidation as well as elucidated patterns of neural network maturation in the developing brain. The biocompatibility of the devices also allowed intra-operative recording from patients undergoing epilepsy and deep brain stimulation surgeries, highlighting the translational capacity of this class of neural interface devices. In parallel, we are developing the high-speed conformable implantable integrated circuits and embedded acquisition and storage systems required to make high channel count, chronic neurophysiological recording from animals and human subjects possible. This multidisciplinary approach will enable the development of new devices based on organic electronics, with broad applicability to the understanding of physiologic and pathologic network activity, control of brain-machine interfaces, and therapeutic closed-loop devices.
B-I6
John A. Rogers is the Louis Simpson and Kimberly Querrey Professor of Materials Science and Engineering, Biomedical Engineering and Medicine at Northwestern University, with affiliate appointments in Mechanical Engineering, Electrical and Computer Engineering and Chemistry, where he is also Director of the recently endowed Querrey Simpson Institute for Bioelectronics. He has published nearly 800 papers, is a co-inventor on more than 100 patents and he has co-founded several successful technology companies. His research has been recognized by many awards, including a MacArthur Fellowship (2009), the Lemelson-MIT Prize (2011), the National Security Science and Engineering Faculty Fellowship (2012), the Smithsonian Award for American Ingenuity in the Physical Sciences (2013), the MRS Medal (2018), the Benjamin Franklin Medal from the Franklin Institute (2019), the Sigma Xi Monie Ferst Award (2021) and a Guggenheim Fellowship (2021). He is a member of the National Academy of Engineering, the National Academy of Sciences, the National Academy of Medicine, the National Academy of Inventors and the American Academy of Arts and Sciences.
Advanced optoelectronic systems that can intimately integrate with the brain and the peripheral nervous system have the potential to accelerate progress in neuroscience research and to enable new approaches in patient care. Specifically, capabilities for injecting electronics, light sources, photodetectors, multiplexed sensors, programmable microfluidic networks and other components into precise locations of the deep brain and for softly laminating them onto targeted regions of the surfaces of the brain or the peripheral nerves will create unique and important opportunities in stimulation, inhibition and real-time monitoring of neural circuits. In this talk, we will describe foundational concepts in materials science and assembly processes for these types of technologies, in 1D, 2D and 3D architectures. Examples in system level demonstrations include ‘cellular-scale’, injectable optofluidic neural probes for behavioral research on animal models, 3D mesoscale networks for study of neural signal propagation in organoids, and closed-loop, wireless systems for optogenetic control of bladder function.
B-I7
Neuralink is building a fully integrated, minimally invasive brain machine interface (BMI). This talk will describe the components of this BMI (including micron-scale electrodes, integrated electronics with wireless power and data telemetry, and a robotic neurosurgical device), how they address the key engineering challenges in building a scalable BMI, and applications of this technology.
Neuralink is building a fully integrated, minimally invasive brain machine interface (BMI). This talk will describe the components of this BMI (including micron-scale electrodes, integrated electronics with wireless power and data telemetry, and a robotic neurosurgical device), how they address the key engineering challenges in building a scalable BMI, and applications of this technology.