G-K1
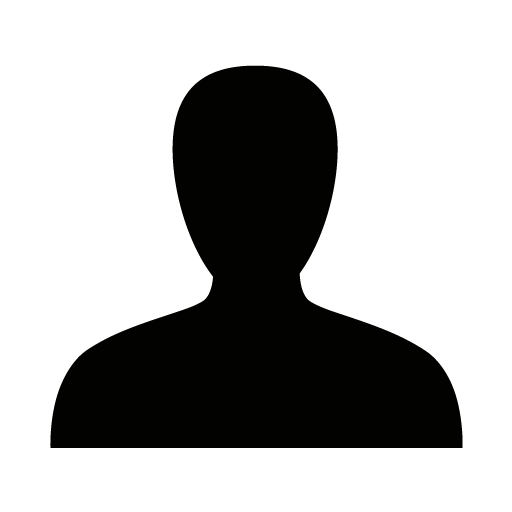
In this online seminar, we want to discuss state of the art metal halides, beyond those of the traditional lead halide perovskites This includes double perovskites (A2BCX6), vacancy ordered perovskites (like A3B2X9 and A2BX6), lower dimensional metal halides and metal chalcohalides. We will address the current challenges of making these materials, their interesting properties, like self-trapped exciton (STE) emissions, and their use in applications such as lead free metal halide based solar cells. We will also discuss how new chemistry can lead to the discovery of new metal halide structures with different properties to those that are exhibited in traditional lead halide perovskites. Finally, we will address how density-functional theory (DFT) calculations can help us to understand the luminescent properties and defects in these materials, which, in turn can provide a better passivation of defects.
G-IS1
Bio Professional Preparation M.S. in Chemistry, with Honours, University of Bari, Italy, 1996 Ph.D. in Chemistry, University of Bari, Italy, 2001 Research interests Prof. L. Manna is an expert of synthesis and assembly of colloidal nanocrystals. His research interests span the advanced synthesis, structural characterization and assembly of inorganic nanostructures for applications in energy-related areas, in photonics, electronics and biology.
Halide
G-IS2
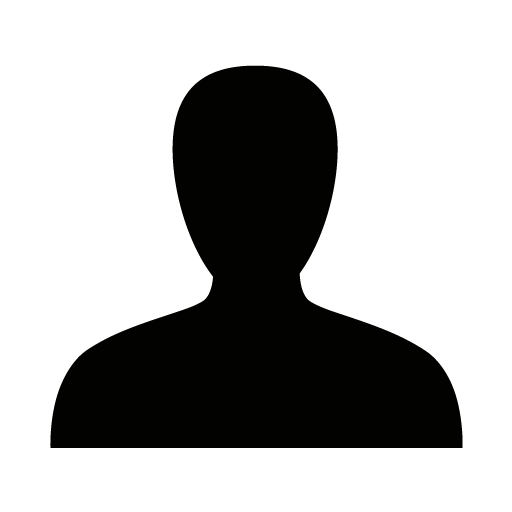
Colloidal inorganic metal halide perovskite nanocrystals (NCs) are characterized by a large surface-to-volume ratio that renders them extremely sensible to surface processes. Passivating ligands, employed to stabilize NCs in an organic solvent, play thus an important role in influencing their structure and optoelectronic properties.
A leap forward in solving the above issues is to analyze the surface using first principle simulations, such as Density Functional Theory (DFT). Until now some of the major drawbacks of this approach have been: (i) the size of the system that can be handled that in the best cases is restrained to a few hundredths atoms (i.e. a small sized NC surrounded by short ligands), and (ii) the description of static properties with the absence of dynamic effects.
Here, I show the first multiscale modeling of real sized CsPbBr3 NCs (about 9.0 nm) passivated with oleate ligands and immersed in hexane with a simulation box containing a million of atoms. Molecular dynamics simulations, carried out up to the nanoscale timescale, provide crucial insights on the surface dynamics, and the role of the ligands on the structural optoelectronic properties of these materials.
G-IS3
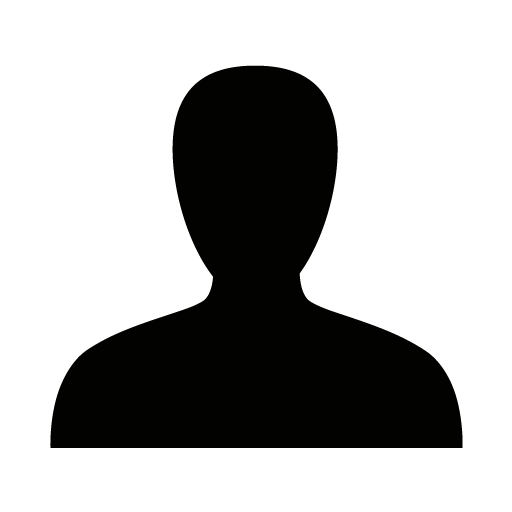
Halide perovskites, AMX3 (A+ = Cs, CH3NH3, HC(NH2)2; M2+ = Ge, Sn, Pb and X- = Cl, Br, I), is an emerging class of high-performance semiconductors which operate in the visible and infrared energy range (1.1-3.0 eV). The remarkable physical properties of the halide perovskites stem from their unique electronic structure, which originates from the characteristic arrangement of the ions in the perovskite structure-type. The electronic structure of the halide perovskites is responsible for the observed high absorption coefficients and charge-carrier mobilities, which are comparable to the venerable III-V semiconductors.
A major challenge in halide perovskites relates to the limited perovskite structure formability due to the strict limitations imposed by the tolerance factor (t) which necessitates the halide perovskites to form only when Cs, CH3NH3 or HC(NH2)2 cations are used as templating A+ cations. These restrictions significantly impact the synthetic toolbox for designing new materials with desired target properties. Thus, exploring new synthetic directions that can modify the crystal structure within the perovskite structure limits presents itself as a great challenge in the field. Using small organic cations that can potentially stabilize the perovskite structure, a wide variety of perovskite-related structures can be obtained. Interesting compounds such as perovskite polytypes or unusual metal halide frameworks (so-called “perovskitoids”) and unconventional dimensionally reduced perovskites. The thus obtained compounds are amenable to property tuning governed by specific structural features as these emerge from the three-dimensional connectivity of the octahedral [MX3]- building blocks. Understanding and controlling these structural features in a designed manner will open the way for the discovery of novel perovskite-based semiconductors which may serve as potent substitutes for the currently employed halide perovskite materials.
G-IS4
Prashant V. Kamat is a Professor of Chemistry & Biochemistry, Senior Scientist at Radiation Laboratory, and Concurrent Professor of Department of Chemical and Biomolecular Engineering, University of Notre Dame. He earned his doctoral degree (1979) in Physical Chemistry from the Bombay University, and postdoctoral research at Boston University (1979-1981) and University of Texas at Austin (1981-1983). He joined Notre Dame in 1983 and initiated the project on utilizing semiconductor nanostructures for light energy conversion. His major research interests are in three areas : (1) catalytic reactions using semiconductor and metal nanoparticles, nanostructures and nanocomposites, (2) develop advanced materials such as inorganic-organic hybrid assemblies for energy conversion, and (3) environmental remediation using advanced oxidation processes and chemical sensors. He is currently serving as a Deputy Editor of Journal of Physical Chemistry Letters and A/B/C and a member of the advisory board of scientific journals, Langmuir, Research on Chemical Intermediates, Electrochemistry and Solid State Letters, and Interface. He has written more than 400 peer-reviewed journal papers, review articles and book chapters with more than 40000 citations and carries an h-index of 109. He has edited two books in the area of nanoscale materials. He was a fellow of Japan Society for Promotion of Science during 1997 and 2003 and was awarded Honda-Fujishima Lectureship award by the Japanese Photochemical Society in 2006 and Langmuir Lectureship Award in 2012. He is a Fellow of the Electrochemical Society, American Chemical Society and AAAS.
Lead-free double perovskites such as Cs2AgBiBr6 are gaining attention because of their environmental friendliness compared to the lead halide perovskites. The Cs2BiAgBr6 QDs exhibit rich excited state dynamics and when adsorbed onto semiconductor oxides they induce ultrafast charge injection process. Whereas the electron transport layer such as TiO2 or ZnO facilitate transport of electrons to the collecting surface, it can also indirectly induce surface oxidation of QDs if holes are allowed to accumulate. Under steady state photolysis (ambient conditions) the electrons injected into TiO2 are scavenged by atmospheric oxygen, leaving behind holes which accumulate within the quantum dots (QDs). These accumulated holes further induce oxidation of QDs, resulting in the overall photodegradation of perovskite film. In order to establish their photoactivity, we have probed the excited state behavior of Cs2AgBiBr6 nanocrystals and charge injection from their excited state into different metal oxides (TiO2, ZnO). The electron transfer rate constants determined from ultrafast transient absorption spectroscopy were in the range of 1.2–5.2 × 1010 s-1. Annealed films of Cs2AgBiBr6 nanocrystals, when employed as an active layer in solar cell, delivered photocurrent under visible light excitation. Although Cs2BiAgBr6 is attractive as lead free perovskite materials, the photovoltaic performance remains rather poor. New strategies are needed to implement these double perovskites in solar cells in a more effective way