The Online Meetup will start at 12:00h UTC / 14:00 CEST, click here to check your local time.
S1-I1
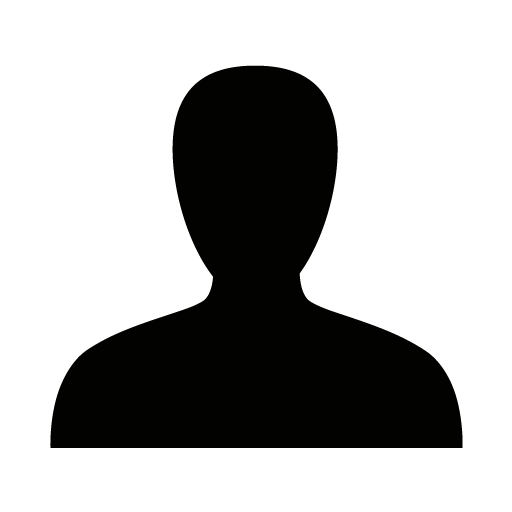
Metal halide perovskites have emerged as promising materials for optoelectronic applications. Here, we control the cation alloying to enhance optoelectronic performance through alteration of the charge carrier dynamics in mixed-halide perovskites. In contrast to single-halide perovskites, we find high luminescence yields for photoexcited carrier densities far below solar illumination conditions. We employ time-resolved spectroscopy and show that the charge carrier recombination regime changes from second to effectively first order within the first tens of nanoseconds after excitation. Supported by microscale mapping of the optical bandgap, electrically gated transport measurements and first-principles calculations, we demonstrate that spatially varying energetic disorder in the electronic states causes local charge accumulation, creating p- and n-type photodoped regions, which unearths a strategy for efficient light emission at low charge-injection in solar cells and light-emitting diodes.
S1-I2
Metal halide perovskite quantum wells (QWs) have been used to fabricate efficient optoelectronic devices, and exhibit stability superior to that of their bulk 3D counterparts. The perovskite QWs are tuned in synthesis so that they possess different bandgaps and exciton binding energies owing to variable quantum confinement as a function of QW thickness. Accordingly, the device performance of these materials depends on the efficiency of various interwell carrier dynamical processes, principally exciton and charge transfer. We probe interwell exciton transfer on timescales of 100s of femtoseconds, and show that interwell charge transfer occurs on timescales of 10s to 100s of picoseconds. These results, in addition to photoelectron spectroscopy experiments, are used to reconcile conflicting observations of type-I and type-II band alignment amongst perovskite QWs.
S1-I3
One of the advantages of halide perovskites over other high-performance semiconductors is their compositional flexibility. For instance, mixing different halides enables to obtain any desired absorption onset or emission energy in the visible. A major drawback of these mixed-halide perovskites is that their bandgap is unstable under illumination or in an electric field, since the halides segregate into domains enriched in a single halide. As a result, the bandgap is no longer homogeneous and light emission mainly occurs from the lowest-bandgap domains. In this talk, I will present how compression of mixed-halide perovskites increases the stability against halide segregation. Using pressure-dependent transient absorption spectroscopy, we found that the segregation in MAPb(I1-xBrx)3 thin films is substantially retarded at high external pressure. Identical behavior was observed in chemically compressed MAPb(I1-xBrx)3, in which compression is achieved by (partially) replacing the MA by the smaller Cs. In addition, we found that the composition of the segregated iodide- and bromide-rich phases is closer to the initial composition in compressed perovskites. I will conclude this presentation with a perspective on the long-term stability of mixed-halide perovskites, which would be essential for any application of perovskites requiring stable tunable emission energies.
S1-I4
In order to get insights on the device level the whole fabrication pre-run has to be constant and optimized. Using zwitterions as ligands for the synthesis of lead halide perovskite nanocrystals enables the reproducible, formation, isolation and complete purification of these nanocrystals in large scale (1 g) with high yields (80%). These colloids have high quantum yields (>80%), narrow emission linewidths and can be stored for months without deterioration of their optical or colloidal properties [1]. In our recent investigation we have rationalized the influence of the ligand tails on the nanocrystals colloidal stability using established models from polymer physics [2]. In the fully optimized system using natural soy-lecithin as ligands, film thicknesses can be varied between 1 µm and interparticle distances of 10 µm by varying the nanocrystal concentration of the ink used by 8 orders of magnitude.
S1-I5
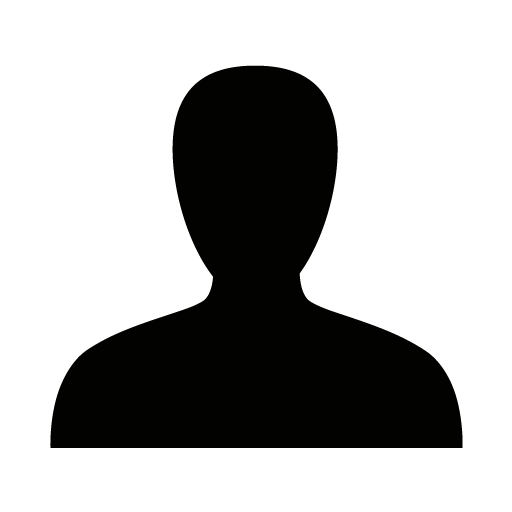
In this talk, I will introduce a new powerful technique with which we can evaluate the performance of perovskite based LEDs at the nanoscale by hyperspectral wide-field imaging. I will show how to obtain maps at the diffraction limit scale for their External Quantum Efficiency, luminance and luminous efficacy. Our observations allow us to identify the degradation paths of these emerging class of LEDs, revealing microscale heterogeneities that limit their ultimate performance. These results open avenues to diagnose the optoelectronic quality of novel semiconductors at the microscale and identify strategies to integrate them in LEDs with spatially maximised performance.
S1-I6
Halide perovskites, especially layered quasi-2D perovskites, offer a number of advantages to creating bright and efficient light-emitting applications. Their combination of excellent charge carrier mobility and low density of recombination centers have enabled their rapid ascent in electroluminescent devices. To bring perovskite LEDs to commercialization, a remaining issue of stability needs to be addressed. In this presentation, we pinpointed the chief cause of the dramatic degradation of halide perovskites in light-emitting diodes (LEDs). We studied photogenerated charges accumulating at exposed perovskite facets activate the physisorbed oxygen, converting it into reactive superoxide that triggers perovskite degradation.
We thus aimed at developing a strategy to protect the perovskite facets. At an applied level, we achieve perovskite films that exhibit a near-perfect passivation, attested to by their photoluminescence quantum yields (PLQYs) that closely approach 100%. These films are stable under continuous illumination in ambient conditions over hundred hours. In addition, we report orders of magnitude improvement in device operating stability relative to the best-performing prior perovskite reports.
S1-I7
Dr. Mahesh Gangishetty is currently a postdoctoral fellow at Rowland Institute at Harvard University. He earned his Ph.D. in Chemistry from the University of Saskatchewan, Canada and M.Sc. in Chemistry from the Indian Institute of Technology in Roorkee. His research is focused on employing variety of optoelectronic materials for energy conversion applications.
Lead halide perovskites have emerged as leading optoelectronic materials in the recent years, owing to their exceptional light emissive properties, they are showing great opportunities for low cost, flexible and high color pure next generation LEDs. Green and red LEDs reached max EQEs over 20%, the blue LEDs, however, are still struggling with EQEs around 10%. In this talk, I am going to discuss the challenges in blue perovskite LEDs and talk about our strategies of doping with metal ion at B site to improve the efficiency of LEDs. We observed a significant increase in the emission quantum yields by doping with Mn2+in blue perovskites, and the devices made by these nanocrystals showed remarkable enhancements, about 30-fold, in their EQEs.1 Further, our studies revealed that the Mn doping benefits are not limited to blue nanocrystals, they showed substantial enhancements in brightness, EQEs and stability of quasi 2D/3D including green and red LEDs.2 Most importantly the sky-blue LEDs took about 20 min until 50% of initial luminance and survived for more than an h without any spectral degradation. Finally, we observed a wide range of dopants that enhances the optical properties of all geometries of perovskites including bulk crystals.
S1-I8
Non-radiative recombination often sets an upper limit to the performance of light emitting and light harvesting devices. To overcome this hurdle, it is important to understand both the unwanted recombination mechanisms and the nature of the underlying defects. Metal halide perovskites offer the unique possibility to study the properties of individual defects beyond ensemble averaging because of their photoluminescence (PL) blinking.[1] The blinking phenomenon occurs due to the switching of individual metastable defects between a passive state and an active state, where they quench a large fraction of the luminescence. We used MAPbI3 nanocrystals as a prototypical system and studied the intensity fluctuations by means of PL microscopy with a temporal resolution of 100 ms, allowing us to analyze the blinking of large numbers of crystals simultaneously. Temperature dependent measurements revealed that the switching process is thermally activated with activation energies on the order of several 100 meV, suggesting that the switching process might be driven by ion migration.[2] Moreover, blinking at low temperatures (100 K) is correlated with fluctuations of the emission spectra, which we attribute to the heterogeneous nature of the material and to the fact that active defects are weakly emissive by themselves with signatures peaking around 800 nm. We use this observation as a starting point to estimate the non-radiative yield of individual defects and find that most of the decay mediated by these recombination centers is non-radiative, even at low temperatures.
S1-I9
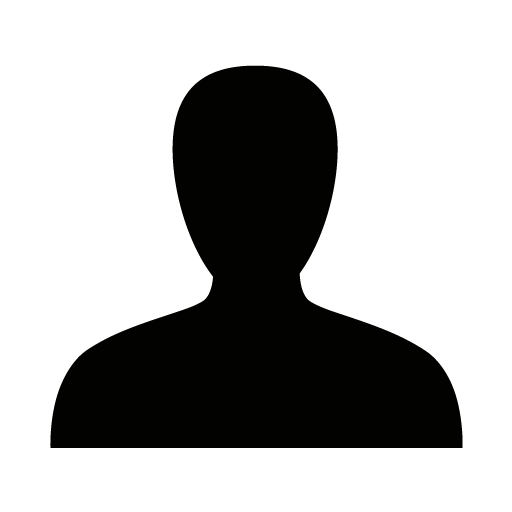
The emission of cesium lead halide (CsPbX3, where X = I,Br,Cl) perovskite-type nanocrystals is tunable over a wide energy range with ultrahigh photoluminescence quantum yields of up to 90%[1] and exhibits narrow emission line widths.
Experiments on single perovskite quantum dots at cryogenic temperature reveal an highly emissive bright triplet exciton state with photon emission rates ~1000 times higher compared to other conventional semiconductor nanocrystals[2]. Using time-tagged time-resolved single photon counting measurements we show that the ultrafast photoluminescence decay is a radiative decay from an exciton state. Furtheremore, by means of polarization dependent high-resolution spectroscopy, the complex nature of the exciton fine structure splitting and charged exciton emission has been investigated.
Based on these measurements, supported by effective-mass models and group theory calculations, we conclude that the energetically lowest triplet state is surprisingly bright opposite to many other materials and that it is responisble for the extraordinary photon emission properties of lead halide perovskites. The high oscillator strength of the bright triplet state is key for observing phenomena based on strong light—matter interactions. Moreover, these findings can assist to identify other semiconductors that exhibit bright triplet excitons, with potential implications for improved optoelectronic devices[3].
References:
[1] Protesescu et al., Nano Lett. 15, 3692–3696 (2015)
[2] Becker et al., Nature 553, 187-193 (2018)
[3] Raino et al., Nature 563, 671-675 (2018)
S1-I10
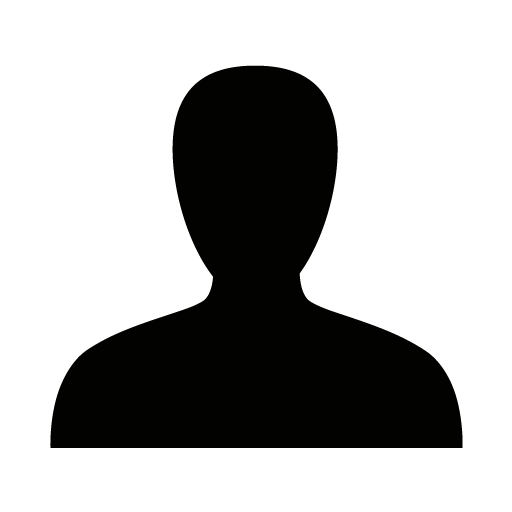
Chemically prepared colloidal semiconductor quantum dots have long been proposed as scalable and color-tunable single emitters in quantum optics, but they have typically suffered from prohibitively incoherent emission. Using advanced photon-correlation spectroscopy, I demonstrate that individual colloidal lead halide perovskite quantum dots (PQDs) – unlike any other colloidal quantum dot material - display highly efficient single-photon emission with optical coherence times as long as 80 ps, an appreciable fraction of their 210ps radiative lifetimes [1]. These measurements suggest that PQDs should be explored as building blocks in sources of indistinguishable single photons and entangled photon pairs. I demonstrate chemical tunability of the single-emitter photo-physics of PQDs as an avenue to rationally design perovskite-based quantum emitters that will benefit from the straightforward hybrid-integration with nano-photonic components that has been demonstrated for colloidal materials. [2]
[1] Utzat et al. Science, 2019, 363 (6431), pp. 1068-1072.
[2] Utzat et al. Nano Lett., 2017, 17 (11), pp 6838–6846.
S1-I11
Diluted magnetic semiconductors (DMS) based on II-VI compounds are among the most prominent materials for future spintronic applications, paving the way to magnetic functionality of nanoscaled semiconductors. Although a great deal of research has focused on the study of the photophysics of an ensemble of DMS nanostructures [1-3], recently parallel efforts have sought to unravel fundamental effects of single magnetically doped nanocrystals. In this work we investigate the formation of an excitonic magnetic polaron (EMP), i.e. the spontaneous magnetization of the dopants triggered by an exciton, via photoluminescence studies on single DMS nanocrystals [4]. While the emission linewidth is drastically enhanced compared to undoped analogues due to the interplay between EMP formation and thermodynamic fluctuations of the dopants’ spin ensemble, magnetic field dependent experiments reveal a clear signature of anisotropy effects of these collective spin phenomena.
S1-I12
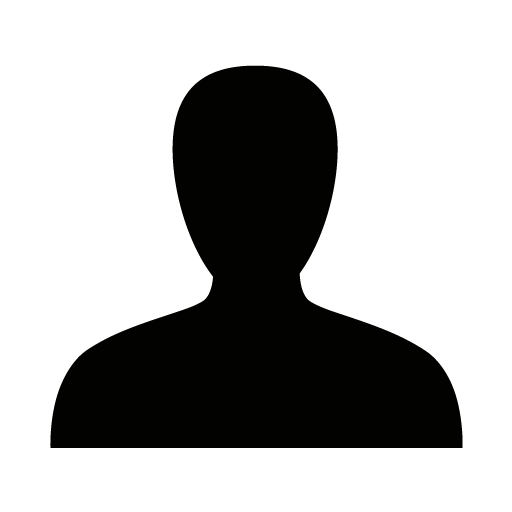
Dawei Di is a MIT Technology Review 'Innovator Under 35' (global, 2019) and 'Innovator Under 35, China' (2018). He has joined the College of Optical Science and Engineering, Zhejiang University as a Principal Investigator. He is currently a visiting researcher at the Cavendish Laboratory, University of Cambridge, UK. Dawei Di obtained a PhD (in Engineering) from the University of New South Wales, Australia and a second PhD (in Physics) from the University of Cambridge, UK. His doctoral supervisors include renowned scientists in optoelectronics and semiconductor physics, Professor Sir Richard H. Friend (FRS, FREng, FIEE, FInstP, Kt) (Cavendish Professor of Physics), and Scientia Professor Martin A. Green (FRS, AM, FIEEE, FAA, FTSE). Dawei Di’s research interests span from the exciton spin dynamics in organic light-emitting molecules, to the physics of record-breaking organic and perovskite optoelectronic devices (LEDs and solar cells). He published more than 40 papers in leading scientific journals including Science, Nature Photonics (cover article), Joule, Nature Communications, Advanced Materials, Nano Letters, ACS Energy Letters, Journal of Physical Chemistry Letters and Progress in Photovoltaics. These include 8 papers in Science/Nature/Cell family journals. He filed 4 international patents, wrote 1 book chapter, and translated 3 textbooks. His work has been featured in research news and highlights in high-profile journals such as Nature, Nature Materials and Nature Reviews Chemistry.
Excitons play a central role in the operation of high-efficiency organic LEDs. In a series of recent works, we demonstrate that intra- and inter-molecular exciton interactions in some novel molecular systems, including those involving carbene-metal-amides, are particularly efficient, leading to near-unity exciton utilization efficiencies at ultrafast (sub-picosecond) timescales. Using a combination of transient spectroscopy and device characterization, we show that these processes are key to the realization of high-performance solution-processed OLEDs. Similar ultrafast excitation transfer processes are found to be responsible for the operation of record-breaking perovskite LEDs, suggesting some similarities and differences in the operation of OLEDs and PeLEDs.
S1-I13
Alexander Romanov holds a PhD in synthetic inorganic and physical chemistry from Nesmeyanov Institute Russian Academy of Sciences. After several postdoctoral positions in the US and UK, he applied his experience in synthetic chemistry towards the discovery of the Carbene Metal Amide (CMA) materials for highly energy-efficient OLEDs. In 2019, he started his independent research career as a Royal Society University Research Fellow at the University of East Anglia. His work is focused on the molecular design towards multifunctional materials for various optoelectronic applications.
Thermally activated delayed fluorescence (TADF) has emerged as a competitive approach to provide a route towards highly energy-efficient OLED lighting and display applications. This motivated us to develop novel materials – carbene metal amides (CMA materials) and light-emitting concept to accelerate the implementation of OLED technology [1], [2], [3]. CMA materials are characterized by very small energy gap between singlet and triplet excited states leading to the delayed fluorescence with sub-microsecond excited state lifetime. Our results show that CMA is an effective platform to control fundamental photophysical properties such as luminescence colour range, excited state lifetime and photoluminescence quantum yields. Here we present our materials design strategy with a new family of materials to demonstrate that electronic characteristics which are particularly important to achieve white emitting and deep-blue CMA materials [4], [5]. As a proof of concept, we fabricated vacuum-deposited deep-blue OLED devices (CIE coordinates 0.17, 0.17) based on gold complexes with high external quantum efficiencies (>19% EQE) and slow roll-off values up to 2% at practical brightness levels of 1000 nits. Comparison and key differences of the various CMA materials will be discussed to showcase the role of the metal and organic ligands, steric and electronic factors or result of their interplay.
S1-I14
Glow-in-the-dark materials also known as Long-persistent luminescence (LPL) work by slowly releasing energy absorbed from ambient light. Used in watches and emergency signs, most commercial glow-in-the-dark materials are based on inorganic compounds and include rare metals. However, these materials are expensive and require high temperatures to manufacture. Carbon-based organic materials can overcome many of these disadvantages.
The long-lived emission from organic molecules is known as phosphorescence, which is a transition between different spin states. However, the phosphorescence does not achieve long-lasting emission over one hour like the inorganic systems because of the emission mechanism. The LPL is an emission mechanism in which the energy passes through an intermediate state like a trapped state. There is no restriction regarding spin state. While LPL is long-lived because of charge separation and subsequent slow recombination (second-order kinetics) of initially generated excitons, phosphorescence is long-lived because of the low probability of the transition (first-order kinetics) occurring in the initially generated excitons.
We realized first organic LPL systems by using intermediate charge-separated (CS) states. By mixing an electron-donating molecule and an electron-accepting molecule, emission lasting for over an hour was demonstrated. we also reported that the energy gap between the lowest localized triplet excited-state and the lowest singlet charge-transfer excited-state in the exciplex system significantly controls the LPL performance. Moreover, greenish-blue to red and even warm white emission are achieved by energy transfer from exciplex in the organic LPL (OLPL) matrix to the emitter dopants. The dopants also improve brightness and emission duration through efficient radiative decay and the trapping of electrons, respectively. We also demonstrated a polymer-based organic LPL system that is flexible, transparent, and solution-processable.
S1-I15
π-Extended organic architectures are a fascinating class of compounds that plays a fundamental role in the materials science. The efficient overlap of atomic orbitals along an extended net of polyaromatic systems enables outstanding properties such as strong luminescence and high charge mobilities. An efficient strategy to modulate the latter optoelectronic properties consists in embedding heteroatoms into the carbon scaffold. To that end, phosphorus atoms are particular interesting. They present an electron lone pair that is readily available for a variety of reversible post-functionalization reactions with transition metals, Lewis acids, oxidizing and alkylating agents,1 which may serve to modulate the structural and electronic properties of the systems they are embedded in. Thus, incorporating phosphorus centers into p-systems prompts unique electronic characteristics that are inaccessible from any other heteroatom.2,3
In this communication, I will provide an overview of our research on π-extended six-membered phosphorus heterocycles (6M-PHs). In particular, I will describe the properties and applications of the phosphaphenalenes4-6 and introduce the chemistry of a new generation of π-extended architectures based on 6M-PHs, the diphosphahexaarenes.7 All in all, I will describe the benefits of embedding 6M-PHs into π-extended polyaromatic hydrocarbons.