This program is in UTC / London time. Click here to check your local time.
- Moscow time: UTC+3
- Paris - Berlin: UTC+1
- New York time: UTC-5
Download pdf
1-I1
A light harvesting system that efficiently drives photocatalytic component is essential for converting solar light into chemical energy. Recently, we constructed biohybrid light-harvesting (LH) systems composed of LH complexes from photosynthetic purple bacteria to which artificial dyes are covalently attached as external LH pigments [1-3]. The LH activity of light-harvesting 2 (LH2) complexes was successfully enhanced through attachment of fluorophores, e.g., Alexas and ATTO. Energy transfer from the fluorophores to bacteriochlorophylls, B800 and B850, was observed in the time domain of sub-pico to dozen of picoseconds. We have also demonstrated that the biohybrid LH2 complexes efficiently drive a photocatalytic component, light-harvesting 1–reaction center core complex (LH1-RC), which exhibited enhanced charge separation and photocurrent generation activity when assembled into lipid bilayer [3,4]. Contribution of LH to the photocatalytic reactions in LH1-RC was quantitatively evaluated. More recently, we constructed biohybrid-type LH1-RCs, to which the fluorophores directly attached to LH1. Enhanced activities of LH and photocurrent generation were observed [5].
1-T1
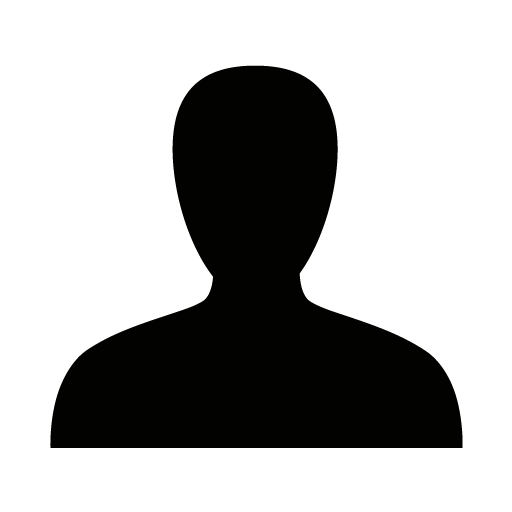
The light-harvesting two complex (LH2) of the purple photosynthetic bacterium Rhodobacter (Rba.) sphaeroides is a highly efficient, light-harvesting antenna that allows growth under a wide-range of light intensities. In order to expand the spectral range of this antenna complex, we first used a series of competition assays to measure the capacity of the non-native pigments 3-acetyl chlorophyll (Chl) a, Chl d, Chl f or bacteriochlorophyll (BChl) b to replace native BChl a in the B800 binding site of LH2. We then adjusted the B800 site and systematically assessed the binding of non-native pigments. We find that Arg-10 of the LH2 β polypeptide plays a crucial role in binding specificity, by providing a hydrogen-bond to the 3-acetyl group of native and non-native pigments. Reconstituted LH2 complexes harbouring the series of (B)Chls were examined by transient absorption and steady-state fluorescence spectroscopies. Although slowed 10-fold to ~6 ps, energy transfer from to Chl a to B850 BChls remained highly efficient. We measured faster energy-transfer rates for Chl d (3.5 ps-1) and Chl f (2.7 ps-1), which have red-shifted absorption maxima compared to Chl a. BChl b, red-shifted from the native BChl a, gave extremely rapid (≤0.1 ps-1) transfer. These results show that modified LH2 complexes, combined with engineered (bacterio)chlorophyll biosynthesis pathways in vivo, have potential for retaining high efficiency while acquiring increased spectral range.
1-T2
Bio-hybrid nanomaterials have great potential for combining the most desirable aspects of biomolecules with the conceptual aspects of nanotechnology, both to potentially develop new solar energy harvesting technology and also to understand fundamental properties of natural systems. The plant antenna protein Light-Harvesting Complex II (LHCII) is extremely efficient at solar energy absorption and subsequent energy transfer in nature and has been demonstrated to perform effectively when incorporated into artificial nanoscale assemblies. The combination of chlorophyll and carotenoid pigments within LHCII provide relatively good absorption coverage across the visible spectrum, except for a ‘green gap’ of minimal absorption between 520-620nm. Here, we demonstrate that this spectral gap can be efficiently filled in a membrane-based assembly of LHCII together with non-covalently incorporated synthetic chromophores. Texas Red, an organic chromophore, was selected as the energy donor as it can be crosslinked onto the headgroup of a lipid, stabilising it within a model lipid membrane system. These “proteoliposomes” (protein-lipid vesicles) act as energy-transferring nanomaterials which offer the modularity to assemble a range of concentrations of the LHCII proteins and the complementary chromophores. A comprehensive analysis of the system on both the ensemble-level and the single-proteoliposome-level revealed highly efficient energy transfer both in solution and when deposited onto surfaces. This was achieved by utilising a combination of steady-state and time-resolved fluorescence spectroscopy alongside fluorescence lifetime imaging microscopy (FLIM). Lipid nanodiscs allow the investigation of energy transfer from lipid-tagged chromophores to membrane reconstituted LHCII, on a single protein level. Very recent ultrafast spectroscopy investigations have revealed the picosecond timescale of this energy transfer, and give insight into the nanoscale interactions between chromophore-linked lipids and light-harvesting membrane proteins. This system provides a platform for controllable distance-dependant energy transfer from synthetic chromophores to light-harvesting proteins, and demonstrates the potential of self-assembly driven bio-hybrid enhancement of natural systems.
1-I2
Julea Butt is Professor of Biophysical Chemistry at the University of East Anglia, Norwich, UK. She graduated from Oxford University in 1989 with a degree in Chemistry, pursued research for her PhD at the University of California, Irvine, USA and post-doctoral research at the National Institutes of Health, USA and Wageningen University, Netherlands. In 1997 she joined the University of East Anglia as a Wellcome Trust Career Development Fellow and was subsequently appointed as a lecturer (2001), reader (2004) and professor (2010). Throughout her career Julea has pursued multi-disciplinary studies for molecular level understanding of the functional properties of electron transfer proteins, both the fundamentals and the opportunities for solar conversion. She collaborates with research teams across the UK and internationally and in 2015 was awarded a Royal Society Senior Research Fellowship.
Coupling synthetic photosensitizers with multiheme cytochromes offers exciting opportunities. Heme-heme electron transfer can be quantified by pump-probe spectroscopy [1,2]. The ability to accumulate photoenergised electrons may allow the coupling of single-electron photochemistry and multi-electron chemical transformations for light-driven chemical synthesis [3,4]. Inspired by these prospects we have been studying an extracellular multiheme cytochrome from electrogenic Shewanella bacteria. This cytochrome is spanned by a chain of 10 hemes and forms an essential part of the molecular wire conducting electrons across the outer membrane of Shewanella [5]. This contribution presents results from our recent studies of biohybrid assemblies in which the extracellular cytochrome is site-selectively labelled with a Ru(II)(2,2’-bipyridine)3 photosensitiser. Fresh insights into electron transfer along the heme chain have been afforded by pump-probe spectroscopy. Implications for using the assemblies for semi-artificial photosynthesis are considered.
1-T3
Sustainable energy production is critical for meeting growing worldwide energy demands in a carbon-neutral manner. One area of research interest to meet this is the development of dye-sensitized solar cells (DSSCs). The operative components of a DSSC consist of: a photosensitive dye adsorbed onto a TiO2 semiconductor, a counter electrode, and a redox mediator. Photosystem I (PSI) is a naturally occurring photoelectrochemical reaction center which converts photons to chemical potential energy during photosynthesis and has shown the ability to act as the photosensitizer in biohybrid DSSCs. The functionality and efficiency of the device are dependent on a number of factors, including the band-gap of the dye, conduction band of the semiconductor, and the midpoint potential of the mediator. Although the I-/I3- redox mediator couple is the most commonly used in DSSCs, it is corrosive to proteins, has a midpoint potential similar to PSI, and absorbs light in the visible spectrum making it unfavorable for a biohybrid devices. Herein, we report on the development of biologically compatible bi-pyridine based cobalt redox mediator complexes for use in PSI-based photovoltaic devices. These Co (II/III) complexes are not corrosive and offer more negative midpoint potentials, resulting in greater driving forces for the regeneration of PSI available for photoexcitation. A series of complexes bearing electron donating functional groups have been prepared and their compatibility tested with PSI. We investigated a number of organic/aqueous solvent conditions to satisfy both mediator and PSI solubility requirements for electrochemical activity. Electron transfer assays have shown that these cobalt complexes are capable of directly reducing photooxidized PSI+. We also report on the effects of a mixed organic solvent-aqueous phase on PSI+ reduction kinetics, slowing direct reduction rates approximately 8-38 depending on the donor, with implication for dye regeneration rates in biohybrid photovoltaic devices based on electrolyte choice. Currently, the synthesis of more water-soluble cobalt complexes bearing polyethyleneglycol (PEG) groups is underway. Both bipyridine and phenanthroline based cobalt complexes with PEG groups will be tested for their electron mediator capabilities with PSI. (Supported by grants from the CSUB Start-up funds, NSF, NIH and Army Research Laboratory.)
1-I3
I am currently an Full Professor at the University of Bonn (Germany), Department of Chemistry. My overarching motivation is to discover and implement the chemistry necessary to transition to a sustainable energy-based society. Specifically, I am developing materials to convert electrical energy to fuels and chemicals.
The synergestic integration of synthetic and biological components can result in functional systems with capacities far beyond their individual components. Within the context of energy research, the use of microbes as catalysts for CO2 conversion is intended to access selectivity not yet attainable with sythetic catalysts (e.g. nanoparticles). To acellerate the progress of these biohybrid systems, a detailed knowledge of how charge is transferred between the inorganic electrode/light absorber and the microbe is key. To this end, this talk will discuss the translation of several analytical techniques (e.g. spectroscopy, QCM...) to the investigation of two separate biohybrid systems: 1) A CdS photocatalyst integrated with moorela thermoacetica as a photocatalytic CO2 conversion platform and 2) metal-oxide electrodes onto which geobacter sulfurreducens biofilms are grown. The work paves the way for further studies of analogous systems and the elucidation of charge transfer pathways [uts forth rational improvisation of biohybrid energy conversion systems.
1-T4
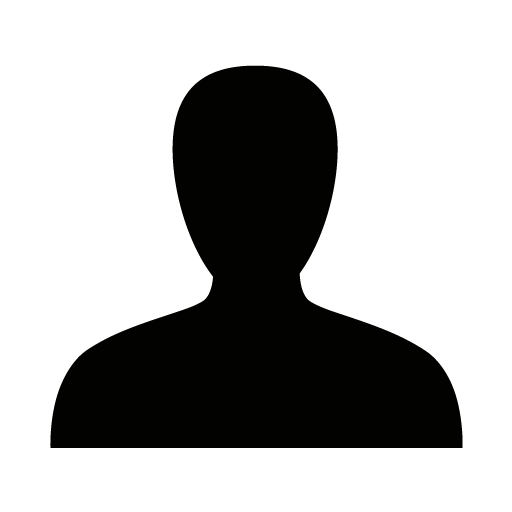
With the aim to harvest solar energy as green energy, photosynthetic protein complexes are attractive building blocks for the fabrication of semi-artificial energy conversion devices. Photosystem 1 (PS1), one of the key enzymes that drive photosynthesis, has been increasingly investigated for this aim due to its strong reductive force upon light-induced charge separation processes. In our work, isolated PS1 was integrated into an Os-complex modified redox polymer, which serves both as immobilization matrix and as efficient electron transfer mediator, resulting in a photocathode with high photocurrent generation, while methyl viologen served as the charge carrier for the collection of electrons exiting PS1 [1]. The fabricated photocathode was further investigated by scanning photoelectrochemical microscopy (SPECM), providing a better understanding of electron transfer processes and revealing possible short circuiting processes involved in photocurrent generation, when a conventional electron scavenger such as methyl viologen was used [2]. The detailed knowledge acquired enabled a further optimized design and fabrication of PS1-based photocathodes, leading to an improved efficiency by minimizing short circuiting processes [3]. Moreover, light-induced H2 evolution was realized when a hydrogenase was integrated within the fabricated PS1 based photocathode. Further coupling with a photosystem 2-based photoanode, made possible the implementation of a fully light-driven Z-scheme type water-splitting cell, without requiring any external bias voltage. In this photovoltaic cell, full biophotoelectrochemical water splitting is relying exclusively on light and the photosynthetic protein complexes as energy and photoactive materials respectively, while providing useful electrical power [3].
1-T5
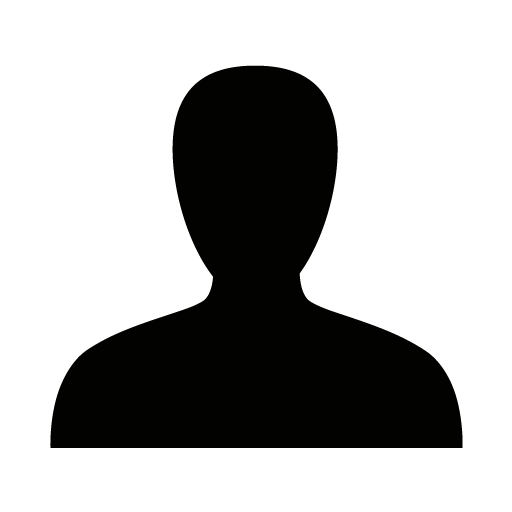
Technological developments in materials chemistry lead to progresses in electrochemistry. The possibility to tune the material properties over a wide range is especially important for biohybrid electrodes to adapt the artificial surface for the needs of the biological component.
These advances are helpful in the field of bioenergy, for example for biophotovoltaics. In these fairly new form of electrodes photo active protein complexes are connected to synthetic surfaces to convert sunlight into electric current and interesting energy-rich chemicals.[1] The goal of this work is the buildup of a biophotovoltaic based on 3D graphene and photosystem I (PSI). Here a facile, scalable approach was targeted. The construction was realized by using reduced graphene oxide (rGO), which is relative cheap and accessible in comparatively high quantities.[2] Photocurrent production was already achieved for 3D rGO when combined with photosystem II but is a novelty for PSI.[3] The 3D structure is generated by a template process based on latex beads and spin coating. The general template procedure already proved to be successful for photobioelectrodes made of other artificial electrode materials.[4] A hydrazine treatment was optimized for the reduction of the constructed 3D material.
The obtained electrode has been evaluated regarding the interaction with PSI. An assembly as well as the direct electron transfer from electrode to the reaction center is ensured resulting in a cathodic photocurrent. The redox protein cytochrome c is added to connect more PSI complexes with the 3D rGO via mediated electron transfer. The protein electrode is characterized with different electrochemical methods such as cyclic voltammetry, chopped light voltammetry and photo action spectroscopy. Additionally, analytical methods such as UV/VIS spectroscopy and scanning electron microscopy (SEM) have been applied. It is important to note that the thickness of the electrode material is adjustable in the manufacturing process. Even by preparing multiple layers the final electrode remains semi-transparent, which is advantageous as it is therefore well suited as biophotovoltaic device. Even more important it was found that the photocurrent can be enhanced with the use of thicker electrode structures.
2A-I1
Dr. Caroline Ajo-Franklin is Professor in the Department of BioSciences at Rice University. Her scientific training started in Chemistry; she earned a B.S. in Chemistry at Emory University in 1997 and received her Ph.D. in Chemistry from Stanford University in 2004. She then trained as postdoctoral fellow in Synthetic Biology with Pam Silver at Harvard Medical School, and moved to Lawrence Berkeley Lab in 2007 to start her independent research career, and then in 2019, she moved to Rice University as a Professor with appointments in BioSciences, Bioengineering and the Systems, Synthetic, and Physical Biology Program. During her career, has built a strongly interdisciplinary research program focused on molecular-level understanding and engineering of the interface between living organisms and non-living materials.
Microorganisms precisely control biological processes, including biosynthesis and cell growth, by regulating the redox state of different biomolecules. These processes can be modulated by electrochemically coupling intracellular biomolecules to an external electrode, but current approaches afford only limited control and specificity. Here we describe using electrical current to reduce specific biomolecules, allowing control of biosynthesis and cell growth in two industrially relevant microorganisms, Escherichia coli and Lactobacillus plantarum.
To enable electrochemical control of E. coli, we used synthetic biology to introduce a heterologous electron transfer pathway. E. coli expressing mtrCAB from Shewanella oneidensis MR-1 consumed electrons directly from a cathode when fumarate or nitrate, both intracellular electron acceptors, were present. The fumarate-triggered current consumption occurred only when fumarate reductase was present, indicating all the electrons passed through this enzyme. Moreover, MtrCAB-expressing E. coli used current to stoichiometrically produce ammonia. Thus, our work introduces a modular genetic tool to reduce a specific intracellular redox molecule with an electrode, opening the possibility of electronically controlling biological processes such as biosynthesis and growth in any microorganism.
Complementing this approach, we discovered that L. plantarum, a lactic acid bacteria used industrially to produce fermented foods, can uptake electrons. This electron uptake occurs under anaerobic conditions when a terminal electron acceptor and its corresponding oxidioreductase are present. Unlike other anaerobic respiratory modes in L. plantarum, this current consumption does not require addition of co-factors such as heme or riboflavin. Electron uptake promotes cell growth and acidification of the media, both processes essential for food fermentation. We find that the L. plantarum metabolism is shifted towards ATP producing pathways in the presence of both a cathode and a suitable electron acceptor. This surprising discovery opens the possibility of using electrical current to drive industrial production of fermented foods using lactic acid bacteria.
2A-T1
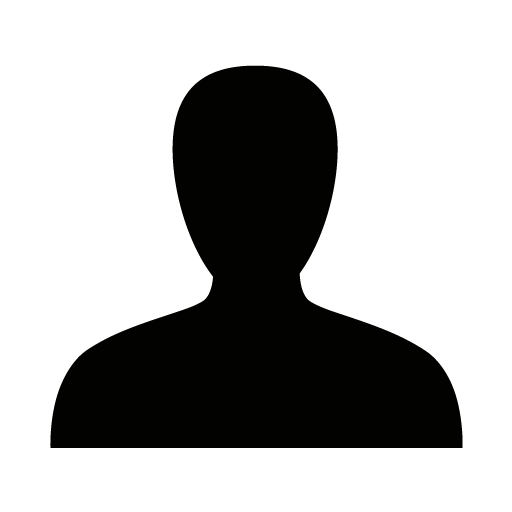
Compartmentalization is a ubiquitous building principle in cells, which permits segregation of biological elements and reactions. The carboxysome is a specialized bacterial organelle that encapsulates enzymes into a virus-like protein shell and plays essential roles in photosynthetic carbon fixation[1-4].The naturally designed architecture, semi-permeability, and catalytic improvement of carboxysomes have inspired rational design and engineering of new nanomaterials to incorporate desired enzymes into the protein shell for enhanced catalytic performance[5-6]. Here, we build large, intact carboxysome shells (over 90 nm in diameter) in the industrial microorganism Escherichia coli by expressing a set of carboxysome protein-encoding genes. We develop strategies for enzyme activation, shell self-assembly, and cargo encapsulation to construct a robust nanoreactor that incorporates catalytically active [FeFe]-hydrogenases and functional partners within the empty shell for the production of hydrogen. We show that shell encapsulation and the internal microenvironment of the new catalyst facilitate hydrogen production of the encapsulated oxygen-sensitive hydrogenases(Li et al, nature communications, accepted). The study provides insights into the assembly and formation of carboxysomes and paves the way for engineering carboxysome shell-based nanoreactors to recruit specific enzymes for diverse catalytic reactions.
2A-T2
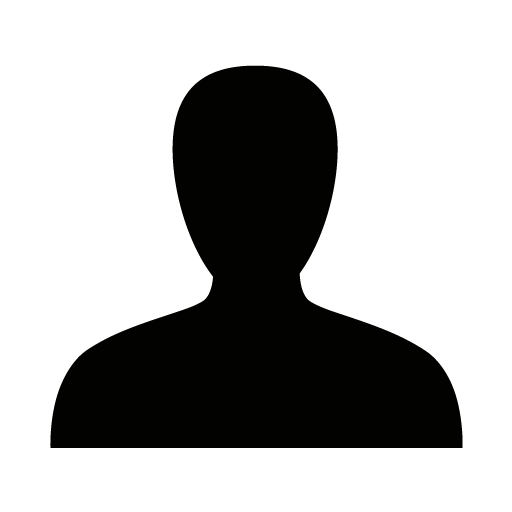
Certain photosynthetic micro-organisms exhibit the ability to export electrons/reducing equivalents upon illumination in a phenomenon called exoelectrogenic activity. There is great potential to harness exoelectrogenesis of photosynthetic micro-organisms in biophotovoltaic systems to renewably generate electricity, but power outputs remain low[1]. Rational optimisation of biophotovoltaic systems is being held back by present limited understanding of the biological basis of exoelectrogenesis of photosynthetic micro-organisms.
We know the most about exoelectrogenesis in the cyanobacterium Synechocystis sp. PCC 6803 (hereafter Synechocystis), which is a model organism for the study of photosynthesis due to the endosymbiotic origin of the chloroplast[2]. The electrons eventually exported upon illumination are known to originate from water by photosystem II action at the start of the photosynthetic electron transfer chain in the thylakoid membrane, and are thought to exit the downstream of photosystem I[3,4]. It is not known how the electrons/reducing equivalents transverse the many boundary layers of the cell from the thylakoid membranes to outside the cell.
A three-electrode set-up can be used to study the photo-electrochemistry of photosynthetic micro-organisms and their isolated photosynthetic machineries[5,6]. In chronoamperometric experiments, the net current is recorded at an electrode as it evolves from the photosynthetic material over time under chopped light (i.e. light/dark cycles). A previous study compared the photoelectrochemistry of whole Synechocystis cells and isolated photosystem II from another cyanobacterium, both using state-of-the-art hierarchically structured inverse opal indium-tin oxide (IO-ITO) working electrodes[7]. These exhibited markedly different photocurrent profiles: whole cells gave a complex photocurrent profile, whereas isolated photosystem II gave a monophasic photocurrent profile.
Presented here is a follow up study of the photoelectrochemistry of intermediate sub-cellular fractions of Synechocystis to identify which topological features of the cyanobacterial cell are responsible for the complexity in the photocurrent profile of whole cells. We found that the periplasmic space, significantly contributes to the complex photocurrent profile of Synechocystis cells, not the surface layer or type IV pili, enhancing our understanding of the exoelectrogenic routes in this model photosynthetic micro-organism.
2B-I1
I am Professor of Microbiology at Queen Mary, University of London, UK. My research interests are on the cell biology of cyanobacteria, including photosynthesis, membrane biogenesis, light perception and signal transduction, motility and multicellularity.
The unicellular cyanobacterium Synechocystis sp PCC 6803 is widely used as a model organism for photosynthesis research. It has roughly spherical cells about 2.5-3 microns in diameter. The photosynthetic light reactions take place in complexes embedded in the thylakoid membranes, which in Synechocystis form multiple, slightly irregular, layers surrounding the central cytoplasm. Whilst investigating the mechanism of directional light perception for phototaxis [1], we stumbled on an unexpected property of Synechocystis cells: they act as very effective microlenses, even when the cells are immersed in water. Under unidirectional illumination, each cell focuses a sharp spot of light close to the opposite edge of the cell. I will show experimental measurements and theoretical simulations that demonstrate that the lensing effect results in drastically different light intensities in different regions of the thylakoid membranes. This effect must have strong implications for photosynthesis, and it could be crucial for efficient solar energy conversion at low light intensities.
2B-T1
I am an Associate Professor at University of Leeds and my group's research interests include: (i) organization and photophysics of Light-Harvesting protein complexes, (ii) biohybrid LH complexes including synthetic pigments, (iii) model systems of lipid membranes and LH complexes to understand photosynthesis.
It is challenging to study the nanoscale biophysics the proteins within intact thylakoid membranes because they are complicated, high-density, multi-layer systems. Here, we present “bio-hybrid membranes” which are generated by combining natural thylakoids and synthetic lipids and assembling them into a micro-patterned template of polymerized lipids. This platform has several useful properties including: a native-like mixture of membrane proteins, a facile self-assembly process, and a robust template which promotes the formation of supported lipid bilayers from such high-protein-density natural membranes. These hybrid membranes allowed us to assess how the energy transfer pathways and protein arrangement are correlated. Fluorescence Lifetime Imaging Microscopy revealed that the fluorescence lifetime of the light-harvesting (LH) proteins within hybrid membranes is significantly longer than native membranes and this, combined with Atomic Force Microscopy data, suggested that protein-protein interactions are reduced. The migration of LH proteins from natural membranes into the putative assembling hybrid membranes was observed in real time with fluorescence microscopy. Finally, hybrid membranes were compared to proteoliposomes as a basis to assess the potential electron activity of Photosystem II, however, these experiments revealed that common photochemical assays used in the photosynthesis community actually produce false-positive results for LH antenna complex proteins. A pre-print of this work is available here [1].
2B-T2
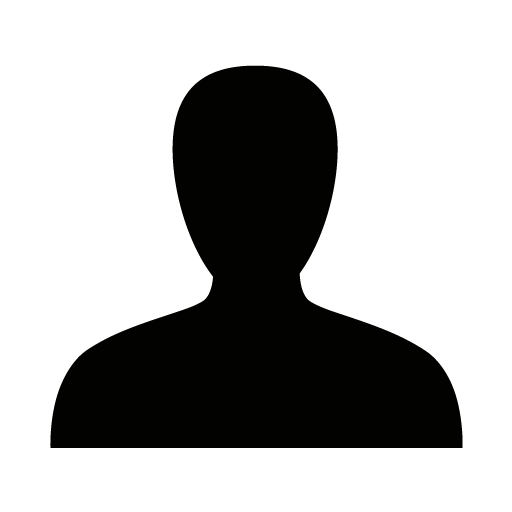
Surface-enhanced infrared absorption (SEIRA) spectroscopy is a powerful technique to understand interfacial events including protein orientation/structure changes and enzymatic reactions at protein/electrode interfaces, which are well known to govern redox properties of redox-active proteins in protein film electrochemistry and redox-based biosensors. Particularly for enzymatic reactions involving infrared-active substrates, SEIRA spectroscopy of enzyme-modified electrodes under potential control allows us to observe reaction intermediates, leading us to gain the mechanistic insight into enzymatic reactions [1]. For example, IR-active nitric oxide (NO) can be efficiently converted to nitrous oxide (N2O) at the binuclear reaction center consisting of a heme and a nonheme iron in a transmembrane metalloenzyme of cytochrome-c-dependent nitric oxide reductase (cNOR). Since NO is highly cytotoxic and reactive with biomolecules, understanding the enzymatic NO reduction mechanism, which remains under debate, would provide insights into the regulation dynamics of cytotoxic NO in biological processes.
Herein, we report electrochemical SEIRA spectra of cNOR-modified gold electrodes under catalytic conditions. The cNOR was immobilized on SEIRA-active gold electrodes via mixed self-assembled monolayers (SAMs) of alkanethiols and then protein-tethered bilayer lipid membranes (BLMs) were constructed at the electrode surface. We will discuss the protein orientation, the BLM assembly kinetics and the reaction intermediate of the enzymatic NO reduction.
2B-I2
She obtained a PhD degree in Azerbaijan. She has spent two years in the Institute of Plant Biology, Szeged, Hungary as UNESCO and ITC fellow. She has joined the Molecular Plant Biology unit at the University of Turku as a postdoctoral research fellow in 2002. Currently she is acting as a PI of the 'Photosynthetic microbes' team. Since 2017 she is Associated Professor of Molecular Plant Biology. She mainly focuses on cyanobacterial / algal research and the alternative electron-transport routes, which are heavily involved in regulation of photosynthesis via maintaining redox homeostasis in cells. She is a co-chair of the Nordic Center of Excellence “Towards Versatility of Aquatic Production Platforms: Unlocking the Value of Nordic Bioresources” (NordAqua, www.nordaqua.fi) funded by NordForsk (2017-2022) and a chair of the Biocity Turku Research Programme “Advanced Bioresources and Smart Bioproducts – Towards Sustainable Bioeconomy” (SmartBio, www.smartbio.fi).
Photosynthetic aquatic microorganisms, cyanobacteria and algae, are considered as third or fourth generation truly sustainable feedstock for blue biorefineries. Proof-of-concept trials for dozens of genetically-engineered photosynthetic organisms hosting novel synthetic pathways for production of desired chemicals are currently available. However, most of the available systems demonstrate low solar-to-product conversion efficiencies and need significant improvements to serve as industrial-scale production platforms. We apply two different strategies to improve photosynthetic production systems: modulation of alternative electron transport pathways in order to eliminate 'waste' points in photosynthesis [1-3] and development of efficient solid-state (cyanobacterial or algal artificial thin films) production system [4-6]. The engineered photosynthetic microbes entrapped in the tailored polymeric matrix could truly act as photo-biocatalyst for the prolonged bioproduction by strongly limiting biomass accumulation and maintaining photosynthetic activity and cell fitness.
2B-T3
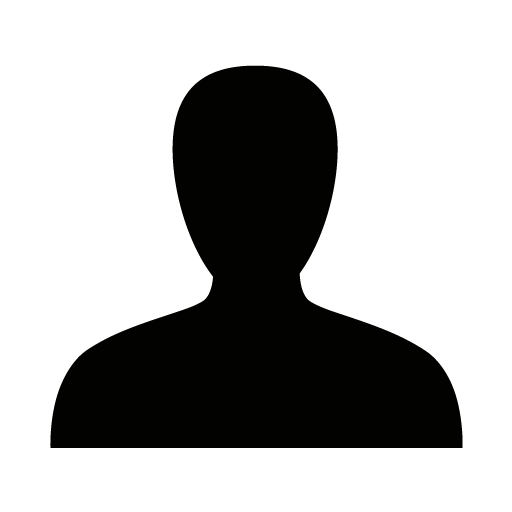
Biogenesis of photosystem II (PSII), nature’s water splitting catalyst, is assisted by auxiliary proteins that form transient complexes with PSII components to facilitate stepwise assembly events. Using cryo-electron microscopy, we solved the structure of such a PSII assembly intermediate with 2.94 Å resolution. It contains three assembly factors (Psb27, Psb28, Psb34) and provides detailed insights into their molecular function. Binding of Psb28 induces large conformational changes at the PSII acceptor side, which distort the binding pocket of the mobile quinone (QB) and replace bicarbonate with glutamate as a ligand of the non-heme iron, a structural motif found in reaction centers of non-oxygenic photosynthetic bacteria. These results reveal novel mechanisms that protect PSII from damage during biogenesis until water splitting is activated. Our structure further demonstrates how the PSII active site is prepared for the incorporation of the Mn4CaO5 cluster, which performs the unique water splitting reaction. [1]
3A-I1
The 3-D structures of light-harvesting (LH) and reaction centre (RC) complexes have revealed the molecular arrangements of pigments that foster efficient solar energy harvesting and charge separation. Atomic force microscopy (AFM) allows us to understand the next level of structural information, namely the supramolecular organization of individual complexes to form a ‘photosynthetic unit’. Recent computational models of whole membrane assemblies, comprising hundreds of protein complexes and thousands of pigments, can predict energy transfer and trapping behaviour and identify desirable design motifs for artificial photosynthetic systems.
Given our ability to purify, characterize, alter, and model the behavior of light-harvesting and reaction centre complexes, we are now in a position to fabricate new 2-D molecular assemblies, forming networks for energy trapping that provide test-beds for controlling and corralling energy migration.
This talk will briefly summarise the ‘design rules’ that apply to native photosystems, and will then outline a number of lithographic approaches for surface fabrication of nanoscale arrays of native and artificial antenna proteins. Methods for measuring the in situ energy and electron transfer properties of immobilised complexes will be presented, and I will outline some future directions for assembling the functional parts of an integrated artificial photosynthetic system.
3A-T1
Many strategies for meeting mankind’s future energy demands through the exploitation of
plentiful solar energy have been influenced by the efficient and sustainable processes of
natural photosynthesis. A limitation affecting solar energy conversion based on
photosynthetic proteins is the selective spectral coverage that is the consequence of their
particular natural pigmentation. Here we demonstrate the bottom-up formation of
semi-synthetic, polychromatic photosystems in mixtures of the chlorophyll-based LHCII
major light harvesting complex from the oxygenic green plant Arabidopsis thaliana, the
bacteriochlorophyll-based photochemical reaction centre (RC) from the anoxygenic
purple bacterium Rhodobacter sphaeroides and synthetic quantum dots (QDs) [1].
Polyhistidine tag adaptation of LHCII and RC enabled predictable self-assembly of
LHCII/RC/QD nanoconjugates, the thermodynamics of which could be accurately
modelled and parameterised. The tri-component biohybrid photosystems displayed
enhanced solar energy conversion via either direct chlorophyll-to-bacteriochlorophyll
energy transfer or an indirect pathway enabled by the QD, with an overall energy transfer
efficiency comparable to that seen in natural photosystems.
3A-T2
Photosystem I from the thermophilic cyanobacterium Thermosynechococcus elongatus is the largest membrane protein complex to have had its structure solved by X-ray diffraction. This trimeric complex has 51 transmembrane domains, 33 distinct subunits, over 330 non-covalently bound cofactors and a molecular weight of ~1.2 MDa. We have succeeded in isolating this complex without the use of detergents, using styrene maleic acid (SMA) alternating copolymer. The resulting SMA lipid particle (SMALP) containing trimeric PSI remains the largest protein complex to be isolated by this method. A host of biochemical, biophysical and functional assays have been applied to characterize this non-detergent form of PSI. Once formed, PSI-SMALP from T. elongatus have demonstrated a 3 nm red-shift in chlorophyll fluorescence, suggesting a more native orientation of the chlorophyll antennae within the PSI, as well as faster reduction kinetics following a photooxidation event. The latter observation may be related to an ultra-fast charge separation event that has been observed within PSI-SMALP by transient absorption femtosecond spectroscopy. This study shows a ~1,000-fold increase in the charge separation event of PSI-SMALP (~80 fs) over detergent solubilized complex (36 ps). Using the powerful tools of contrast variation and matching available only to SANS, we have performed a detailed dimensional analysis of the ~1.47 MDa PSI-SMALP, breaking it into its individual components of protein core, lipid annulus and copolymer or detergent micelle layers (for PSI-DDM). These trimeric PSI nanodiscs show ~50-fold increase in native lipids retained, with sulfoquinovosyldiacylglycerol being the most enriched lipid class in these PSI-SMALPs, suggesting the lipids of the thylakoid membrane are heterogeneously distributed, with alterations in the local profile within these highly uniform, PSI containing regions. These highly active and cost-effective PSI nanodiscs show great promise for scalability and integration into biohybrid solar devices for sustainable, non-resource limited electricity generation from sunlight.
3A-I2
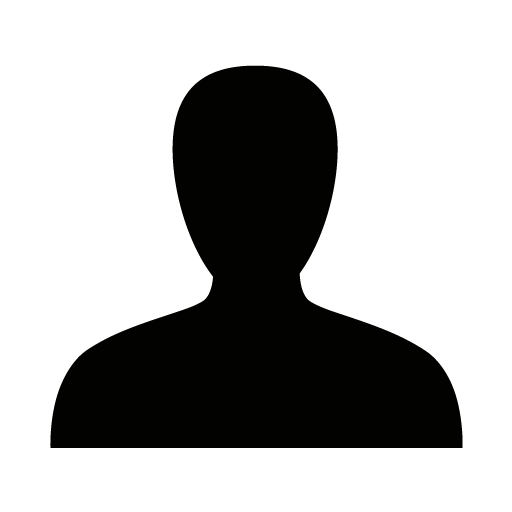
Biohybrid systems assembled from photosynthetic elements interfaced with electrode materials are envisioned as promising alternatives for light energy conversion. In analogy to the working principle of dye sensitized solar cells, the central feature of biohybrid cells is the light harvesting component needed for generation of the high-energy electrons. The main challenges for achieving efficient energy conversion reside in the wiring of the photosynthetic materials. This lecture focusses on electrochemical tools to study the electron delivery and collection from photosystems immobilized in thin films on electrode surface. Methods for quantification of the various steps of the electron transfer chain will be presented. The impact of the composition and geometry of the photoelectrocataytic film will be discussed with respect to both energy conversion efficiency and electron transfer mechanism. Importantly, charge recombination at the electrode or within the photosynthetic film, a process that decreases the energy conversion efficiency, will be delineated. Further development of electroanalytical methods and their combination with spectroscopic tools will help to gain the necessary information for rational design and optimization of the light-induced electron transfer chain in biohybrid photosynthetic systems.
3A-T3
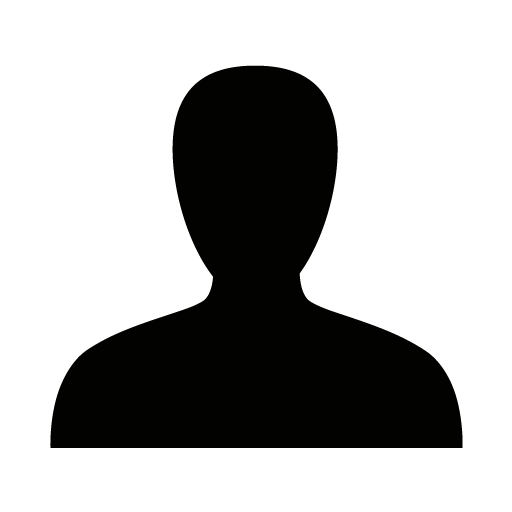
In the emerging field of microbial based electrochemical devices much effort has been put into the analysis and benchmarking of the underlying mechanisms of those systems.[1] However, two major hinderances appear in the general analysis. Firstly, electrochemistry is a technique which always measures the processes of the whole electrode-solution interface. Hence, it is not capable to distinguish between different processes simultaneously taking place but will average the local current. On the biological side, organisms have complex reaction cascades and charge transfer mechanisms making it difficult to analyse the individual steps and the bottlenecks related to them. In both fields modelling evolved as a powerful tool to overcome those hinderances.[2-4] Nonetheless, combination of electrochemical modelling and modelling of biological systems can be a challenging task. Here we present the first steps of modelling cyanobacterial photoelectrodes by a combination of a kinetic photosynthesis model embedded in an electrochemical reaction-diffusion model. With this we try to fit and analyse experimentally observed electrochemical data to reveal the underlying charge transfer mechanism. After finding a precise and reliable description of the system we can use this model to predict the associated bottlenecks of the photoactive device. As the total flux determines the performance of electrochemical devices, these findings can be applied to genetic and device engineering to overcome the currently low power output. Additionally, the general structure how we face this problem cloud be applied to other biological and non-biological devices with sophisticated catalytic mechanisms.
3B-I1
Activation of rhodopsin in tissue for optogenetic applications suffers from ineffective light delivery due to limited penetration depth of visible light. At longer wavelengths scattering is reduced and near-infrared (NIR) light in biological tissues can penetrate deeper than visible light. Lanthanide-doped upconversion nanoparticles (UCNPs) convert NIR light to visible light. We synthesized UCNPs to render rhodopsin Near-InfraRed (NIR)-sensitive, and used FTIR spectroscopy to probe the light-induced conformational changes in sensory rhodopsin II (SRII). Sub-20 nm lanthanide-doped UCNPs emit blue light upon NIR excitation, which overlaps with the absorption spectrum of SRII. Engineering these UCNPs to SRII, we examine wireless activation of SRII by NIR light. The IR difference spectrum of SRII in the presence of UCNPs with NIR excitation reveals striking similarities to that of SRII triggered with blue light illumination. This study demonstrates the first molecular spectroscopic evidence for NIR activation of a microbial rhodopsin via UCNPs.
3B-T1
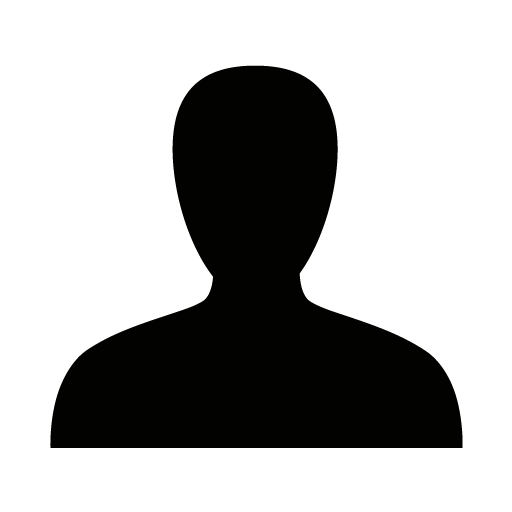
Interprotein electron transport (ET) is a key process for living organisms, playing a fundamental role in respiration and photosynthesis. ET processes between photosynthetic complexes and electron carrier partner proteins have been widely studied with bulk spectroscopic techniques [1]. However the characterization of ET at the level of single molecules with controlled protein-protein distance is lacking. This is due in part to the lack of a well-defined experimental setup for protein orientation and current measurement. In this work, a peptide [2] that binds selectively to plant Photosynthetic Complex I (PSI) is used to functionalize atomically flat gold monocrystal electrodes.
PSI binding is evaluated in bulk with photo-current and chopped light voltammetry and by scanning probe techniques with atomic force microscopy (AFM) and electrochemical scanning tunneling microscopy (ECSTM). ECSTM-based spectroscopic measurements allow investigating the current decay distance [3] (β [nm-1]) of PSI functionalized electrodes under electrochemical control. Mapping β over sample and probe potentials reveals enhanced charge exchange distance as probe potential is aligned with PSI’s electron acceptor cofactor redox potential.
3B-T2
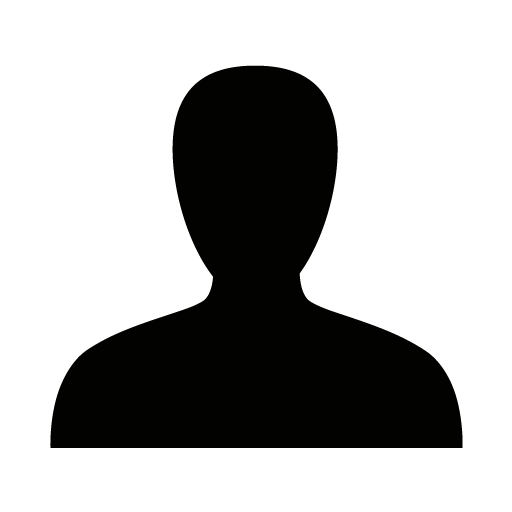
In our work, we study and compare the electron transfer (ET) reactions inside the photosystem I (PSI) cores from different photosynthetic organisms prepared in solution and immobilized on conducting glass (fluorine-doped tin oxide; FTO) forming a biophotoelectrode. The decay kinetics and the time scales of ET recombination reactions in the PSI incorporated in the photovoltaic systems define the amount of photocurrent produced/photoactivity of PSI. In the previous work performed in our group, the photovoltaic efficiency turned out to be low (below 1% of absorbed photons to generated photoelectrons) when PSI from cyanobacterium Synechocystis sp. PCC 6803 was used as a light sensitive material in a semiartificial solar cell.[1] One of the hypothetical reasons for this low efficiency can be disturbed ET inside the PSI and, if so, an accurate understanding of the decay kinetics of P700+ (often caused by charge recombination reactions) is necessary. The ET in the cyanobacterial PSI suspended in a buffer solution was studied by transient absorption spectroscopy in the micro- to millisecond time domain with probing light of 700-nm wavelength (ground state absorption maximum of P700). The results of the preliminary measurements of charge recombination in PSI demonstrates that the electron from excited P700 reaches the final electron acceptor only in a small fraction of proteins (a few percent; a constant component resolved in a ~50-ms time window). The remaining faster phases (dominated by a ~1-ms component) observed were assigned to the charge recombination reactions (back ET to P700+) from the intermediate ET acceptors occurring in the disturbed proteins. Studies of P700+ decay by transient absorption at 700 nm were thus used as a fingerprint to reveal whether the electron localized on any of the intermediate ET cofactors recombines with the hole on P700⁺ or is transferred forward to the final electron acceptor, FA/FB, from which it can be transferred further outside PSI, and thus may contribute to the photocurrent in a photovoltaic system.
3B-I2
Living photovoltaics represent a growing class of microbial devices that are based on whole cell–electrode interactions. The limited charge transfer at the cell–electrode interface represents a significant bottleneck in realizing an efficient technology. This presentation focuses on the development of poly(3,4‐ethylenedioxythiophene) (PEDOT)‐based electrodes that are electrosynthesized in the presence of a sodium dodecyl sulphate (SDS) dopant. Potentiodynamic and potentiostatic electrochemical techniques, as well as scanning electron microscopy (SEM), atomic force microscopy (AFM), Raman spectroscopy, and theoretical modelling of the electropolymerization transient, are employed to create and characterize PEDOT electrodes under various conditions. The electrodes are able to capture photosynthetically derived current under multiple light–dark cycles when interfaced with Synechocystis sp. PCC 6803. In the presence of the Synechocystis, the PEDOT electrodes show a six-fold and two-fold enhancement over conventional graphite electrodes for both mediatorless and K3Fe(CN)6‐mediated conditions, respectively. The ability of these electrodes to enhance extracted photocurrent for both direct and indirect electron transfer mechanisms provides a versatile platform for improving various microbial devices.
Reference : Reggente, M.; Politi, S.; Antonucci, A.; Tamburri, E. ; Boghossian, A. A. Adv. Mater. Technol. 5, 1900931 (2020).
3B-I3
The growing field of biohybrid systems for solar energy conversion is yielding new promising directions for sustainable energy systems. A new scientific field also leads to new and interesting questions to explore. As highlighted in this conference, a key challenge in biohybrid systems is controlling the bio-material interface. The interactions between the various materials in a system will mediate photoactive processes such as light-harvesting, energy transport, and charge separation. In order to develop a scheme by which we can design materials from the bottom up, we must understand how these interactions will either drive or limit device function.
Transient absorption (TA) spectroscopy is a valuable tool for characterizing solar energy conversion materials. This technique allows us to track the dynamics of photoexcited energy transfer, charge separation, and quenching channels. It is used extensively in the field of emerging photoconversion materials, such as organic electronics. It has also played a critical role in developing the library of knowledge on photosynthetic systems. This talk will present an overview of how TA spectroscopy can be used to advance the field of biohybrid solar energy conversion systems.
Here you can see your Local Time and UTC time